Clinical steps
Etching
Primer
Resin
Etch-and-rinse (3-steps)
Phosphoric acid (30–35 %)
HEMA, organic solvent (ethanol/acetone/water), proprietary monomers
Hydrophilic and hydrophobic monomers (HEMA, TEGDMA, Bis-GMA, UDMA), initiators
Etch and rinse (2-step)
Phosphoric acid (30–35 %)
Hydrophilic and Hydrophobic monomers (HEMA, TEGDMA, Bis-GMA, UDMA, proprietary monomers), organic solvent (ethanol/acetone/water), fillers, initiators
Self-etching (2-step)
Functional and hydrophillic acidic monomers (Phenyl-P, 4-MET, 10-MDP, MDPB and HEMA), initiator, solvents and water
Functional, hydrophilic and hydrophobic monomers (Bis-GMA, TEGDMA), initiator
Self etching (1-step)
Functional, hydrophilic acidic and hydrophobic monomers (4 META, Phenyl-P, 10-MDP, HEMA, Bis-GMA, UDMA), initiators, solvents and water
Universal
Optional Phosphoric acid (30–35 %)
Functional, hydrophilic and hydrophobic monomers(10-MDP, HEMA, Bis-GMA, TEGDMA), proprietary monomers, solvent, initiator
The basic components of a dental bonding system include a primer, the adhesive resin, an organic solvent and polymerization initiators. Primers contain hydrophilic blends of resin monomers/co-monomers. Adhesive resins contain blends of hydrophobic monomers/co-monomers. Solvents are added to the systems to enhance resin infiltration into the tissue, whereas photoinitiators are commonly used for convenient operator-controlled photopolymerization of the adhesive. Other ingredients such as fluoride, glutaraldehyde, antimicrobials are also commonly added to the mixture in an attempt to further protect or strengthen the adhesive interface.
The adhesion mechanism is tissue-dependent; in general bonds with greater clinical durability are achieved on enamel surfaces when compared to dentin. The surface treatment, adhesive chemistry, application protocol and different forms of enamel and dentin are also determinant factors in the adhesion process. The main mechanism of bonding to sound enamel is the formation of resin microtags following infiltration of resin monomers into a superficially decalcified microstructured prismatic layer. In dentin, resin tags can also be formed and it is estimated that 60–80 % of the bond strength to dentin is provided by the formation of the hybrid layer, which is the impregnation of the collagen network with the adhesive resin (further details below). The complexity in composition and structure of dentin is a major obstacle for proper interfacial sealing and high bond strength overtime. A proof to the importance of the hybrid layer was poor success rate of earlier dental adhesive systems that did not bond to dentin. Due to the importance of dentin to the extended service-life of a restoration, novel materials have been developed based on high affinity for the dentin structure components. The process of hybrid layer formation is described below.
The Process of Hybrid Layer Formation
In order to properly bond to dentin, resin monomers must interact with the dentin matrix. The term hybrid layer is used to describe the physical interaction between the resin and the demineralized dentin. Adhesion in dentin is mainly obtained by micromechanical inter-locking of cured resins and the exposed dentin collagen network. Earlier adhesive systems provided the foundation for the development of a hybrid layer in dentin. These systems called “etch-and-rinse” remove smear layer—a surface layer composed of organic and inorganic debris resulting from the drilling process—smear plugs and superficially decalcify the dentin with a separate application step of acidic etchant—generally phosphoric acid. Microscopically, a clean surface with the exposed collagen fibrils is apparent and ready for the priming step with hydrophilic-based monomers and subsequent coating with hydrophobic blends of resin monomers. Following resin polymerization, the infiltrated resin will be anchored onto the exposed dentin matrix. This complex process takes place very quickly, usually between 30–90 s.
The primer is constituted of a blend of organic solvents and hydrophilic monomers to enable proper resin infiltration. Following the priming step, a more hydrophobic adhesive layer is applied acting as a barrier for the outward water movement from the dentin tubules, also providing the necessary hydrophobicity to chemically bond the adhesive material with the resin composite, which will make up the bulk of the dental restoration. Because of the presence of water and organic components, the technique is highly sensitive. The surface of dentin must remain hydrated prior to the application of the primer to avoid collapse of the collagen exposed during decalcification. The technique was developed in the early 90s and is called wet bonding technique. Overwetting and overdrying the dentin will result in a significant decrease in the bond strength. The later generation of etch-and-rinse system simplified the technique by applying the primer/adhesive at the same time (Table 9.1).
Self-etching systems also have the ability to form hybrid layers however in a limited manner and non-uniform fashion. A thinner hybrid layer is also observed when functional acidic monomers are used to demineralize and simultaneously infiltrate the dentin matrix. The functional monomers in the adhesive blends are ionized in water and etch the dentin surface while penetrating within the collagen framework [12]. The adhesive system is further polymerized in both techniques, resulting in mechanical interlocking with the dentin matrix. Hydrophilic monomers are preferable for penetrating within the dentin matrix after demineralization. However the excess water may result in separation of the hydrophilic and hydrophobic components of the adhesive system, decreasing the mechanical properties of the resin-dentin interface [13]. Good penetration of the adhesive system enveloping the exposed collagen fibrils is important for the success of the hybridization process.
Adhesive Interface Degradation
The hybrid layer is believed to be essential for maintaining the integrity of the resin-dentin bonded interface. The complete replacement of mineral by resin monomers during infiltration is unlikely even with the use of low viscosity hydrophilic monomers and organic solvents [12]. The infiltration of adhesive into demineralized dentin is influenced by the diffusion ability of the resin monomers plus organic solvents within the dentin matrix. Complete enveloping of the dentin matrix is likely not to occur by passive infiltration of the resin monomers due to the size of the molecules and the available spaces within the collagen molecules [1, 14]. The incomplete resin infiltration affects the stability of the interface by establishing pathways for fluid penetration accelerating hydrolytic and enzymatic degradations.
In addition to the hybrid layer, critical components of the adhesive interface are the underlying dentin and the adhesive layer itself (Fig. 9.1). The biomechanical properties of these 3 components are distinct and their integrity overtime also plays an important role in the service-life of dental restorations. Hydrolytic and enzymatic degradation mechanisms are believed to be major players in the degradation of the interface components. The processes can be accelerated by the chemical, mechanical stresses in the oral environment. Some of the well-established mechanisms of interfacial degradation are described below in detail.
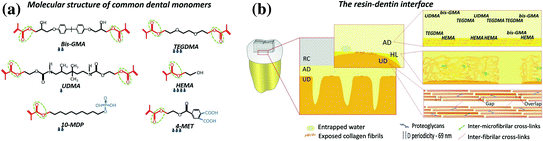
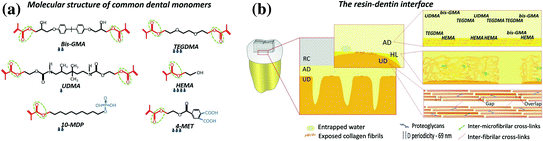
Fig. 9.1
a Typical monomer molecules used in dental adhesive systems. b Schematic of the resin dentin interface
Degradation of Resin at the Interface
The hydrolysis of monomers and breakdown of polymeric chains of methacrylate-based resins are associated with adhesive degradation. After polymerization the adhesive resins absorb water by diffusion through poorly polymerized chains and hydrophilic domains [15–17]. The distance between polymers tends to increase, allowing water to stay entrapped between the polymeric networks, decreasing the mechanical properties of the polymer [16, 18]. The decrease in the modulus of elasticity of the polymer, will allow for polymer chain movement, facilitating the swelling of unreacted monomers [19]. Water can break the ester bonds in methacrylate monomers. In addition to hydrolysis, degradation of 2,2-bis[4-(2-hydroxy-3-methacryloxypropoxy)phenyl]propane (Bis-GMA), by salivary and bacterial esterases has been reported [20, 21]. Hydrophilic monomers are used as priming agents to facilitate the diffusion of resin into the collagen rich layer in dentin. However, hydrophilic monomers such as HEMA (Hydroxyethyl Methacrylate) and diluent monomers with ethylene glycol group (i.e. TEGDMA—triethyleneglycol-dimethacrylate) greatly increase the water sorption of adhesives [22].
The clinical technique can also affect the performance of dental adhesive systems. Significant reduction in the degree of conversion and mechanical properties of adhesive systems was observed when solvents were not properly evaporated [23–26]. The application of simplified-step adhesive systems to an excessively wet dentin surface may lead to phase separation and a hydrophobic-poor and hydrophilic-rich zone may be formed, lowering the stability of the adhesive interface [13]. Acidic monomers remain active when poorly polymerized resulting in continuous etching of the underlying dentin [27].
Degradation of Dentin Matrix at the Interface
Dentin matrix is mainly composed of type I collagen fibrils, non-collagenous proteins and enzymes [1, 28]. Proteolysis of collagen and non-collagenous components are associated with loss of anchorage to the dentin reducing the bond strength and increasing permeability at the resin-dentin interface. At the adhesive interface, the organic dentin matrix can either be left exposed by incomplete resin infiltration or become exposed following degradation of adhesive components. The exposed collagen is vulnerable to hydrolytic and proteolytic degradation. Collagen degradation at the interface has been linked to host-derived enzymes that are commonly latent in fully mineralized dentin [29, 30]. More specifically, the degradation process takes place by activation of host-derived matrix metalloproteinases (MMPs) and cysteine cathepsins [31]. The MMPs are zinc and calcium-dependent proteolytic enzymes capable of degrading the organic network. Collagenolytic (MMP-1, -8 and -13) and gelatinolytic (MMP-2) enzymes, can cleave the collagen triple-helical molecule in ¼ and ¾ fragments. The denatured fragments can be further degraded by gelatinases and other non-specific tissue proteinases.
Proteoglycans (PGs), which represent about 3 % (w/v) of the organic composition of dentin, are organic structures strongly bound to collagen which play important roles on the structure and biomechanics of the matrix. The most prevalent PGs in dentin, decorin and biglycan, contain either one (decorin) or two (byglycan) glycosaminoglycan (GAG) chains attached to a core protein. In mature dentin, negatively charged GAGs provide tissue hydration and organization by interconnecting adjacent fibrils. Selective removal of GAGs and PGs results in decreased mechanical properties of mineralized dentin and also significantly affects the resin-dentin bond strength. Decrease in the resin-dentin bond strength of PGs-depleted dentin matrix has been reported following a re-wetting restorative technique [32]. Resin infiltration is compromised due to the inability of PGs- and GAGs- depleted dentin matrix to re-expand following surface desiccation. Reports have also shown that under hydrated condition, enzymatic removal of PGs may result in better diffusion of monomers into the dentin tubules [33], as PGs control diffusion through the matrix and water displacement. The function of PGs on mature dentin deserves more attention.
Emerging Concepts and Future Prospects for Polymers in Dental Adhesion
It is estimated that resin composite restorations have a service life of 6–7 years, which is far less than half of the service life of dental amalgam. The main reason for replacement of direct adhesive resin composite restorations is secondary caries. Therefore failures at the interface has, to a great extent, inspired development of novel strategies to reduce degradation of the resin-tooth interface. Limitations within the material and intrinsic properties of the dentin have sparked a need to acknowledge the biology nature of the tissue as well as new directions in the resin chemistry that has been used in dental adhesive systems for the past 40 years. Some of the emerging and future concepts are detailed in the two sub-items below.
Material Perspective
Poor degree of conversion of dental resin monomers, elution of un-polymerized monomers and degradation of polymeric chains by enzymatic and hydrolytic challenges, have all been associated with the low stability of the resin at the bonded interface. Novel approaches have focused on improving the adhesion to the dental tissue as well as increasing stability of the resin and resin-dentin interface.
Resin monomers promoting chemical bond to enamel and dentin have been added to many contemporary adhesive systems in an attempt to achieve high bond strength via chemical bonding to the inorganic component of the tissue [34]. Specifically, formation of ionic bonds between 10-methacryloyloxydecyl dihydrogen phosphate monomer (10-MDP) and tissue hydroxyapatite crystals resulting in stable Ca-MDP salts [11]. Other functional monomers are also capable of chemical interaction with the tooth inorganic content. The functional monomers 4-methacryloxyethyl trimellitic acid (4-MET) and 2-methacryloxyethyl phenyl hydrogen phosphate (Phenyl-P) can also interact with hydroxyapatite crystal; however a more stable reaction is observed for Ca-MDP resulting salts [10, 11].
Aiming at establishing a polymeric chain that is less susceptible to degradation, a new class of monomers has been proposed. Silorane-based materials have an oxirane ring-opening mechanism of polymerization which is proposed to reduce polymerization shrinkage. In addition, siloxane molecules are more hydrophobic than methacrylate monomers, which may improve silorane resins` resistance to hydrolysis. However, the mechanical performance of silorane resins are not as predictable as compared to methacrylate resins [35], which may limits its application to areas of non-critical stresses. The substitution of TEGDMA by thiol-ene systems has been studied. Thiol-enes alone may not attain as good mechanical properties as methacrylate resins, but in association, the mechanical properties were equivalent. The methacrylate-thiol-ene resin systems showed increased methacrylate functional group conversion and decreased volumetric shrinkage [36, 37] and are promising alternative dental restorative materials.
Additional improvements on the degree of conversion of the adhesive systems were observed with new and less hydrophobic initiators of polymerization. Camphorquinone and aromatic amines are the most commonly used photoinitiator systems for light-activated dental resins, but they can be excessively hydrophobic making it difficult to activate the more hydrophilic monomers at the adhesive systems. The addition of alternative initiators such as 1-phenyl-1,2-propanedione (PPD), 2,4,6-trimethylbenzoyl-diphenylphosphine oxide (Lucerin-TPO) and bisacylphosphine oxide (BAPO) improve resin polymerization within hydrophilic domains and reduce susceptibility to inactivation by acidic adhesive monomer in self-etching systems. The use of diphenyliodonium hexafluorophosphate (DPIHP) in Bis-GMA and HEMA based experimental adhesives accelerates the resin polymerization and improve the mechanical properties [38].
Biological approaches to reduce degradation of the organic matrix resulted on the investigation of functional monomers to inhibit collagenolytic enzymes. An example is the incorporation of 12-methacryloyloxydodecylpyridinium bromide (MDPB), a polymerizable quaternary ammonium methacrylate [39], into self-etching resin adhesive blends. As for total-etch adhesive systems, an enzyme inhibitory effect can be achieved by adding a quaternary ammonium group, the benzalkonium chloride (BAC), to the etching solution. Studies using experimental materials showed possible incorporating MMP inhibitors in the methacrylate resin composition, aiming to a slow and continuous release of the inhibitors within the adhesive interface.
Tissue Perspective
As a major component of the bonded interface, the dental tissue components have a dramatic effect of the stability of resin-tooth interface. While there is still much to learn about the composition and role of organic components in different forms of dentin and enamel, there is a consensus that the stability of the dentin matrix remains key to the long-term strength and permeability of the interface.
The application of enzyme inhibitors to prevent dentin matrix degradation has been extensively investigated and few materials are already available to allow dental practitioners to rinse the surface with agents such as chlorhexidine (CHX). CHX is a potent antimicrobial agent and it can inhibit MMP-2, -9 and -8 by binding to the enzyme’s active sites. Similarly, it interacts with cysteine cathepsins, likely by interacting with the S2 subsites [40, 41]. It has been suggested that low concentrations of CHX (0.05–0.2 %) can inhibit the collagenolytic activity of dentin matrix, however the relative low substantivity of priming solutions may limit the long-term protective effect. Among other synthetic MMP inhibitors are the modified tetracycline. Special attention has been given to Galardin, a hydroxamate-based bisphosphonate, which inhibits MMPs by chelating its zinc active sites [31, 42]. This potential effect against MMP-1, -2, -3, -8 and -9 may reduce the bond strength loss overtime when compared to CHX. Because the inhibitory effect is mainly due to competitive binding of the inhibitory solutions with specific sites, the effectiveness is concentration dependent.
Remineralization of unprotected collagen at the dentin-resin interface has been proposed to preserve the adhesive bond strengths overtime. The biomimetic remineralization strategy is based on the use of polyanionic molecules such as polycarboxylic and polyphosphonic acids, which will be explained with greater detail below. The molecules mimic the mineral nucleation and growth control functions of endogenous non-collagenous proteins bound to collagen. In vitro intra-fibrillar and extra-fibrillar mineralization has been reported at the adhesive interfaces in presence of Portland cement (tricalcium silicate, dicalcium silicate, tricalcium aluminate, and a tetra-calcium aluminoferrite) mineralized using the biomimetic mineralization approach.
Another innovative approach is the biomodification of dentin matrix by multifunctional agents that increase the biomechanical properties and reduce the biodegradation rates of the dentin matrix [43]. Enhanced mechanical properties of biomodified dentin matrices are a result of the presence of non-enzymatic collagen cross-links induced by synthetic and nature-derived agents. These agents are also potent enzyme inhibitors and greatly decrease biodegradation of dentin in presence of host-derived enzymes as well as bacterial collagenase. Plant-derived oligomeric proanthocyanidins (OPACs), in particular, strongly interact with dentin collagen and also non-collagenous components such as PGs and endogenous proteases. Glutaraldehyde is another effective synthetic agent for collagen crosslinking, however due to its toxicity its clinical use is limited. 1-ethyl-3-[3-dimethylaminopropyl] carbodiimide hydrochloride associated with n-hydroxysuccinimide (EDC/NHS) has received much attention as a synthetic option with lower toxicity when compared to glutaraldehyde. Priming dentin with EDC/NHS shows increased long-term stability of the resin-bonded interfaces [44]. Riboflavin [45] has also been studied for this purpose, but the use of UV light may limit its use in clinical setting. Strategies to incorporate most of these agents into the restorative systems are ongoing.
Polymers in Restorative Composites Resins
First introduced over 50 years ago, polymer-based dental materials revolutionized restorative Dentistry primarily due to their outstanding esthetic and adhesive properties. These characteristics have allowed for substantially improved preservation of healthy tooth structures, prevention of postoperative sensitivity, reduction of microleakage, among other advantages compared to dental amalgams.
Over the years, resin based restorative materials have been the focus of a great deal of research, being drastically improved by manufactures, particularly with respect to aesthetic quality and mechanical behavior. Despite great improvements, failure and replacement of dental composite restorations continue to have great impact on clinical outcomes [46]. For instance, restorative composites still present a number of drawbacks, like wear, lack of a consistent degree of conversion, fracture and secondary caries [47, 48].
There have been several attempts to improve clinical performance of composite restorative materials by incorporating novel multifunctional monomers, developing different polymerization strategies or modifying filler components of the formulation. The following sections will explore some of the recent developments in restorative polymer composites.
Composition and Classification of Composite Resins
Composites used in Dentistry were developed in 1962 by combining dimethacrylates (epoxy resin and methacrylic acid) with silanized quartz powder [49]. Modern restorative composites are comprised of synthetic monomers, typically dimethacrylates, reinforcing fillers, typically made from radiopaque glass, quartz or silica, chemicals which promote or modify the polymerization reaction, and silane coupling agents which bond the reinforcing fillers to the polymer matrix [26].
The resin matrix of commercial dental composites has bis-GMA (bisphenol-A- glycidyldimethacrylate) as its predominant base monomer. Due to its high viscosity, bis-GMA is mixed with other dimethacrylates, such as TEGDMA, UDMA or other monomers of lower molecular weight [26, 50] to reduce viscosity. The monomers are heavily reinforced with filler particles, which add dimensional stability, improve wear and strength of the material, also reducing polymerization shrinkage [51].
A number of classification systems have been proposed to describe restorative composites. These materials may be distinguished by their consistency, and classified as flowable, conventional and packable [26]; but the most used classification system is based upon filler particle size. As restorative composites have evolved, the size of filler particles and their size distribution have been changed in an attempt to achieve the best possible mechanical properties while maintaining esthetics.
Initial formulations of dental composites (also known as macrofill) had average particle-sizes ranging from 10 to 50 µm. Clinically they were very resistant, but difficult to polish, also retaining poor surface smoothness overtime. Microfill composites generally present a wide range of size-distribution of silica particles (40–400 nm). At this size, filler loading represents a challenge for manufacturing composites with higher filler content due to agglomeration of the small particles in the matrix. These characteristics render microfilled composites highly polishable, but generally weak due to their relatively lower filler content and particle size.
One of most recent innovations in composite resins has been the development of nanofil composites, containing nanoscale particles ranging from 1 to 100 nm with a more homogenous size distribution. The increased filler content results in a lower amount of resin, which may significantly reduce polymerization shrinkage and improve the physical performance of nanocomposites [52]. Further details on the advantages of nanocomposites are presented in Sect. “Nanocomposites”.
The majority of resin composites in clinical use today are categorized in the general term of hybrid or micro–hybrid composites [41]. This broad category includes traditional hybrids, midifill, and minifill composites. The ‘‘hybrid’’ denomination implies a resin composite containing submicron inorganic filler particles and fine (over one micron) particles. Traditional hybrid resins consisted of a combination of 10–50 µm filler particles with amorphous spherical silica reinforcing particles of 40 nm. Midifill composites contain average particle sizes slightly greater than 1 µm, but also containing 40 nm-sized fumed silica microfillers. Minifill (also referred as mycrohybrid) materials present refinements in particle size, which generated restorative composites with sub-micron particles averaging from 0.4 to 1 µm. Most manufacturers have modified the formulation of their mycrohybrids to include more nanoparticles, and have named this category as nanohybrids [26]. The combination of various sizes of filler particles corresponds to an improvement in physical properties as well as acceptable levels of polishability [53].
Emerging Classes of Composite Resins
Anti-caries and Ion-Releasing Polymers
Due to the high frequency of recurrent caries after restorative treatments, much attention has been given to the therapeutic effects manifested by direct restorative materials. Restorative composites have demonstrated to accumulate more biofilm over time, when compared to enamel and other restorative materials, thus favoring the development of recurrent caries around these restorations [54]. Therefore, in an attempt to control or even prevent secondary caries, alternative clinical methods for caries prevention have been proposed including the search for new restorative materials with antibacterial activity.
Great emphasis has been given to the development of fluoride releasing materials, however, the direct antibacterial effect of dental materials is another important property as the inactivation of bacteria is a direct way to eradicate the cause of dental caries. Many attempts for developing dentin-bonding systems and restorative materials presenting antibacterial activity have been performed [55–62].
In the pursuit of developing composites with antibacterial activity, alterations to the resin matrix and filler components have been performed. Alterations of resin matrix constituents have included two relevant methods: firstly, the addition of soluble and immobilized antimicrobial agents in the resin matrix; secondly, the alteration of the filler components by addition of silver. Similarly, the immobilization of an antibacterial agent in a prepolymerized resin filler (PPRF) utilizing an antibacterial monomer has been previously reported [63].
Polymers with Soluble Antimicrobial Agents
The antibacterial effects of the restorative composites are relevant primarily in inhibiting plaque accumulation on the surfaces of the restorative material and tooth structures surrounding the restoration. Soluble antimicrobial agents added to the resin matrix, when exposed to a wet environment, have a tendency to be released from the restorative material, thus preventing plaque accumulation. Commonly, large amounts of these agents are released within a few days, followed by a dramatic decrease in concentration.
Chlorexidine has been the most frequently antibacterial agent incorporated into the resin matrix, and has demonstrated a strong antibacterial activity due to the release of antibacterial agents [64]. However, while a strong effect against bacteria has been obtained, the antibacterial activity drastically decreases over time, since large amounts of the agent are leached out within a few days [65]. Furthermore, it has been reported that the addition of chlorhexidine gluconate at a concentration of as low as 1 % resulted in significant a reduction of tensile and compressive strengths of restorative composite resins strengths [35].
Soluble fluoride agents have also been used to modify the resin matrix and obtain antibacterial properties in resin composites. Fluoride levels leached from composites are mostly much lower compared to levels released from conventional or resin-modified glass-ionomers [66]. Fluoride releasing resin composites might contribute to the decrease in cariogenic composition of dental biofilms if an appropriate amount of fluoride is released in the early stages of biofilm formation [67], yet challenges in developing composites with a sustained fluoride release remain.
Polymers with Immobilized Antimicrobial Agents
The immobilization of antibacterial components in the resin matrix has been another attempt to modify resin components to render restorative materials caries-resistant. This approach is used to obtain antibacterial composites that do not release any antibacterial component. Rather, the immobilized agent acts as a contact inhibitor against bacteria attaching the material surface [32].
To that end, quaternary ammonium dimethacrylate monomers, such as 12-methacryloyloxydodecylpyridinium bromide (MDPB), were copolymerized with resins to yield antibacterial activity. MDPB was developed by combining a quaternary ammonium—which presents a wide spectrum of antibacterial activity—and a methacryloyl group, incorporated into the composite matrix. This agent copolymerizes with other monomers in the composite and thus, the antibacterial component is covalently linked to the polymeric network [32]. The immobilized agent does not leach out of the composite but functions as a contact inhibitor against bacteria attaching to the surface, therefore its effect is not able to reach the tooth structures surrounding the restoration [68]. In summary, the effects of the MDPB-containing composites are not so intensive as the materials that release antibacterial agents. Its effect is mainly bacteriostatic, as the agent cannot penetrate through the cell wall or membrane unlike free antibacterial agents described above [32].
In order to improve the antibacterial activity of these systems, the addition of antibacterial monomers in prepolymerized resin fillers (PPRF) have also been reported. Using this method, the PPRF can be highly cured and washed before they are loaded into the composite, thus ensuring greater immobilization of the antibacterial components than when the antibacterial agent is added to the monomer phase. In an attempt to increase the concentration of MDPB in resin composites, the antibacterial monomer was utilized as a PPRF. The incorporation of MDPB to the composite as a PPRF, for instance, has been shown to allow for an increase of MDPB concentration in the order of 10 times, thus promoting more reliable inhibitory effects on plaque accumulation [69]. An experimental composite prepared by the addition of PPRF-MDPB to a commercially available composite demonstrated to inhibit the progression of artificially induced secondary root caries lesions regardless of adhesive system [70]. The satisfactory results found with MDPB led to the incorporation of quaternary ammonium compounds (QAS) into restorative composites. In recent years, many attempts to incorporate QAS into polymer based restorative materials have been performed, demonstrating good results in antibacterial activity [13, 19, 71, 72]. Therefore, it may be expected that future composites will present relevant antibacterial properties and that this will be a subject of intensive research in future years.
Antimicrobial Fillers
Similar to the modifications described above, alterations to the filler components have been conducted in order to achieve antibacterial composites. Numerous studies have evaluated composites modified by silver-functionalized filler particles. For dental composites, in particular, the use of silver-zeolite, silver-apatite, and silver-supported zirconium phosphate has been reported [46]. Silver-zeolite and silver-apatite show antibacterial effects which are dependent upon the slow release of silver ions, and these effects are expected to last for longer periods of time when compared to materials with embedded antibacterial components. Composites containing silver nanoparticles have demonstrated inhibited biofilm formation and reduction of biofilm viability [73]. However, poor color stability is a common problem for these types of restorative composites.
Low-Shrinkage Composite Resins
Ring-Opening Monomers
One of the main drawbacks in dental composites remains the high polymerization shrinkage of these materials. It is well recognized that the polymerization stresses resulting from polymerization shrinkage of composite restorations can lead to numerous adverse clinical effects, including de-bonding, post-operative sensitivity, marginal discrepancies, among other clinically relevant issues [26]. The extent of shrinkage is generally influenced by the volume of resin, its composition, and the degree of conversion of the cured monomers [41]. Current commercial dental composites have a volumetric shrinkage ranging from 1.6 to 8 vol % [74]. Therefore, the development of non- or minimal-shrinkage dental composites has been the focus of extensive research. Investigators have made several attempts to reduce shrinkage by introducing monomer molecules that present different polymerization strategies to more common linear chain lengthening, such ring-opening monomers like spiro-orthocarbonates [75], epoxy-base resins like the siloranes [76], as well as high-molecular-weight monomers like dimer acid-based dimethacrylates [77], tricyclodecane (TCD) urethane and organically-modified ceramics (ormocers) [78, 79]. Low shrinkage oxiranes, for instance, are cyclic ethers that polymerize through a cationic ring-opening mechanism, in contrast to the free radical polymerization of methacrylates [54, 80]. Oxirane based-resins have shown many advantageous properties, such as improved depth of cure, lower polymerization shrinkage, higher strength, as well as comparable hardness and acceptable glass transition temperature when compared with conventional bis-GMA-based dental composites [81]. However, residual monomers released from oxirane-based composites after polymerization have shown relevant toxicity [82].
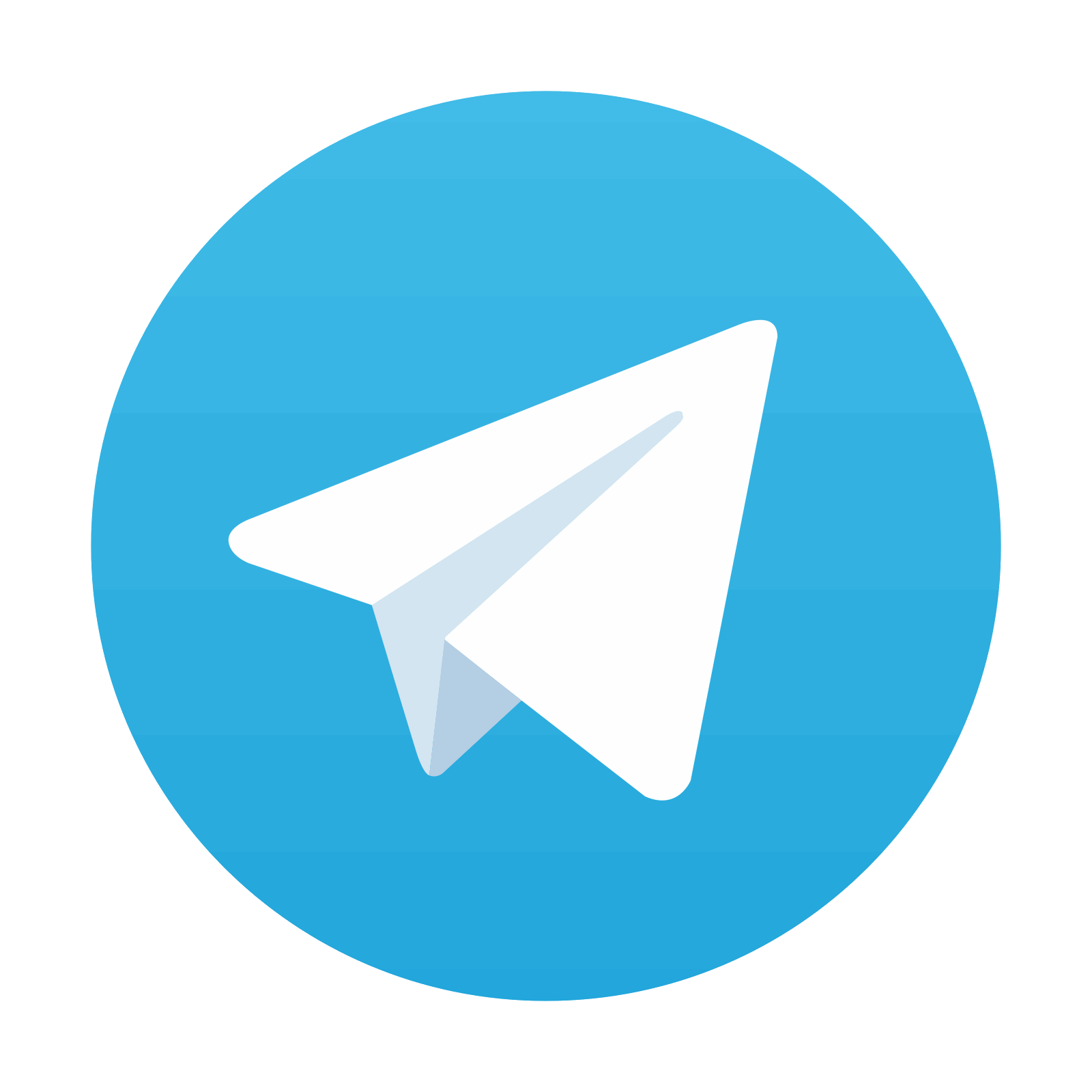
Stay updated, free articles. Join our Telegram channel

Full access? Get Clinical Tree
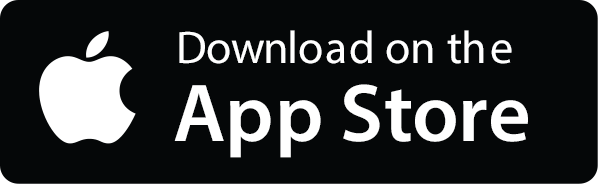
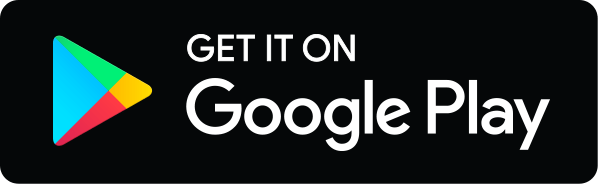
