INTRODUCTION
The Enterobacteriaceae are a large, heterogeneous group of gram-negative rods whose natural habitat is the intestinal tract of humans and animals. The family includes many genera (Escherichia, Shigella, Salmonella, Enterobacter, Klebsiella, Serratia, Proteus, and others). Some enteric organisms, such as Escherichia coli, are part of the normal microbiota and incidentally cause disease, but others, the salmonellae and shigellae, are regularly pathogenic for humans. The Enterobacteriaceae are facultative anaerobes or aerobes, ferment a wide range of carbohydrates, possess a complex antigenic structure, and produce a variety of toxins and other virulence factors. Enterobacteriaceae, enteric gram-negative rods, and enteric bacteria are the terms used in this chapter, but these bacteria may also be called coliforms.
CLASSIFICATION
The Enterobacteriaceae are the most common group of gram-negative rods cultured in clinical laboratories and along with staphylococci and streptococci are among the most common bacteria that cause disease. The taxonomy of the Enterobacteriaceae is complex and rapidly changing since the introduction of techniques that measure evolutionary distance, such as nucleic acid hybridization and nucleic acid sequencing. According to the National Library of Medicine’s Internet Taxonomy database (available at ), 63 genera have been defined; however, the clinically significant Enterobacteriaceae comprise 20–25 species, and other species are encountered infrequently. In this chapter, taxonomic refinements will be minimized, and the names commonly used in the medical literature are generally used. A comprehensive approach to identification of the Enterobacteriaceae is presented in Chapters 33, 37, and 38 of Jorgensen et al, 2015.
Members of the family Enterobacteriaceae have the following characteristics: They are gram-negative rods, either motile with peritrichous flagella or nonmotile; grow on peptone or meat extract media without the addition of sodium chloride or other supplements; grow well on MacConkey agar; grow aerobically and anaerobically (are facultative anaerobes); ferment rather than oxidize glucose, often with gas production; are catalase positive, oxidase negative (except for Plesiomonas) and reduce nitrate to nitrite; and have a 39–59% G + C DNA content. They can be differentiated to species level by a vast array of biochemical tests. In the United States, commercially prepared kits or automated systems are used to a large extent for this purpose. However, these are largely being replaced by other methods. The implementation of matrix-assisted laser desorption ionization time of flight mass spectroscopy (MALDI-TOF MS) for identification of culture isolates is replacing the more traditional panels of biochemicals currently in use in most clinical microbiology laboratories. This new technology seems to work quite well for identification of most of the common Enterobacteriaceae encountered in clinical material except for Shigella species. This technology is unable to differentiate Shigella from E coli.
The major groups of Enterobacteriaceae are described and discussed briefly in the following paragraphs. Specific characteristics of salmonellae, shigellae, and the other medically important enteric gram-negative rods and the diseases they cause are discussed separately later in this chapter.
The Enterobacteriaceae are short gram-negative rods (Figure 15-1A). Typical morphology is seen in growth on solid media in vitro, but morphology is highly variable in clinical specimens. Capsules are large and regular in Klebsiella species, less so in Enterobacter species, and uncommon in the other species.
E coli and most of the other enteric bacteria form circular, convex, smooth colonies with distinct edges. Enterobacter colonies are similar but somewhat more mucoid. Klebsiella colonies are large and very mucoid and tend to coalesce with prolonged incubation. The salmonellae and shigellae produce colonies similar to E coli but do not ferment lactose. Some strains of E coli produce hemolysis on blood agar.
Carbohydrate fermentation patterns and the activity of amino acid decarboxylases and other enzymes are used in biochemical differentiation. Some tests, such as the production of indole from tryptophan, are commonly used in rapid identification systems, but others, such as the Voges-Proskauer reaction (production of acetylmethylcarbinol from dextrose), are used less often. Culture on “differential” media that contain special dyes and carbohydrates (eg, eosin-methylene blue [EMB], MacConkey, or deoxycholate medium) distinguishes lactose-fermenting (colored) from non–lactose-fermenting colonies (nonpigmented) and may allow rapid presumptive identification of enteric bacteria (Table 15-1).
Lactose fermented rapidly Escherichia coli: metallic sheen on differential media; motile; flat, nonviscous colonies Enterobacter aerogenes: raised colonies, no metallic sheen; often motile; more viscous growth Enterobacter cloacae: similar to Enterobacter aerogenes Klebsiella pneumoniae: very viscous, mucoid growth; nonmotile |
Lactose fermented slowly Edwardsiella, Serratia, Citrobacter, Arizona, Providencia, Erwinia |
Lactose not fermented Shigella species: nonmotile; no gas from dextrose Salmonella species: motile; acid and usually gas from dextrose Proteus species: “swarming” on agar; urea rapidly hydrolyzed (smell of ammonia) Pseudomonas species (see Chapter 16): soluble pigments, blue-green and fluorescing; sweetish smell |
Many complex media have been devised to help in identification of the enteric bacteria. One such medium is triple sugar iron (TSI) agar, which is often used to help differentiate salmonellae and shigellae from other enteric gram-negative rods in stool cultures. The medium contains 0.1% glucose, 1% sucrose, 1% lactose, ferrous sulfate (for detection of H2S production), tissue extracts (protein growth substrate), and a pH indicator (phenol red). It is poured into a test tube to produce a slant with a deep butt and is inoculated by stabbing bacterial growth into the butt. If only glucose is fermented, the slant and the butt initially turn yellow from the small amount of acid produced; as the fermentation products are subsequently oxidized to CO2 and H2O and released from the slant and as oxidative decarboxylation of proteins continues with formation of amines, the slant turns alkaline (red). If lactose or sucrose is fermented, so much acid is produced that the slant and butt remain yellow (acid). Salmonellae and shigellae typically yield an alkaline slant and an acid butt. Although Proteus, Providencia, and Morganella species produce an alkaline slant and acid butt, they can be identified by their rapid formation of red color in Christensen’s urea medium. Organisms producing acid on the slant and acid and gas (bubbles) in the butt are other enteric bacteria.
1. Escherichia—E coli typically produces positive test results for indole, lysine decarboxylase, and mannitol fermentation and produces gas from glucose. An isolate from urine can be quickly identified as E coli by its hemolysis on blood agar, typical colonial morphology with an iridescent “sheen” on differential media such as EMB agar, and a positive spot indole test result. More than 90% of E coli isolates are positive for β-glucuronidase using the substrate 4-methylumbelliferyl-β-glucuronide (MUG). Isolates from anatomic sites other than urine, with characteristic properties (above plus negative oxidase test results) often can be confirmed as E coli with a positive MUG test result.
2. Klebsiella–Enterobacter–Serratia group—Klebsiella species exhibit mucoid growth, large polysaccharide capsules, and lack of motility, and they usually give positive test results for lysine decarboxylase and citrate. Most Enterobacter species give positive test results for motility, citrate, and ornithine decarboxylase and produce gas from glucose. Enterobacter aerogenes has small capsules. Some species of Enterobacter have been moved into the genus Cronobacter. Serratia species produces DNase, lipase, and gelatinase. Klebsiella, Enterobacter, and Serratia species usually give positive Voges-Proskauer reactions.
3. Proteus–Morganella–Providencia group—The members of this group deaminate phenylalanine, are motile, grow on potassium cyanide medium (KCN), and ferment xylose. Proteus species move very actively by means of peritrichous flagella, resulting in “swarming” on solid media unless the swarming is inhibited by chemicals, such as phenylethyl alcohol or CLED (cystine-lactose-electrolyte-deficient) medium. Whereas Proteus species and Morganella morganii are urease positive, Providencia species usually are urease negative. The Proteus–Providencia group ferments lactose very slowly or not at all.
4. Citrobacter—These bacteria typically are citrate positive and differ from the salmonellae in that they do not decarboxylate lysine. They ferment lactose very slowly if at all.
5. Shigella—Shigellae are nonmotile and usually do not ferment lactose but do ferment other carbohydrates, producing acid but not gas. They do not produce H2S. The four Shigella species are closely related to E coli. Many share common antigens with one another and with other enteric bacteria (eg, Hafnia alvei and Plesiomonas shigelloides).
6. Salmonella—Salmonellae are motile rods that characteristically ferment glucose and mannose without producing gas but do not ferment lactose or sucrose. Most salmonellae produce H2S. They are often pathogenic for humans or animals when ingested. Organisms originally described in the genus Arizona are included as subspecies in the Salmonella group.
7. Other Enterobacteriaceae—Yersinia species are discussed in Chapter 19. Other genera occasionally found in human infections include Cronobacter, Edwardsiella, Ewingella, Hafnia, Cedecea, Plesiomonas, and Kluyvera.
Enterobacteriaceae have a complex antigenic structure. They are classified by more than 150 different heat-stable somatic O (lipopolysaccharide) antigens, more than 100 heat-labile K (capsular) antigens, and more than 50 H (flagellar) antigens (Figure 15-1B). In Salmonella serotype Typhi, the capsular antigens are called Vi antigens. The antigenic classification of Enterobacteriaceae often indicates the presence of each specific antigen; for example, the antigenic formula of an E coli may be O55:K5:H21.
O antigens are the most external part of the cell wall lipopolysaccharide and consist of repeating units of polysaccharide. Some O-specific polysaccharides contain unique sugars. O antigens are resistant to heat and alcohol and usually are detected by bacterial agglutination. Antibodies to O antigens are predominantly IgM.
Although each genus of Enterobacteriaceae is associated with specific O groups, a single organism may carry several O antigens. Thus, most shigellae share one or more O antigens with E coli. E coli may cross-react with some Providencia, Klebsiella, and Salmonella species. Occasionally, O antigens may be associated with specific human diseases (eg, specific O types of E coli are found in diarrhea and in urinary tract infections).
K antigens are external to O antigens on some but not all Enterobacteriaceae. Some are polysaccharides, including the K antigens of E coli; others are proteins. K antigens may interfere with agglutination by O antisera, and they may be associated with virulence (eg, E coli strains producing K1 antigen are prominent in neonatal meningitis, and K antigens of E coli cause attachment of the bacteria to epithelial cells before gastrointestinal or urinary tract invasion).
Klebsiellae form large capsules consisting of polysaccharides (K antigens) covering the somatic (O or H) antigens and can be identified by capsular swelling tests with specific antisera. Human infections of the respiratory tract are caused particularly by capsular types 1 and 2 and those of the urinary tract by types 8, 9, 10, and 24.
H antigens are located on flagella and are denatured or removed by heat or alcohol. They are preserved by treating motile bacterial variants with formalin. Such H antigens agglutinate with anti-H antibodies, mainly IgG. The determinants in H antigens are a function of the amino acid sequence in flagellar protein (flagellin). Within a single serotype, flagellar antigens may be present in either or both of two forms, called phase 1 (conventionally designated by lowercase letters) and phase 2 (conventionally designated by Arabic numerals), as shown in 15-3. The organism tends to change from one phase to the other; this is called phase variation. H antigens on the bacterial surface may interfere with agglutination by anti-O antibody.
There are many examples of overlapping antigenic structures between Enterobacteriaceae and other bacteria. Most Enterobacteriaceae share the O14 antigen of E coli. The type 2 capsular polysaccharide of Klebsiella is very similar to the polysaccharide of type 2 pneumococci. Some K antigens cross-react with capsular polysaccharides of Haemophilus influenzae or Neisseria meningitidis. Thus, E coli O75:K100:H5 can induce antibodies that react with H influenzae type b.
Most gram-negative bacteria possess complex lipopolysaccharides in their cell walls. These substances, cell envelope (cytoplasmic membrane, peptidoglycan, outer membrane) endotoxins, have a variety of pathophysiologic effects that are summarized in Chapter 9. Many gram-negative enteric bacteria also produce exotoxins of clinical importance. Some specific toxins are discussed in subsequent sections.
DISEASES CAUSED BY ENTEROBACTERIACEAE OTHER THAN SALMONELLA AND SHIGELLA
E coli are members of the normal intestinal microbiota (see Chapter 10). Other enteric bacteria (Proteus, Enterobacter, Klebsiella, Morganella, Providencia, Citrobacter, and Serratia species) are also found as members of the normal intestinal microbiota but are considerably less common than E coli. The enteric bacteria are sometimes found in small numbers as part of the normal microbiota of the upper respiratory and genital tracts. The enteric bacteria generally do not cause disease, and in the intestine, they may even contribute to normal function and nutrition. When clinically important infections occur, they are usually caused by E coli, but the other enteric bacteria are causes of hospital-acquired infections and occasionally cause community-acquired infections. The bacteria become pathogenic only when they reach tissues outside of their normal intestinal or other less common normal microbiota sites. The most frequent sites of clinically important infection are the urinary tract, biliary tract, and other sites in the abdominal cavity, but any anatomic site (eg, bloodstream, prostate gland, lung, bone, meninges) can be the site of disease. Some of the enteric bacteria (eg, Serratia marcescens, E aerogenes) are opportunistic pathogens. When normal host defenses are inadequate—particularly in infancy or old age, in the terminal stages of other diseases, after immunosuppression, or with indwelling venous or urethral catheters—localized clinically important infections can result, and the bacteria may reach the bloodstream and cause sepsis.
The clinical manifestations of infections with E coli and the other enteric bacteria depend on the site of the infection and cannot be differentiated by symptoms or signs from processes caused by other bacteria.
1. Urinary tract infection—E coli is the most common cause of urinary tract infection and accounts for approximately 90% of first urinary tract infections in young women (see Chapter 48). The symptoms and signs include urinary frequency, dysuria, hematuria, and pyuria. Flank pain is associated with upper tract infection. None of these symptoms or signs is specific for E coli infection. Urinary tract infection can result in bacteremia with clinical signs of sepsis.
Most of the urinary tract infections that involve the bladder or kidney in an otherwise healthy host are caused by a small number of O antigen types that have specifically elaborated virulence factors that facilitate colonization and subsequent clinical infections. These organisms are designated as uropathogenic E coli. Typically, these organisms produce hemolysin, which is cytotoxic and facilitates tissue invasion. Strains that cause pyelonephritis express K antigen and elaborate a specific type of pilus, P fimbriae, which binds to the P blood group antigen.
Over the last decade, a pandemic clone, E coli O25b/ST131, has emerged as a significant pathogen. This organism has been successful largely as a result of its acquisition of plasmid-mediated resistance factors that encode resistance to β-lactam antibiotics (elaboration of extended spectrum β-lactamases), fluoroquinolones, and aminoglycosides (see the review by Johnson et al, 2010).
2. E coli–associated diarrheal diseases—E coli that cause diarrhea are extremely common worldwide. These E coli are classified by the characteristics of their virulence properties (see later discussion), and each group causes disease by a different mechanism—at least six of which have been characterized. The small or large bowel epithelial cell adherence properties are encoded by genes on plasmids. Similarly, the toxins often are plasmid or phage mediated. Some clinical aspects of diarrheal diseases are discussed in Chapter 48.
Enteropathogenic E coli (EPEC) are an important cause of diarrhea in infants, especially in developing countries. EPEC adhere to the mucosal cells of the small bowel. Pathogenicity requires two important factors, the bundle forming pilus encoded by a plasmid EPEC adherence factor (EAF) and the chromosomal locus of enterocyte effacement (LEE) pathogenicity island that promote the tight adherence characteristic of EPEC (attachment and effacement). After attachment, there is loss of microvilli (effacement); formation of filamentous actin pedestals or cuplike structures; and, occasionally, entry of the EPEC into the mucosal cells. Characteristic lesions can be seen on electron micrographs of small bowel biopsy lesions. The result of EPEC infection in infants is characterized by severe, watery diarrhea, vomiting, and fever, which are usually self-limited but can be prolonged or chronic. EPEC diarrhea has been associated with multiple specific serotypes of E coli; strains are identified by O antigen and occasionally by H antigen typing. A two-stage infection model using HEp-2 or HeLa cells also can be performed. Tests to identify EPEC are performed in reference laboratories. The duration of the EPEC diarrhea can be shortened and the chronic diarrhea cured by antibiotic treatment.
Enterotoxigenic E coli (ETEC) are a common cause of “traveler’s diarrhea” and a very important cause of diarrhea in children less than 5 years of age in developing countries. ETEC colonization factors (pili known as colonization factor antigens [CFAs]) specific for humans promote adherence of ETEC to epithelial cells of the small bowel. Some strains of ETEC produce a heat-labile enterotoxin (LT) (molecular weight [MW], 80,000) that is under the genetic control of a plasmid and is closely related to cholera toxin. Its subunit B attaches to the GM1 ganglioside in the apical membrane of enterocytes and facilitates the entry of subunit A (MW, 26,000) into the cell, where the latter activates adenylyl cyclase. This markedly increases the local concentration of cyclic adenosine monophosphate (cAMP) after which ensues a complex cascade that involves the cystic fibrosis transmembrane conductance regulator. The end result is an intense and prolonged hypersecretion of water and chlorides and inhibition of the reabsorption of sodium. The gut lumen is distended with fluid, and hypermotility and diarrhea ensue, lasting for several days. LT is antigenic and cross-reacts with the enterotoxin of Vibrio cholerae, which has an identical mechanism of action. LT stimulates the production of neutralizing antibodies in the serum (and perhaps on the gut surface) of persons previously infected with enterotoxigenic E coli. Persons residing in areas where such organisms are highly prevalent (eg, in some developing countries) are likely to possess antibodies and are less prone to develop diarrhea on reexposure to the LT-producing E coli. Assays for LT include (1) fluid accumulation in the intestines of laboratory animals, (2) typical cytologic changes in cultured Chinese hamster ovary cells or other cell lines, (3) stimulation of steroid production in cultured adrenal tumor cells, (4) binding and immunologic assays with standardized antisera to LT, and (5) detection of the genes that encode the toxins. These assays are done only in reference laboratories.
Some strains of ETEC produce the heat-stable enterotoxin STa (MW, 1500–4000), which is under the genetic control of a heterogeneous group of plasmids. STa activates guanylyl cyclase in enteric epithelial cells and stimulates fluid secretion. Many STa-positive strains also produce LT. The strains with both toxins produce a more severe diarrhea.
The plasmids carrying the genes for enterotoxins (LT, ST) also may carry genes for the CFAs that facilitate the attachment of E coli strains to intestinal epithelium. Recognized colonization factors occur with particular frequency in some serotypes. Certain serotypes of ETEC occur worldwide; others have a limited recognized distribution. It is possible that virtually any E coli may acquire a plasmid encoding for enterotoxins. There is no definite association of ETEC with the EPEC strains causing diarrhea in children. Likewise, there is no association between enterotoxigenic strains and those able to invade intestinal epithelial cells.
Care in the selection and consumption of foods potentially contaminated with ETEC is highly recommended to help prevent traveler’s diarrhea. Antimicrobial prophylaxis can be effective but may result in increased antibiotic resistance in the bacteria and probably should not be uniformly recommended. When diarrhea develops, antibiotic treatment effectively shortens the duration of disease.
Shiga toxin-producing E coli (STEC)
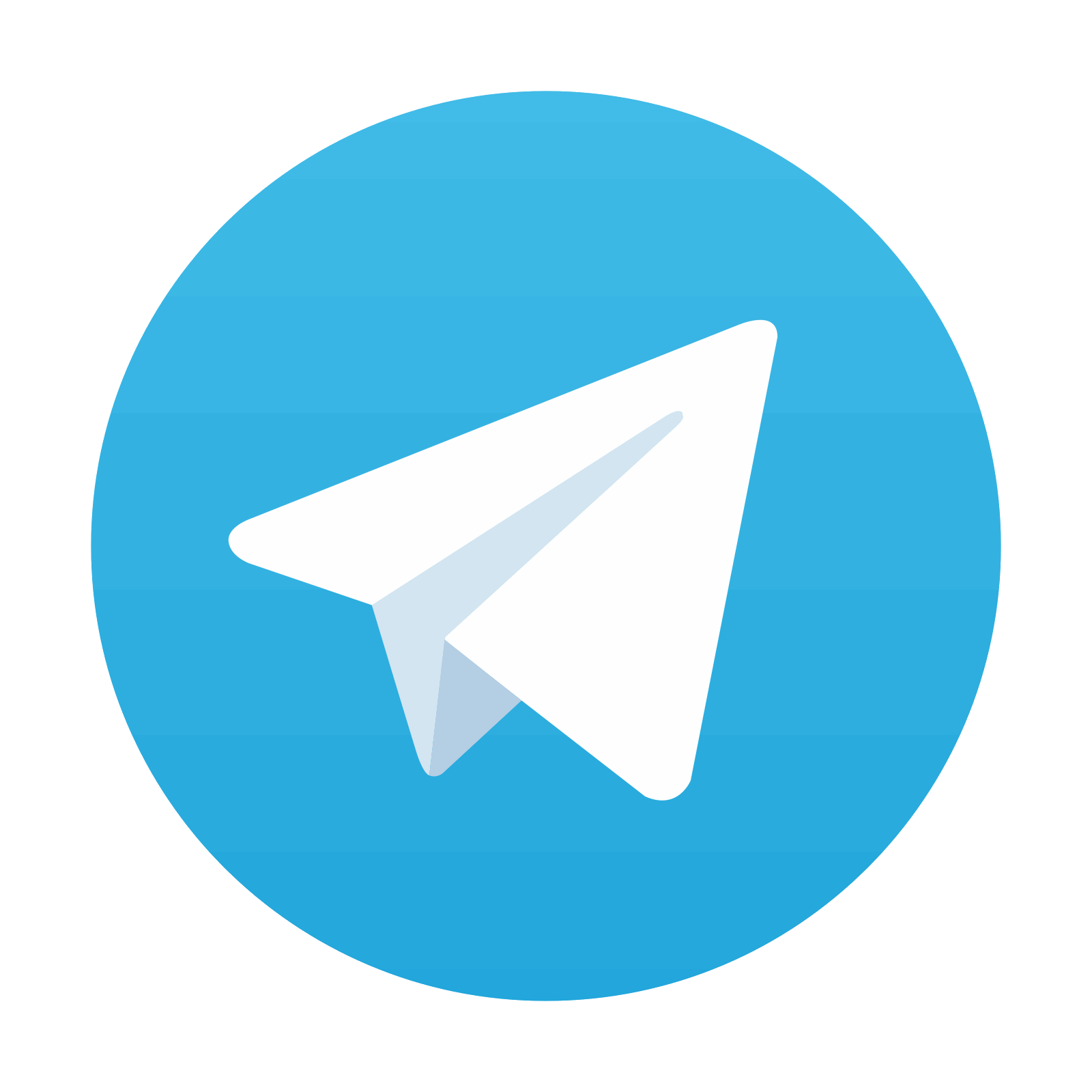
Stay updated, free articles. Join our Telegram channel

Full access? Get Clinical Tree
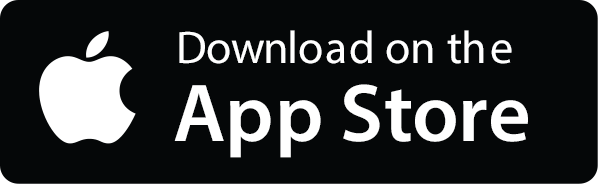
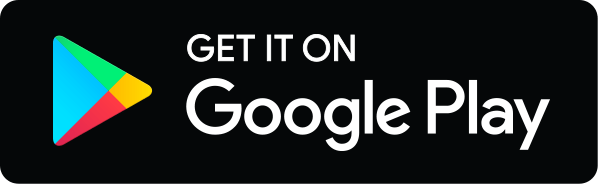
