Manufactured nanomaterial
Cell model/species
Mechanisms/effects
References
Barium sulphate
BaSO4
Ion release
Protein corona
Carbon nanotubes, multiwalled (MWCNs)
C
Human T cells (mitogen-stimulated)/healthy and allergic humans
Induction of IFN-g (healthy subjects)
Inhibition of IL-5 (allergic subjects)
Carbon nanotubes, single–walled (SWCNTs)
C
Toll-like receptor
Induction of IL-1
Cobalt
Co
3T3 fibroblasts/
Ion release
BALB/c mice
Protein corona
Fullerene C60
C
–
Toll-like receptors
Apten
Nickel oxide
Ni
ROS, catalytic activity
[31]
Palladium
Pd
Peripheral blood mononuclear cells/human, healthy
ROS, catalytic activity, induction of IFN-g
Silver
Ag
–
Ion release
[18]
Titanium dioxide
TiO2
Peripheral blood mononuclear cells/human
ROS, apoptosis
Personal data
Zinc oxide
ZnO
Macrophages
Ion release, impairment of cell function
[17]
3.3 Allergy-Related Biological Mechanisms of Nanomaterials in Animal Models
It has been shown that NPs can cause specific immune reactions, immunosuppression and autoimmunity in animal models. Mouse and rat are considered well suited to mimic the basic immunological mechanisms of allergic sensitisation, atopic/contact dermatitis and asthma development. However, the validity to predict the sensitising and asthmogenic health risk for NP-exposed workers is not straightforward. In fact, the sensitivity against NPs seems to depend on the mouse strain [42]. Furthermore, the study design is crucial to obtain relevant data from animal models. In this regard, it seems that exposure to the test NPs should be done during the sensitisation and challenge phases. Moreover, studies should focus on the use of agglomerate/aggregate NPs. Even if not completely following such criteria and not using standardised NPs, a consistent amount of data are emerging from animal studies regarding allergy. The exposure route seems to be relevant for the observed findings: NPs cause inflammation of the lung if inhaled or instilled into the trachea or worsen the allergic airway inflammation and act as Th2 adjuvant following pulmonary exposure [43].
Some types of engineered nanomaterials induce immune responses in the lung; in particular, they appear to alter the allergen-induced eosinophilia [44] by modulating the balance between Th1, Th2 and Th17 cells [28].
Copper NPs are the most potent inducers of inflammation of the lung in a model of pulmonary bacterial infection sustained by neutrophils and inflammatory cytokines, an effect also caused by iron oxide NPs [45].
Adjuvant properties of NPs could have the undesirable effect of upholding allergic sensitisation. For instance, 3-day long subcutaneous or inhalant administration of carbon black NPs (22 and 39 nm) to transgenic mice (DO11.10) expressing the T cell receptor specific for the immunodominant peptide of ovalbumin (peptide 323–339) favours the sensitisation; in fact, secondary responses, induced in peptide-restimulated splenocytes in vitro, comprise Th2 cytokines (IL-4, IL-10 and IL-13) and reduced expression of the transcription factor Stat4, specific for Th1 cells. Notably, the authors find a relationship between the size of the particle and the magnitude of the effect. However, in this study, equal mass of the two types of NPs were compared implying that the total reactive surface was much higher for the smaller ones, accounting for the higher intensity of the observed responses. Nevertheless, carbon black NPs indeed showed a Th2 adjuvant effect in this animal model [46]. Also carbon nanofibres have IgE adjuvant capacity but are less potent than nanotubes in promoting allergic airway responses [47]. Engineered silica NPs act as adjuvants enhancing allergic airway disease in mice [48].
The direct implication of NPs in the exacerbation of pre-existing type I allergy has been shown in rats sensitised to ovalbumin. Intratracheally administered silicon dioxide NPs (nonspherical, 10–20 nm, 140–180 m2·g−1) induced increase of IL-4 in the lung tissue, airway remodelling and worsening of the respiratory parameters, with more pronounced effects at the highest dose (80 μg·ml−1) [49]. Notably, such adverse effects on lung function occur with or without ovalbumin immunisation [49]. These findings suggest that NPs may have a direct role in the development of bronchial asthma, not only as an exacerbating factor of a pre-existing allergic condition. Zinc oxide NPs provide an adjuvant effect to ovalbumin via a Th2 response [50], involving TLRs and Src signalling [51], and induce eosinophilic airway inflammation [44]. Moreover, these NPs (21 nm) do not affect oral tolerance [52].
A paradigm of the potential pro-allergic effect of nanoparticles comes from a study on cerium dioxide NPs, used for medical applications and also present as side products in the exhaust gas from diesel engines, able to generate multiple types of water-soluble NPs of concern for environmental and in vivo effects. Indeed, they are strongly suspected to promote allergic diseases since selectively taken up from alveolar macrophages and able to induce an inflammatory state [53].
Silver NPs have been associated with exacerbation of airway hyperactivity. Their role in the allergic sensitisation has been studied in experimental asthma induced by ovalbumin: the animals were exposed to 3.3 mg/ml NPs (33 nm) continuously inhaled; in both groups of healthy and sensitised mice, these NPs caused infiltration of neutrophils and lymphocytes in the airways (bronchocentric) and in the peritoneum at the sites of higher accrual; moreover, it induced elevation of mediators and effectors of the allergic response (total IgE, leukotriene E4 (LTE4), Th2 cells, IL-13 and oxidative stress) [54] and significant increase in allergen-specific IgE [55]. This same detrimental effect on the allergic airways was associated with altered VEGF signalling pathway and mucus hypersecretion [56]. Low-dose intratracheal instillation (0.2 mg per rat) of aggregates of nickel oxide NPs (26 nm) induces a transient increase in chemokines associated with relentless lung inflammation and in the expression of cytokines involved in allergy inflammation and fibrosis [57].
The case of iron oxide NPs is very interesting because the allergic response is suppressed or enhanced depending on the particle dose: in fact, iron oxide NPs intratracheally administered before and during ovalbumin sensitisation significantly inhibit the allergen-specific Th2 immune response at the two highest doses (4 × 250, 4 × 500 μg per mouse), whereas the lowest dose (4 × 100 μg per mouse) promotes the allergic response [58].
It is worth pointing out also that dermal exposure to combination of agglomerates of silica NPs and mite allergens induced an IgE-biased immune response and an increased sensitivity to anaphylaxis in not pre-sensitised mice [59].
Titanium dioxide NPs, the most produced amongst nanomaterials, are regarded as relatively nontoxic at the concentration levels of the occupational environments [60]. However, studies on inhalation exposure found this type of NPs to alter the inflammatory response in asthmatic mice [61]; moreover, they aggravate atopic dermatitis (AD)-like skin lesions in mice [62]. Lung injury-inducing high doses (32 mg·kg−1 twice a week for 4 weeks) of titanium dioxide NPs are not associated with significant changes of IL-4 in the lung, ruling out the involvement of an allergic reaction in the mechanism of damage [63]. Route-dependent immune effects are observed following exposure to titanium dioxide-NP immune effects [64]. Also the sensitisation status seems to determine different outcomes: ovalbumin-induced allergic pulmonary inflammation characterised by eosinophil infiltration in the lung and IL-4 is reduced upon inhalation of (agglomerated) titanium dioxide NPs (32 mg/ml for 6 and 42 h post-sensitisation) in asthmatic rats [65]; in non-sensitised rats, TiO2 NPs (75 % anatase, 25 % rutile, 21 nm, aggregates of 200 nm and 2 μm, 5 mg·kg−1) intratracheally instilled once provoke acute airway inflammation with transitory IL-4 production, replaced by a durable Th1 response [22]. In a mouse model, instead, the same component instilled at 5–50 mg·kg−1 (150 μm aggregate mean size) induced a Th2-mediated chronic inflammation, evidencing a species-related bias of the immune response [66].
Polystyrene NP application to atopic dermatitis-like skin lesions (related to mite allergen) induces local size-dependent increase of protein levels of IL-4 and various chemokine ligands for monocytes and macrophages recruitment [67], whereas, on normal skin, it induces sensitisation [68]. In the same AD (atopic dermatitis), an aggravation of the pathological condition is induced size dependently by amorphous silica NPs upon intradermal injection [69].
NPs of nickel oxide (NiO) and cobalt oxide (Co3O4) induce lung-delayed hypersensitivity-like responses in mice [70], whereas hydroxyapatite NPs do not produce any effect in the guinea pig model of this pathology [71]. Allergy-relevant mechanisms of action and effects of the manufactured NPs based on in vivo findings are summarised in Table 3.2.
Table 3.2
List of principal manufactured nanomaterials with high industrial burden and mechanisms favouring allergic diseases triggered by exposure described in animal studies
Nanomaterial | Formula | Production and usage sectors | Animal model | Exposure route | Mechanism | Biological/pathological outcome | References |
---|---|---|---|---|---|---|---|
Cerium dioxide | CeO2 | Medical applications | CD-1 mouse | Intratracheal | Release of water-soluble nanoparticles | Selective uptake by alveolar macrophages | [53] |
Pro-inflammatory | |||||||
Cobalt oxide | Co3O4 | Sensors, energy, drug delivery | Healthy rats | Lung instillation | Apten | Delayed-time hypersensitivity | [70] |
Copper | Cu | Electrical, engineering, transport, building and construction | Pulmonary bacterial infection in mice | Inhalant | Pro-inflammatory | Strong lung inflammation sustained by neutrophils and inflammatory cytokines | [45] |
Carbon black | C | Tyres, printer toner, dyes for leather or textiles, cosmetics | Transgenic mice (OVA-specific T cell receptor) | Intratracheal | Th2 adjuvant | IL-4, IL-10 and IL-13 | [46] |
Subcutaneous | Th1-specific Stat 4 transcription factor | ||||||
Carbon nanofibres | C | Power, wind energy, aeronautical, sports, construction, chemical, automotive | Healthy mice | Intratracheal | IgE adjuvant | Allergic airway disease | [47] |
Iron oxide | FeO | Glass, paints, coatings, automotive | Healthy mice | Intratracheal | Neutrophil, inflammatory cytokines | Lung inflammation | |
Nickel oxide | NiO | Microelectronics, aerospace materials, batteries, electrochromic coatings and materials | Healthy rats | Intratracheal | Pro-inflammatory | Th2 cytokines | |
0.2 mg per rat (low) | |||||||
chemokines | |||||||
Fibrosis | |||||||
Sustained lung inflammation | |||||||
Delayed-time hypersensitivity | |||||||
Polystyrene | (C8H8)n | Atopic dermatitis mouse model | Healthy mice | On skin lesion | Pro-inflammatory | Site-dependent IL-4, chemokines | |
On normal skin | |||||||
Sensitisation | |||||||
Silicon dioxide | SiO2 | Concrete, plastics, synthetic materials | OVA-sensitised rats | Intratracheal | Adjuvant of type I allergy | Induction of IL-4 | |
140–180 m2·g−1 | |||||||
Allergic airway disease (only sensitised) | |||||||
Healthy rats | |||||||
Adverse effect on lung function | |||||||
Silver | Ag | Plastics, synthetic materials | OVA-sensitised mice | Inhalant | Th2 adjuvant | Induction of IL-4, IL-13, total IgE, allergen-specific IgE, LTE-4, Th2 cells, neutrophils | |
7 days continuously | |||||||
Oxidative stress | |||||||
Exacerbation of airway hyperactivity | |||||||
VEGF | |||||||
Mucus hypersecretion | |||||||
Pro-inflammatory | |||||||
Healthy mice | |||||||
3.3 mg·m−3 | |||||||
Titanium dioxide | TiO2 | Cosmetics and paint | AD mouse model | Topical | ROS, apoptosis | Aggravation of AD | |
Inhalant | |||||||
Lung injury (IL-4 independent) | |||||||
Intratracheal | |||||||
Healthy mice | |||||||
Th1 response/Th2-mediated inflammation | |||||||
Healthy rats/mice | |||||||
OVA-sensitised mice | |||||||
Zinc oxide | ZnO | Cosmetics and paint | OVA-sensitised mice | Th2 adjuvant | Eosinophilic airway inflammation | ||
AD mouse model | Induction of IgE |
Interestingly, relevant NPs in the field of advanced technologies, such as silver NPs and fullerene C60, own intrinsic capacity useful to control the allergic reactions; for instance, fullerene C60 was found to prevent the histamine release in a mast cell-dependent anaphylaxis model [72], and silver NPs (5 nm) reduce hyperreactivity in a murine model of airway allergic inflammation, perhaps due to its antioxidant and anti-inflammatory activities [73]. Topically applied zinc oxide NPs (ZnO) suppress allergen-induced skin inflammation but induce vigorous IgE production in the atopic dermatitis mouse model [17].
3.4 Occupational Allergic Diseases with Potential Nanomaterial Aetiology
Taking into account the global experimental information gathered so far on the biological impact of nanomaterials, for exposed workers, the risk of developing nanoparticle-exposure associated pathologies cannot be totally ruled out, as well as exacerbating pre-existing pathologies. Moreover, it is conceivable that adverse health effects may especially develop in hypersensitive populations, such as individuals with respiratory diseases (Fig. 3.1). Whatever form and chemical species they acquire inside the body, engineered nanomaterials can induce oxidative stress responsible for lung diseases [74] attributable to their particulate physical form. Pleural effusion, pulmonary fibrosis and granuloma can be related to exposure to NPs [75]. The possibility of aggravation of chemically induced occupational asthma in the presence of NP exposure is suggested by recent findings showing that a very low dose (approx. 0.8 mg·kg−1) of intrapulmonary gold or titanium dioxide NPs modulates a non-IgE-mediated asthma [76, 77] (Fig. 3.1). The pro-inflammatory activity shown by most nanomaterials makes plausible the hypothesis that the occupational exposure might provide stimuli able to trigger sarcoidosis-like illness (giant cell-like nodules), typically affecting individuals exposed to foreign antigens and to inorganic particulates and recognised and compensable as work-associated disease [78].


Fig. 3.1
Potential influence of NPs in the development of allergic and intrinsic asthma, as suggested by in vitro and in vivo findings. Pathways and molecules possibly affected by NPs activity are coloured in red (Modified from [89])
It is well known that occupational asthma may be caused by metals (e.g. cobalt, platinum, chromium, vanadium, nickel) and that sensitisation to the professional agent is one of the known aetiopathogenetic mechanisms triggering this immunologically mediated disease. It has been found that also nanosized metals own pro-sensitising effects in vitro and in vivo, suggesting a potential role in the development of this life-threatening professional disease (Fig. 3.1). The most challenging of them regards the possibility of acting as such, as aptens or as released ions once inside the body.
Even more concerning, a role cannot be excluded for nanoparticles in the exacerbation of pre-existing eosinophil-mediated allergic diseases of nonoccupational aetiology, such as rhinitis, atopic dermatitis and food allergy, as well as other Th2-mediated nonallergic pathologies, such as aspergillosis, eosinophilic gastroenteritis and Churg-Strauss syndrome (Figs. 3.1 and 3.2).


Fig. 3.2
Potential role of NPs on allergen-induced airway inflammation, as sustained by in vitro and in vivo data. Cell types, pathways and molecular mediators emerging to be influenced by NPs are coloured in red (Modified from [89])
Pro-sensitising effects, as well as other unpredictable immunological effects, might be worrying for those workers of pharmaceutical industries producing nanovaccines made of NPs loaded with immunogenic proteins to be used for immunotherapy or functionalised on the surface to generate immunoactivating compounds for anticancer therapy. Several anti-allergy nanovaccines have been designed so far with the purpose of switching off the detrimental immune response towards an allergen. They consist of porous and/or polymeric substances (PLGA, dextran, silica) with adjuvant activity (either intrinsic or conferred by LPS) that are loaded [17, 79, 80] or agglomerated [81] with the therapeutic allergen. In vitro and in vivo experimental testing of these products has been carried out recently. The results suggest beneficial effects of these nanovaccines in allergic asthma and atopic dermatitis model that appear to rely on the potentiated adjuvancy given by the NP component. In particular, the treatment with PLGA profilin modifies the Th2/Th1 cytokine balance and inhibits the differentiation of eosinophils [79]; dextran-NP ovalbumin activates the antigen-presenting cells and induces potent immunomodulation and proliferative responses of allergen-specific T cells (experimentally grafted in mice) [80]. Moreover, zinc oxide NPs, designed for local treatment of atopic dermatitis, are able to suppress allergen-induced skin inflammation in the mouse model of this disease but also to induce strong IgE production [17].
A very high rate of immunoactivation of bone marrow-derived dendritic cells (MDDCs) and T cells is achieved experimentally by exposing these cells to porous silicon nanoplatforms designed for immunotherapy, with dependency on surface chemistry [82].
3.5 Nanoparticle Sensitising Effects in Humans
Data on the effects of occupational exposure to NPs are very limited. In fact, their ability to induce sensitisation has been investigated for a chronic nonoccupational exposure due to hip replacement with cobalt-chromium-coated polyethylene NP-based prosthesis, characterised by strong metal ion release. There was a significant increase of the two metals in the urine of the subjects who had received this type of prosthesis, compared with those who had received the standard non-NP type; however, no relative increase of metal allergy was observed, with a follow-up of 5 years after surgery [83]. Even though this study does not attribute to in vivo NPs with a higher sensitising potential compared to non-nanomaterials, this conclusion is made on a limited number of subjects and a short time of observation, while the long-term effects are not predictable [83]. Recently, a first human study of a systemically ingested silver-NP product [84] has been carried out. Also in this case, silver was present in serum supposed to be mostly in the ionic form, since after 14 days no evidence was found of intact silver NPs absorbed through the human digestive tract or attached to blood components. Moreover, no clinically significant changes in metabolic, haematologic, urine and physical findings, sputum morphology and/or imaging changes were observed. Hence, even for exposed workers, ion released from metal NPs could represent a potential allergic/health threat.
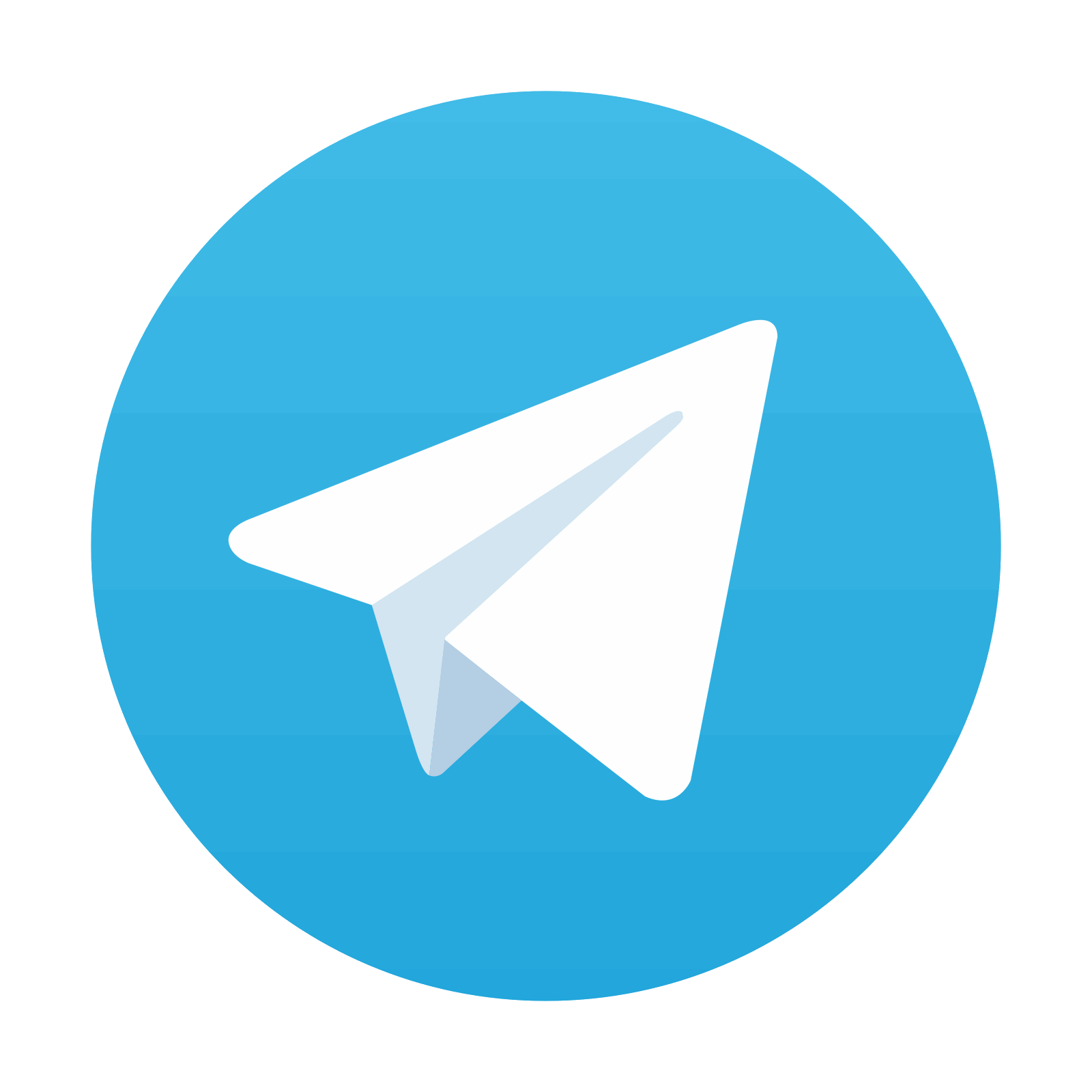
Stay updated, free articles. Join our Telegram channel

Full access? Get Clinical Tree
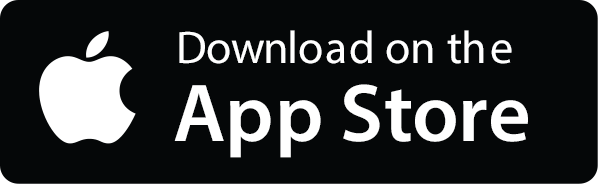
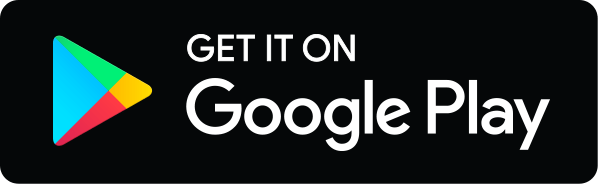