© Springer Science+Business Media New York 2014
Vangelis Sakkalis (ed.)Modern Electroencephalographic Assessment TechniquesNeuromethods9110.1007/7657_2014_73Clinical Electroencephalography in the Diagnosis and Management of Epilepsy
(1)
Cleveland Clinic Epilepsy Center, 9500 Euclid Avenue, Desk S-51, Cleveland, OH 44195, USA
Abstract
This chapter deals with the clinical electroencephalography (EEG) and its application on epilepsy. EEG, its origin, its technical aspects comprising also the electrode placement, and the related montages are addressed. Then, the clinical applications of EEG and mainly its use in epilepsy are reviewed, while cardinal EEG findings in interictal and ictal recordings, such as the epileptiform discharges and the ictal patterns in seizures, respectively, are mentioned. The rest of the chapter deals with the epilepsies, their classification as focal or generalized with their sub-divisions, and the related clinical manifestation. Then, EEG and epilepsy in specific populations, such as neonates and infants, are addressed, and finally, main pitfalls in the interpretation of EEG are also reviewed.
Key words
Electroencephalography (EEG)Technical aspectsClinical applicationEEG in epilepsyThe epilepsies1 Introduction
Electroencephalography (EEG) constitutes the single most important and frequently performed neurodiagnostic study in patients with seizures and related paroxysmal events. It is essential to state at the outset that epilepsy remains a clinical diagnosis, and therefore the results of any EEG study need to be carefully examined individually and under the prism of each patient’s clinical presentation. The descriptions of the seizure by witnesses (observer-report) when available, and by the patient (self-report) when possible, are key elements of the diagnostic evaluation. Preictal signs and behaviors, presence of aura, and ictal and postictal signs and symptoms are cardinal functional features. These features can be further elucidated with additional laboratory investigations, the majority of which rely on the use of EEG, e.g., outpatient or ambulatory EEG recordings and inpatient continuous bedside EEG or video-EEG monitoring. Despite tremendous progress in structural and functional neuroimaging techniques and improved spatial and temporal resolution of functional neurodiagnostic studies, EEG remains the paramount tool to assess ictal and interictal electrical brain signals.
2 History
Hans Berger (1873–1941), a professor of psychiatry in Jena, was the first to record the human EEG in 1924. His discovery that the brain develops a low-level subaudio-frequency electrical activity gave birth to a new neurophysiological specialty [1]. Berger coined the term “electroencephalography” and described for the first time the alpha wave (occipital dominant) background rhythm and its relationship to eye closure. Prior to this discovery quantification or description of the ongoing brain activity had not been possible. Later in the 1930s, Gibbs, Lennox, and Jasper defined the patterns of generalized spike-and-wave activity and focal spikes or sharp waves as markers of epilepsy [2].
As time went by the techniques of EEG recording became more sophisticated with the addition of more channels and emergence of the first ambulatory EEG systems in the 1970s [3]. The development of video technology allowed for the patient’s peri-ictal behavior to be captured and time-locked with the EEG and facilitated the creation and steady increase of long-term video-EEG monitoring units. Multichannel intracranial long-term EEG recordings appeared together with the advancement of the epilepsy surgery.
3 Technical Aspects: Electrode Placement
Although there is no single best method for recording EEGs under all circumstances, the various guidelines and consensus statements that have been published and revised over the years [4, 5] have largely been adopted by most EEG laboratories. The current guideline of the American Clinical Neurophysiology Society [5] on the minimal technical requirements for performing clinical scalp EEG recordings suggests that 16 electrodes (rather than only 8 electrodes as had been stated in the previously published guidelines [6]) are required at a minimum to adequately analyze the areas producing most of the normal and abnormal EEG patterns [5]. Additional electrodes (including closely spaced “10–10” electrodes) are often needed for monitoring other physiologic activities and resolving subtle epileptiform patterns.
Appropriate alternating current (AC) wiring and adequate grounding of all EEG equipment to a common point are mandatory. For standard recordings, the low-cut frequency filter should be no higher than 1 Hz (corresponding to a time constant of at least 0.16 s), while the high-cut filter must be at least at 70 Hz. It is important to emphasize that a setting lower than 70 Hz for the high-cut frequency filters can distort or attenuate spikes and other pathologic discharges into unrecognizable forms and can cause muscle artifact to resemble spikes.
Occasionally a 60 Hz filter (also called notch filter) is used when other measures to eliminate 60 Hz interference have failed, but this can also distort or attenuate important EEG features such as spikes. For many years EEGs were obtained with analog recordings that were then charted on paper. The available amplifiers and the frequency response of the EEG pens hampered our ability to record higher frequency activity [7]. With the advent of digital recordings we are now able to display and explore much higher frequencies, when the record has been digitized at a high enough rates (at least twice as high as the frequency of interest).
Electrode impedances should not exceed 5 kΩ and should be checked as a routine prerecording procedure and rechecked during the recording, whenever there is concern that an artifactual pattern has appeared as the study progresses. Appropriate calibrations should be made at the beginning and end of every EEG recording. The calibration procedure is an integral part of every EEG recording, provides a scaling factor for the interpreter, and tests the EEG machine for sensitivity, high- and low-frequency response, and noise level among other parameters. The sensitivity of the EEG for routine recordings must be within 5–10 μV/mm of pen deflection, while for young children sensitivity is usually decreased to 10–15 μV/mm due to the higher voltage of EEG activity in this age [8]. Sensitivity is defined as the ratio of input voltage to pen deflection. It is expressed in microvolts per millimeter (μV/mm). A commonly used sensitivity is 7 μV/mm, which, for a calibration signal of 50 μV, results in a deflection of 7.1 mm.
For routine recordings, a paper speed of 3 cm/s or digital display of 10 s per page is usually utilized in the US. A lower paper speed of 1.5 cm/s or more condensed digital display of 20 s/page is sometimes used for EEG recordings in newborns or in other special situations. Silver–silver chloride or gold disk electrodes held on by collodion are mostly recommended, but other electrode materials and pastes may be used effectively especially with contemporary amplifiers which allow for high input impedances.
Routine EEG consists of at least 20 min of technically satisfactory recording. In the usual 20-min recording specific tasks should be included. Thus, it must be ensured that the recording contains periods of eye opening/eye closure. Moreover, activation procedures, which might elicit the appearance of pathologic electro-clinical features (such as spikes or ictal patterns), should be routinely performed save for medical or other contraindications. These maneuvers include hyperventilation, which is performed for a minimum of 3 min, and intermittent photic stimulation. Sleep deprivation prior to the recording is another activation technique that increases the probability of recording the states of drowsiness and/or sleep, which are critical segments of time especially in patients with suspected or known seizure disorders [6].
It is the responsibility of the EEG technologist to specify on the EEG recording the patient’s level of consciousness (awake, drowsy, sleeping, lethargic, stuporous, or comatose) and any related changes. In patients with depressed level of consciousness or those showing invariant EEG patterns of any kind the technologist should systematically apply visual, auditory, and somatosensory stimuli and document the patient’s responses [5]. Careful observation of the patient with frequent notations is essential particularly when unusual waveforms are observed in the tracing or paroxysmal behavioral changes occur during the period of the recording. Every effort should be made to obtain a technically satisfactory record. Production of a clinical EEG record with lost or inaccurate information due to technical oversights is poor medical practice.
The 10-20 system or International 10-20 system is an internationally recognized method that describes the application and location of EEG electrodes on the scalp. This largely accepted method of electrode placements was proposed by Jasper in 1958 to ensure standardized reproducibility within and between subjects [9]. The designation of each electrode takes into account the underlying area of cerebral cortex that is covered by the particular electrode. Odd or even numbers (i.e., F3, F4) refer to left and right hemisphere, respectively. The measurement technique is based on standard landmarks on the skull (namely, the nasion, inion and the left and right preauricular points) and allows for measurements to be proportional to the size and shape of the skull. The numbers “10” and “20” refer to the fact that the actual distances between adjacent electrodes are either 10 or 20 % of the total front-to-back (nasion to inion) or right-to-left distances of the skull [10].
The first measurement is in the anterior-posterior plane through the vertex (taken from the nasion to the inion). This measurement divides the skull from anterior to posterior into five separate regions, expressed as Fp, F, C, P, and O with the letters representing the expected location of the underlying frontopolar, frontal, central, parietal, and occipital areas. The first mark (Fp) is placed above the nasion at 10 % of the nasion–inion distance. The second (F), third (C), and fourth (P) marks—corresponding to the position of the three midline electrodes Fz, Cz, and Pz, respectively—are placed at 20 % intervals of the total nasion–inion distance. Accordingly, the midline O mark would be located above the inion at 10 % of the total distance.
Regarding the lateral measurements across the central coronal plan, 10 % of the entire distance is taken for the temporal (T) point (positions of T7 and T8 electrodes) up from the preauricular point on either side. The corresponding left and right central points (C3 and C4 electrodes) are then marked 20 % of the distance above the temporal points. As described above, the center of central-coronal line corresponds to the C vertex (Cz) location. The anterior-posterior line of electrodes over the temporal lobe, frontal to occipital, is assessed by measuring the distance between the Fp midline point through the T position of the central-coronal line and back to the mid-occipital spot. The left and right Fp electrode position (Fp1 and Fp2) is marked 10 % of the distance from the midline in front, while the occipital electrode position (O1 and O2, respectively) is marked 10 % of the distance from the midline (O position) in the back. The inferior frontal (F7 and F8) and posterior temporal (P7 and P8) positions then fall 20 % of the distance from the left and right Fp and occipital electrodes, respectively. Finally, the midfrontal and midparietal electrodes (F3, F4 and P3, P4, respectively) are placed along the frontal and parietal coronal lines, respectively, equidistant between the temporal and the midline. The 10-20 system is the only one recommended by the International Federation of Clinical Neurophysiology (IFCN). All 21 electrodes and placements of the 10-20 system as recommended by IFCN should be used [9, 11].
EEG montages may vary among laboratories. It is very desirable for all EEG laboratories to use at least some comparable montages and include the classical montages (longitudinal bipolar, transverse bipolar, and a referential montage, for example an ipsilateral ear reference montage) to facilitate communication and comparisons. The ACNS recommends that EEG montages should be designed in conformity with Guideline 6: A Proposal for Standard Montages to Be Used in Clinical Electroencephalography [12]. Both bipolar and referential montages should be used. In general the longitudinal bipolar (LB or “double banana”) and the referential (R) montages consist of a series of leads grouped in anatomical proximity and extending sequentially across the head from the left to the right side. In this fashion hemispheric differences can be readily appreciated and blocks of homologous derivations involving homologous brain regions can be compared.
4 Clinical Applications
The main indication for performing an EEG is clinical suspicion of epilepsy, although a single routine EEG study may be normal in up to 50 % of individuals with epilepsy [4, 13]. Repeat or prolonged EEG studies with the use of appropriate activation maneuvers (such as hyperventilation, sleep deprivation, and photic stimulation) may yield epileptiform abnormalities in up to 80–90 % of individuals with epilepsy [7, 14]. Therefore, a normal EEG study by itself can never be used in isolation to rule out the diagnosis of epilepsy.
The purpose of EEG recordings is to detect interictal epileptiform abnormalities, which include spikes and sharp waves. When such abnormalities are present, the EEG can help regionalize the brain areas that are involved to produce the observed interictal and/or ictal patterns on scalp electrodes. The ability of the scalp EEG to detect interictal epileptic discharges depends on the extent of the irritative zone, the location and proximity of the generator in relation to the scalp, and the orientation of the dipole [15]. In the presence of epileptiform activity, the EEG recording can also help determine the type of seizure or epilepsy syndrome. The two main EEG hallmarks of epilepsy are the focal interictal spike or sharp wave in focal epilepsies and the bifrontocentral spike–wave discharges in the generalized epilepsies.
EEGs may also be used in the assessment of acute or chronic encephalopathies (e.g., secondary to metabolic derangements, infectious conditions, or degenerative disorders) and focal brain lesions (such as cerebral infarction, hemorrhage, neoplasms). In pediatrics the EEG is useful in assessing the level of maturation of the brain. Lastly, the only clear indication for use of the EEG as a tool to monitor the effect of treatment with antiepileptic drugs is in patients with absence epilepsy [4].
Quantitative electroencephalography (qEEG) allows for a compressed view of several hours of EEG and has been used by some investigators to enhance the clinical diagnosis and treatment planning provided to individuals with mild traumatic brain injury and postconcussive symptoms [16]. Finally continuous bedside EEG monitoring and qEEG in the intensive care unit (ICU) setting have been shown to significantly increase the detection of nonconvulsive seizures (NCS) and nonconvulsive status epilepticus (NCSE) in critically ill patients, who typically have subtle if any clinical manifestations [17].
Fast sampling rates (up to 2,000 Hz or higher) and wideband intracranial EEG recordings have allowed investigators to explore high-frequency oscillations (HFOs) in the 100–600 Hz frequency range, which are more clearly defined on intracranial recordings but have also been detected with scalp electrodes. Some investigators have reported that HFOs represent a promising neurophysiological biomarker of epileptogenic foci [18], but their clinical significance is not well established at this time, and their discussion is beyond the scope of this chapter.
5 The EEG in Epilepsy
5.1 Interictal Recording: Epileptiform Discharges
Interictal epileptiform discharges (IEDs)—which include spikes and sharp waves (SWs)—are extremely useful in the diagnosis of epilepsy and in the classification of the epileptic condition, because they occur much more frequently than clinical seizures during routine EEG recordings. In the appropriate clinical setting and depending on the patient’s age and type of epilepsy syndrome, up to 98 % of patients whose EEG demonstrates clear epileptiform discharges may have a history of epilepsy [19]. Moreover, by defining the “irritative zone” (the area of cortical hyperexcitability responsible for the generation of localized epileptiform activity during the interictal state) these epileptiform abnormalities can be extremely helpful in regionalizing the epileptogenic focus, which is a key step in patients with medically intractable focal epilepsy who are potential candidates for epilepsy surgery [20, 21]. Thus, by combining the patient’s clinical and semeiological history with the observed IEDs the clinician is often able to correctly identify and classify the electroclinical syndrome [21]. Electroclinical correlations are essential. It is important, for example, to differentiate IEDs in the setting of benign rolandic epilepsy from IEDs that involve a similar brain region but occur in a different subset of patients who may manifest difficult-to-control focal motor seizures. This differentiation has clinical implications, which may include considerations for or against resective epilepsy surgery.
The presence or the absence of IEDs is influenced by the duration of the EEG recording and the need to sample EEG activities at different periods of time. Although a single routine EEG study in a person with epilepsy may only capture IEDs in 50 % of cases, the yield increases with repeat EEG recordings performed at different times and plateaus around 92 % by the fourth routine EEG study [14]. Similarly, recordings performed in epilepsy monitoring units (EMU) increase the probability of capturing IEDs over a period of several days although approximately 10–19 % of patients with epilepsy do not demonstrate IEDs on scalp EEG regardless of the number and duration of recordings [13]. The frequency of IEDs can vary for many reasons. Their frequency may increase near the seizure onset [22, 23], and/or after a seizure [24, 25], during NREM sleep [26], or following withdrawal of antiepileptic medications [25, 27] and activation maneuvers (i.e., hyperventilation). Lastly, IEDs may (rarely) be observed in people with no seizure history. Even though the presence of IEDs may be an incidental finding an increased epileptogenic tendency cannot be excluded, and avoidance of known seizure precipitants may be advised in some cases (such as individuals with abnormal photoparoxysmal responses during intermittent photic stimulation).
Typical spikes and sharp waves should be easily distinguishable from the background EEG activity [19, 28]. However, the experienced electroencephalographer should also be familiar with numerous “sharp transients” such as vertex or lambda waves which are features of normal EEG studies or wicket spikes and other variants of benign nature that should not be confused with epileptiform patterns [19]. Spikes are epileptiform discharges of 40–80 ms of duration, while sharp waves have a duration of 80–200 ms (both are measured at the midline between the baseline and peak of the spike or the sharp wave). As a rule the main component of epileptiform discharges has a negative polarity of scalp EEG. There is no difference between spikes and sharp waves with regard to the diagnostic value of these abnormalities [19, 28]. Both are sharply contoured transients that stand out from the background (their amplitude is usually at least 30 % higher than the amplitude of the background EEG) and are usually followed by a slow wave.
Spike and SW discharges are more commonly focal in terms of distribution but can also be generalized and appear either as independent waveforms or as components of complexes that encompass various different waves [20]. The background often shows slowing in the same regions that are implicated by the epileptiform discharges or may have periods of slowing with a more diffuse distribution. Focal and/or diffuse slowing is seen mostly in patients with focal or symptomatic generalized epilepsies, while epileptiform discharges in idiopathic generalized epilepsy are usually accompanied by an otherwise normal background activity [29]. The typical epileptiform discharges of IGE are bisynchronous, symmetrical, generalized, and fairly regular spike–wave discharges, polyspikes, and polyspike–wave complexes [30]. Other abnormalities such as periodic lateralized epileptiform discharges have important clinical implications and may be seen in the setting of acute or subacute structural lesions, such as stroke, necrotizing focal encephalitis, and rapidly growing neoplasms, or may represent a transient postictal finding following a seizure in some patients with focal epilepsy [28].
5.2 Ictal Recording: Ictal Patterns
Focal and generalized seizures usually manifest with distinctly different ictal patterns. In contrast to the paroxysmal epileptiform discharges occurring during the interictal state, ictal patterns are characterized by an EEG onset, a distinct evolution, and an end (EEG offset). The EEG onset in focal epilepsies may be nonspecific manifesting with either focal or generalized desynchronization, low-voltage fast activity, or irregular focal or bilateral delta activity. The evolution of the seizure is often the clearest part of the ictal EEG, which may demonstrate progressive changes from lower amplitude, faster activity to activities of higher amplitude, slower frequencies, and wider distribution. Postictal slowing may be seen at the end of the electrographic seizure marking the end of the ictal event and the transition to the postictal period. Postictal slowing, when present, is definitely less rhythmic than the ictal pattern itself.
Some simple partial seizures, such as auras or focal motor seizures, may be accompanied by subtle EEG changes without clear evolution of amplitude or frequency. Importantly, up to 70 % of simple partial seizures may not be associated with a definite EEG correlate. Therefore, more often than not, focal seizures that only activate a small or a deep area of cortex have no EEG signature on scalp recordings [7]. It is critical to understand that the absence of scalp EEG changes during such paroxysmal events cannot rule out an epileptic etiology for these events. On the other hand, a clear ictal pattern is always present in secondarily generalized tonic-clonic (GTC) seizures. In cases characterized by rapid spread of the ictal discharge, a focal onset may not be readily apparent. However, significant postictal slowing may be seen in some of these cases following secondary generalization. The EEG pattern itself during the period of a secondarily GTC seizure is similar to that seen with GTC seizures in primary generalized epilepsy. The usual pattern is rapid low-amplitude spiking evolving to a slower spike–slow wave discharges. These patterns correlate with the observed tonic and clonic activity, respectively [29, 31].
Other distinct ictal EEG patterns may be observed in the generalized epilepsies. Generalized myoclonic seizures are associated with a high-amplitude, generalized, polyspike activity with frontocentral accentuation occurring in isolation or repeating itself at a frequency of 10–16 Hz. Ictal discharges are sometimes preceded by irregular 2–5 Hz generalized spike–wave complexes and are followed by irregular 1–3 Hz slow waves. In myoclonic-astatic seizures, simultaneous myoclonic jerks of the flexor and extensor muscles of the body are associated with fast (>2.5–3 Hz), generalized, polyspike–wave discharges or spike–wave complexes. Typical absence seizures exhibit a characteristic monomorphic ictal rhythm of abrupt onset and offset, which consists of bilateral, symmetrical, and synchronous 3 Hz spike–wave discharges on a normal background. In epilepsy with myoclonic absences the ictal EEG shows regular, rhythmic, bisynchronous, 3 Hz spike–wave discharges, which are also commonly associated with an abrupt onset and end. Admixed polyspikes may also be seen occasionally during the ictal discharge in these particular seizures [30, 32].
6 The Epilepsies
6.1 Focal Epilepsies
The definition of focal seizures according to International League Against Epilepsy (ILAE) is “a seizure whose initial semeiology indicates, or is consistent with, initial activation of only part of one cerebral hemisphere” [33]. The ictal symptomatology and the EEG features vary among the different locations of focal seizure onset.
Mesial Temporal Epilepsy
The temporal lobe is the most epileptogenic region of the human brain. Within the temporal lobe the three-layered archicortex of the hippocampal formation is the central component of the highly epileptogenic electrophysiologic network that includes the amygdalohippocampal complex as well as the subiculum and entorhinal cortex [34]. The most common cause of mesial temporal epilepsy (mTLE) is hippocampal sclerosis (HS) which is oftentimes associated with an initial precipitating injury, such as complex febrile seizures, perinatal insults, head trauma, and infection of the central nervous system. Not infrequently HS is a bilateral disease [35], with bilateral hippocampal neuropathological abnormalities present in autopsy tissue from patients with a clinical diagnosis of temporal lobe seizures [36].
Clinically, the most frequent seizures in mTLE are the so-called complex partial seizures with or without automatisms. 80 % of patients report auras or a history of auras, which are mainly associated with experiential (i.e., deja vu, fear, anxiety) and viscerosensory symptoms (i.e., nausea, or indescribable rising sensation from the epigastrium) [37]. Prolonged seizures and status epilepticus are uncommon in most patients with mTLE.
Typical complex partial seizures are characterized by a fixed motionless stare and limited if any motor manifestations with the exception of oroalimentary or distal manual automatisms. These seizures are commonly accompanied by autonomic manifestations such as tachycardia, hyperventilation, and piloerection. Lateralizing ictal signs include contralateral hand dystonia, which is usually accompanied by ipsilateral automatisms. Ictal speech, ictal emesis, and automatisms with preserved awareness suggest nondominant lateralization, whereas postictal aphasia implicates the dominant temporal lobe. Prominent motor phenomena such as tonic manifestations or contralateral clonic jerking and contralateral postictal paresis (Todd’s phenomenon) suggest propagation outside the mesial temporal lobe to involve the ipsilateral motor neocortex [37, 38].
More than 90 % of patients with mTLE exhibit anterior temporal spikes with maximal amplitude on scalp EEG over the F7, F8 electrodes (in the standard 10-20 system described earlier in this chapter) or over additional anterior temporal surface electrodes [such as the commonly added FT9, FT10 electrodes, which are placed in the FT position (between the F and T positions) and inferior—by 10 % of the measured distance—to the standard infrasylvian chain of temporal electrodes, i.e., below the FT7 and FT8 marks] and over the so-called basal temporal electrodes, which may be utilized during video-EEG monitoring in some centers [such as sphenoidal (Sp1 and Sp2) or less commonly oropharyngeal electrodes] [31].
The sphenoidal electrode consists of a flexible wire with an uninsulated tip and is placed near the sphenoidal wing over a cannula that is first inserted between the zygoma and mandibular notch traversing the temporal and masseter muscles. The guiding cannula is removed after advancing the wire to a depth of 4–5 cm. This is a simple bedside procedure, although some authors favor precise placement under fluoroscopic guidance. Sphenoidal electrodes are mainly of value for distinguishing between mesial and lateral temporal epileptogenic sources. Whether sphenoidal electrodes should be used in the presurgical evaluation of selected patients with medically intractable focal epilepsy remains a matter of debate [39, 40].
Anterior temporal sharp waves may be seen bilaterally in approximately one-third of patients with unilateral mTLE particularly during sleep. Strongly lateralized (>90 %) or exclusively unilateral anterior temporal IEDs are predictive of the side of seizure onset in patients with unilateral mTLE. IEDs that are maximum over the mid-temporal (T7 and T8) electrodes may be seen in some patients with documented mTLE, but such finding may also signify the presence of an extramesial temporal generator or a more extensive irritative zone. Subtle or no definite EEG changes may be seen during auras or at the time of the initial behavioral change. Therefore, the clinical onset of the seizure precedes the EEG onset in these patients. Ictal (as well as interictal) activities arising from the mesial temporal lobe will not produce visible changes on scalp EEG, if they remain confined to the mesial temporal structures, unless or until the epileptiform activities have also recruited the inferolateral temporal neocortex. As the seizure evolves, a regional anterior temporal or lateralized ictal pattern, which consists of rhythmical theta or alpha activity (typically 5–9 Hz), is typically seen within 10–40 s after clinical onset in up to 80 % of patients with mTLE [41]. If present this activity, which is considered to be a hallmark of mTLE, correctly lateralizes the seizure focus in up to 95 % of patients [42].
The postictal record should be examined for the presence of lateralized focal postictal slowing that involves the temporal region. Postictal slowing may be seen in approximately 70 % of seizures and has strong lateralizing value offering correct lateralization in up to 90 % of cases [31, 42]. The probability of a favorable surgical outcome following anterior temporal lobectomy is highest in patients, who exhibit exclusively unilateral anterior temporal IEDs combined with scalp ictal EEGs that remain regionalized without contralateral propagation.
In patients with suspected pharmacoresistant mTLE, in whom the scalp EEG provides discordant information and/or non-localizable ictal patterns, further evaluation with intracranial recordings (preferably using depth electrodes) may be necessary. As expected, intracranial electrodes detect a higher number of localized discharges and focal hypersynchronous activities as compared to scalp EEG [43]. In fact, most patients with mTLE (>60 %) respond to antiepileptic medications and enjoy long periods of sustained seizure freedom. However, a significant number of patients, and in particular the majority of patients (up to 60–90 % in some studies) with the substrate of HS, continue to experience recurrent seizures despite multiple trials of antiepileptic medications at high doses.
Mesial TLE is the prototype surgically remediable epilepsy syndrome. Therefore, it is imperative for these patients to be carefully identified and referred to a comprehensive epilepsy center early on in the course of the intractability. Standard anterior temporal lobectomy (ATL) is the most common surgical procedure performed in approximately two-thirds of patients with medically intractable focal epilepsies [42, 43]. Indisputable class I evidence supports the effectiveness and superiority of ATL, as compared to continued medical therapy, in patients with intractable mTLE [44].
Neocortical Temporal Lobe Epilepsy
According to the current epilepsy classification the entity of neocortical temporal lobe epilepsy (nTLE), together with that of mTLE, is classified under the diagnosis of TLE. nTLE compared to mTLE is a rather heterogeneous entity as far as the etiologies and the electroclinical features are concerned. nTLE is clearly less common and usually presents later in life. As compared to mTLE, individuals with nTLE are usually older by an average of 5–10 years when they first start having seizures. The causes of nTLE are variable and include the so-called long-term epilepsy-associated tumors such as gangliogliomas and dysembryoplastic neuroepithelial tumors along with vascular malformations (mainly caveromas), focal cortical dysplasia, and trauma.
Motionless staring and unresponsiveness may represent the first objective clinical manifestations in nTLE and are often followed by contralateral clonic jerks. Patients with nTLE are more likely to report auditory, vertiginous, or experiential auras. Other clinical symptoms suggestive of nTLE include cephalic/indescribable sensations, ictal speech and vocalizations, whole-body movements, and rapid onset of version. nTLE seizures are generally shorter in duration. Moreover, evolution to secondarily GTC seizures is seen more frequently in nTLE as compared to mTLE patients [45–47].
Regarding scalp EEG features, the presence of interictal rhythmic slowing over one temporal region may raise the question of nTLE. Ipsilateral interictal epileptiform abnormalities are seen in up to 80–90 % of nTLE patients [48, 49]. However, there is little evidence to support the use of interictal EEG alone in differentiating nTLE from mTLE. The ictal pattern in NTLE usually consists of an irregular, polymorphic delta slowing with lateralized rather than regionalized (temporal) distribution at seizure onset [46]. This can be preceded by repetitive spiking or followed by a more rhythmic theta or alpha activity [50].
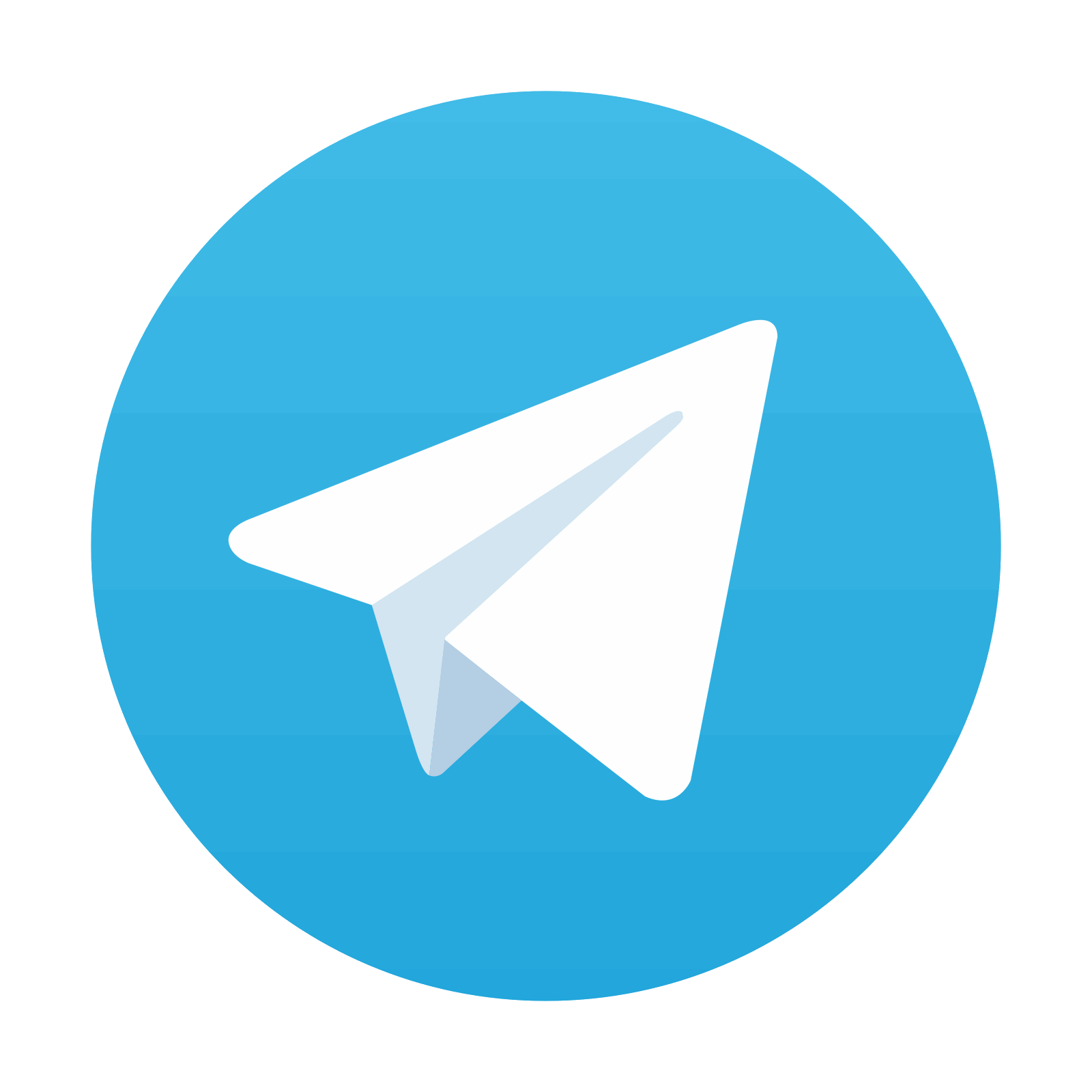
Stay updated, free articles. Join our Telegram channel

Full access? Get Clinical Tree
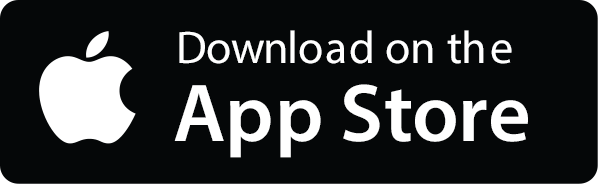
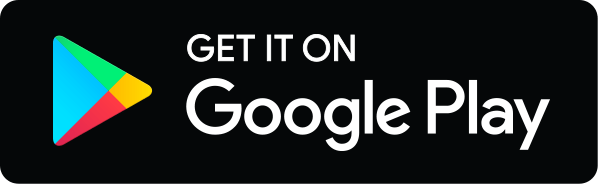
