Chapter 8 Endocrine physiology
Endocrine regulation is an important element of homeostatic control. Integration of endocrine and nervous controls is a major function of the hypothalamus. These neuroendocrine links will be considered in some detail, along with the actions of various hormones which regulate cell metabolism or some aspects of fluid and electrolyte balance. Other functions which are also under endocrine control are considered elsewhere, e.g., cardiovascular performance (Ch. 3), renal function (Ch. 5), gastrointestinal motility and secretion (Ch. 6) and reproductive physiology (Ch. 9).
8.1 Principles of endocrine function
Endocrine control involves secretion of a hormone into the circulation. This messenger molecule binds to any cells which carry the relevant receptors, initiating the observed response (Section 1.5). As far as the target cell is concerned this is similar to other forms of chemical signalling, e.g., chemical neurotransmission. Indeed, a given substance may act both as a neurotransmitter and as a hormone at different sites. For example, noradrenaline (norepinephrine) is a postganglionic sympathetic neurotransmitter but is also released into the circulation as a hormone from the adrenal medulla (Section 7.6). Because of the large diffusion distances and circulation delays involved, however, hormonal responses are generally slower in onset than those mediated by nerves. They are also more persistent, since removal of the hormone from the bloodstream may take some time after secretion has stopped.
The release of endocrine substances is controlled in three ways:
Control by a regulated solute
Changes in a controlled variable may directly trigger changes in hormone levels; e.g., the cells which secrete parathormone, which acts to reduce the concentration of Ca2+ in the extracellular fluid, are themselves directly sensitive to [Ca2+] (Section 8.4). This is an example of a classical negative feedback mechanism.
Control by a different hormone
Secretion may be regulated by another hormone, e.g., several pituitary hormones stimulate secretion from other endocrine glands. This is also often under negative feedback control, with inhibition of pituitary secretion by the product hormone. Positive feedback may occur, however, leading to a rapid rise in hormone levels, e.g., prior to ovulation in the female reproductive cycle (Section 9.4).
8.2 Hypothalamic and pituitary function
At the end of this section you should be able to:
The hypothalamus regulates both autonomic nervous activity (Section 7.7) and several aspects of endocrine function. The latter role is fulfilled through its links with the pituitary gland, which secretes a wide range of hormones. Some of these regulate human physiology directly, while others control the activity of various endocrine glands around the body. The result is a multitiered endocrine control system which can be influenced through the nervous inputs to the hypothalamus.
Relevant structure
The hypothalamus is located adjacent to the third ventricle in the forebrain and is connected by the hypophyseal stalk to the pituitary gland (hypo-physis cerebri) immediately inferior to it (Fig. 159). This has two embryologically distinct components.
Hypothalamic control of pituitary function
This is different for the anterior and posterior lobes of the pituitary.
Anterior pituitary
The endocrine activity of the anterior pituitary is regulated by hormones secreted from the hypothalamus and transported in the hypophyseal portal blood. Each of these factors selectively promotes or inhibits the secretion of a specific pituitary hormone and so they are referred to as releasing or inhibiting hormones, respectively. They are generally oligopeptides, although prolactin-inhibiting hormone is probably the transmitter substance dopamine. Hypothalamic secretion of these pituitary-regulating hormones is influenced by neurological inputs and feedback control. Both the pituitary hormones themselves and circulating products of the endocrine systems controlled by those hormones can regulate hypothalamic secretion, usually through feedback inhibition (Fig. 160).
Posterior pituitary
The posterior pituitary secretes two peptides which are manufactured in the cell bodies of hypothalamic neurones (Fig. 159). These hormones are packaged in vesicles and transported along the axons into the posterior pituitary itself to be stored in the axon terminals. Appropriate sensory stimuli lead to activation of the hypothalamic cells and the release of the hormones from the posterior pituitary into the bloodstream. This adaptation of neurotransmission to fulfil an endocrine role is sometimes called neurocrine control.
Anterior pituitary hormones
Follicle-stimulating hormone (FSH) and lutein-izing hormone (LH) are secreted in response to gonadotrophin-releasing hormone (GnRH) from the hypothalamus. These gonadotrophins stimulate the male and female gonads (Sections 9.2 and 9.4).
Prolactin secretion may be influenced by a prolactin-releasing hormone (PRH) but is mainly controlled by a prolactin-inhibiting hormone (PIH), (this is probably dopamine). Stimuli which reduce PIH release from the hypothalamus raise prolactin levels. This occurs during pregnancy, favouring development of the breasts ready for lactation, and as part of the suckling reflex during breast feeding. The resultant prolactin peaks stimulate milk production (Section 9.5). Prolactin also inhibits GnRH secretion from the hypothalamus, thus inhibiting the reproductive cycle during lactation.
Posterior pituitary hormones
Oxytocin is produced by cells in the paraventricular and supraoptic nuclei of the hypothalamus. These are reflexly activated by sensory inputs from mechanoreceptors in the breast during suckling, and oxytocin is released from the posterior pituitary in response. This stimulates the ejection of milk through contraction of the myoepithelial cells surrounding the milk ducts (Section 9.5). Oxytocin also stimulates uterine contraction during labour (Section 9.5).
Antidiuretic hormone (ADH) is also produced in the paraventricular and supraoptic nuclei of the hypothalamus. Sensory inputs, both from local osmoreceptors and from stretch receptors in the cardiovascular system (the cardiopulmonary and systemic arterial baroreceptors), stimulate these cells whenever the osmolality of the extracellular fluid rises, or if blood volume or blood pressure falls. The resulting increase in ADH promotes water reabsorption from the collecting ducts and distal convoluted tubules of the kidney (Section 5.4), and so tends to reduce the osmolality and expand the volume of the extracellular fluids by promoting the production of a small volume of concentrated urine. At the same time, peripheral resistance is increased through arteriolar constriction, which also helps to maintain arterial pressure (Section 3.6). This pressor effect, though less important physiologically, explains the derivation of ADH’s alternative name, which is vasopressin.
8.3 Thyroid function
Relevant structure
The thyroid gland is located in the neck, in front of and just below the level of the larynx, and consists of two lobes joined by a central isthmus. Histologically, it consists of numerous spherical follicles, each with an outer layer of cuboidal epithelium and filled with proteinaceous colloid (Fig. 161A). These follicles represent the functional subunits of the gland, responsible for synthesis, storage and release of the thyroid hormones. The thyroid also contains parafollicular C cells, which secrete calcitonin. These will be considered along with other aspects of body calcium regulation (Section 8.4).
Box 34 Clinical note: Abnormal pituitary function
Deficient secretion
Excess secretion
Hormone synthesis
The first step in the synthesis of the thyroid hormones involves active pumping of iodide ions (I−) from the extracellular space into the follicular epithelium (Fig. 161B). The trapped I− enters the colloid and is oxidized to iodine, which then combines with tyrosine molecules attached to a colloid-binding protein known as thyroglobulin. Monoiodotyrosine (MIT) and diiodotyrosine (DIT) are generated as a result. These then combine to produce the hormonal products, triiodothyronine (1 MIT + 1 DIT) and thyroxine (2 × DIT). These thyroid hormones may be stored in the colloid for some months but are eventually detached from the thyroglobulin and released into the bloodstream. The quantity of thyroxine (T4) produced greatly exceeds that of triiodothyronine (T3), but the latter is more biologically active.
Regulation of thyroid secretion
Thyroid activity is controlled by the hypothalamus and anterior pituitary and provides a classical example of the feedback loops typical of such regulation (Section 8.2; Fig. 160). The hypothalamus releases thyrotrophin-releasing hormone (TRH) into the hypophyseal portal blood and this stimulates secretion of thyroid-stimulating hormone (TSH) from the anterior pituitary. Thyroid hormone synthesis and secretion are both stimulated by TSH. Normal levels of circulating T3 and T4 are maintained through their negative feedback effects on TRH and TSH secretion. Other stimuli may also influence secretion, e.g., cold conditions may stimulate hypothalamic TRH release as part of temperature homeostasis (Section 1.1).
Whole body actions of thyroid hormones
Box 35 Clinical note: Abnormal thyroid function
This leads to inadequate or excess amounts of circulating thyroid hormone.
Deficient secretion
Excess secretion
Hyperthyroidism, or thyrotoxicosis, can arise in two main ways.
8.4 Hormonal control of Ca2+
Calcium pools in the body
There are three main body calcium pools (Fig. 162):
Intracellular pool
The size of the intracellular Ca2+ pool is hard to estimate since much of it is normally bound to proteins within stores of unknown capacity. It is clear, however, that changes in cytoplasmic [Ca2+], whether caused by release of stores, as in skeletal muscle (Section 1.6), or by entry of Ca2+ from the extracellular fluid, as at the axon terminal (Section 7.2), act as important intracellular signals in controlling a wide variety of cell functions.
Extracellular pool
Calcium in the extracellular fluid is involved in continuous exchange with that in bone and body cells (Fig. 162). Absorption from the gut and urinary excretion also occur directly into and out of the extracellular space. All these processes are affected by the extracellular [Ca2+]. Even more importantly, extracellular Ca2+ is crucial in determining the ease with which excitable cells can be stimulated and so plasma [Ca2+] must be closely regulated. The normal value is about 2.5 mmol L−1 but approximately half of this is bound to protein. The remainder consists of Ca2+ free in solution, and it is only this fraction which is biologically active.
Physiological functions of extracellular Ca2+
The excitability of nerve and muscle is increased by a fall in plasma [Ca2+] (hypocalcaemia). This may result in spontaneous skeletal muscle contraction (which can be fatal because of laryngeal spasm and respiratory arrest), cardiac arrhythmias and abnormal sensations caused by spontaneous sensory nerve activity (paraesthesia). The mechanism underlying these effects is an increase in ion channel permeability to Na+ in the presence of low extracellular [Ca2+]. This promotes inward Na+ currents so that the membrane depolarizes towards the threshold for action potential production. Conversely, a high extracellular [Ca2+] depresses nerve and muscle activity by reducing the Na+ current (Section 1.4).
Excitation–contraction coupling in muscle depends on an increase in intracellular [Ca2+] (Section 1.6). Smaller decreases in extracellular [Ca2+] than those which stimulate spontaneous excitability may reduce the strength of contraction, both by decreasing the influx of Ca2+ during the action potential and by depleting the intracellular stores within the sarcoplasmic reticulum.
Blood clotting is dependent on plasma Ca2+, which acts as an essential clotting factor (Section 2.6).
Regulation of body Ca2+
Parathormone (PTH)
Vitamin D
Vitamin D is a fat-soluble vitamin which comes from two main sources, the diet (vitamin D2 or ergocalciferol) and the skin (vitamin D3 or cholecalciferol). These two forms differ slightly in structure but fulfil identical functions. The main dietary sources are fish, liver and ultraviolet (UV) irradiated milk, and D2 is absorbed along with lipid in the small intestine. Alternatively, UV radiation from sunlight can convert a cholesterol derivative into vitamin D3 in the skin. The relative importance of these sources in a given individual largely depends on the local climate.
Vitamin D acts to elevate plasma levels of both Ca2+ and phosphate. It achieves this by:
8.5 Functions of the adrenal cortex
Relevant structure and biochemistry
Hormone synthesis and excretion
Mitochondrial conversion of cholesterol to pregnenolone provides a common biochemical platform leading to the production of aldosterone (the main mineralocorticoid), cortisol and corticosterone (the main glucocorticoids), and adrenal androgens (Fig. 163). Following release, a considerable fraction of these lipophilic (hydrophobic) adrenal steroids is bound to plasma proteins. Eventually the circulating hormones are broken down in the liver and the metabolites are conjugated with glucuronic acid prior to excretion in the faeces and urine. This explains why conditions associated with excess production of adrenal corticosteroids may be usefully diagnosed by measuring urinary excretion of specific metabolites.
< div class='tao-gold-member'>
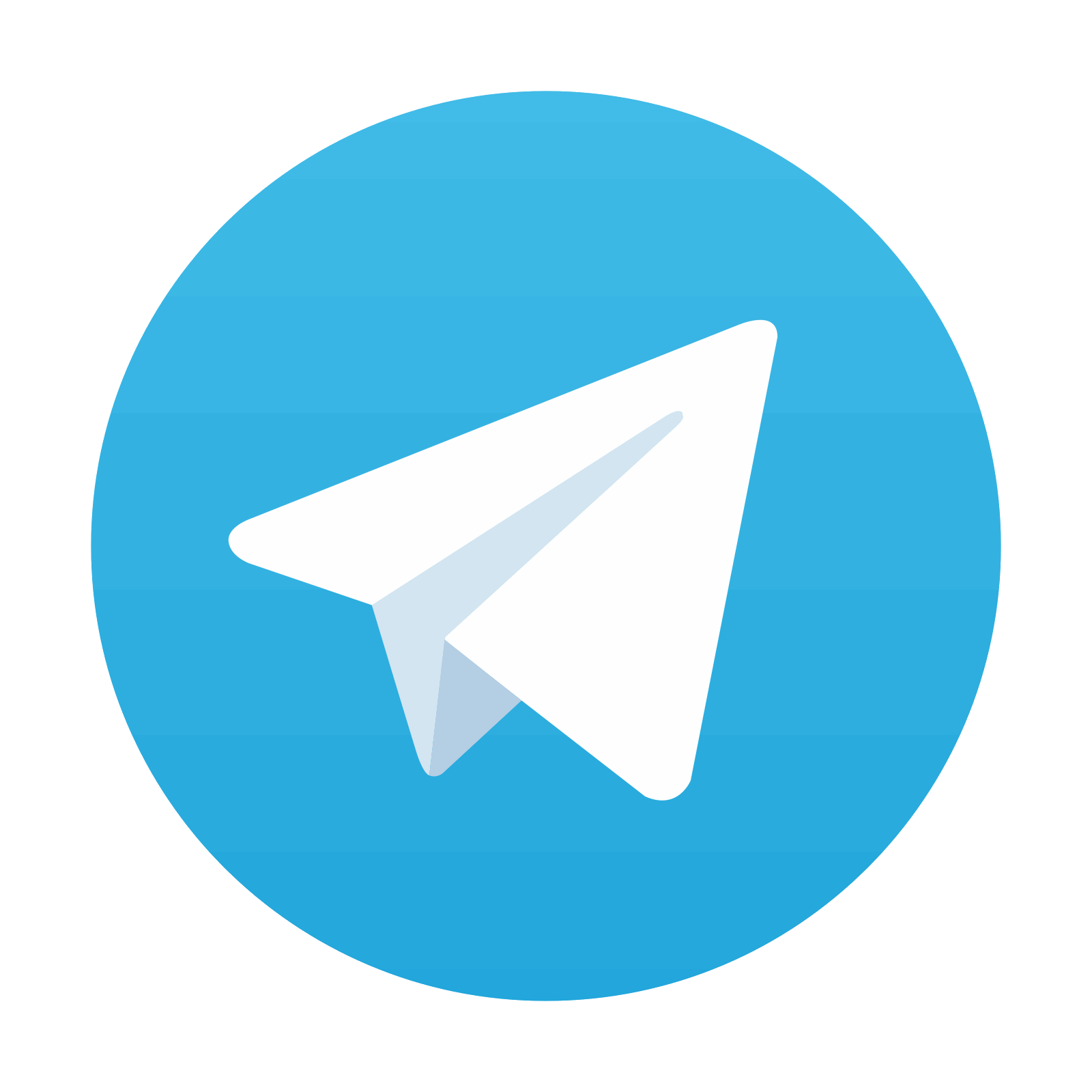
Stay updated, free articles. Join our Telegram channel

Full access? Get Clinical Tree
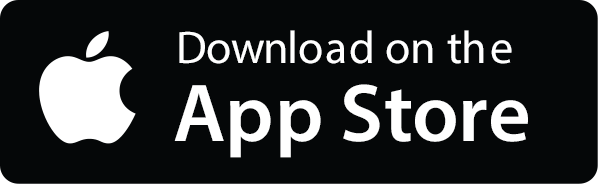
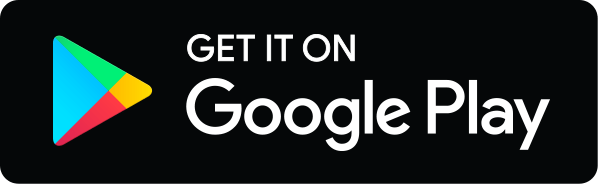