24 Dyslipidaemia
Epidemiology
The ideal serum lipid profile is unknown and varies between different populations, even across Europe, and also within a given population. For practical purposes the values presented in Table 24.1 represent the target levels for TC and LDL-C in the UK for adults receiving treatment for secondary prevention of CVD. For completeness, the values for triglycerides and HDL-C are also presented, although the benefit of achieving the stated targets is less clear.
Table 24.1 Optimal serum lipid profile
Total cholesterol (TC)a | <4.0 mmol/L |
LDL cholesterol (LDL-C)a | <2.0 mmol/L |
Triglyceridesb | <1.7 mmol/L |
HDL cholesterol (HDL-C) | >1.0 mmol/L in men |
>1.2 mmol/L in women |
a Target levels in individuals with established atherosclerotic disease, coronary heart disease, stroke, peripheral arterial disease, diabetes mellitus or where there is a cardiovascular disease risk >20% over 10 years. In these identified individuals, the aim is to achieve the value stated in the table or a 25% reduction in total cholesterol and a 30% reduction in LDL-C from their baseline levels should these set target levels lower than those stated in the table.
Despite a 50% reduction in the death rate from CVD over the past 25 years, it remains the leading cause of premature death and morbidity in the UK (British Heart Foundation, 2008), and the higher the levels of TC in an individual the greater the chance of developing CVD. At the individual level there appears no level below which a further reduction of TC or LDL-C is not associated with a lower risk of CVD. The death rate from CVD is threefold higher in males than females, but because women live longer and are at increased risk of stroke after the age of 75 years their lifetime risk of disease is greater (National Institute of Health and Clinical Excellence, 2008a).
Lipid transport and lipoprotein metabolism
The protein components of lipoproteins are known as apoproteins (apo), of which apoproteins A-I, E, C and B are perhaps the most important. Apoprotein B exists in two forms: B-48, which is present in chylomicrons and associated with the transport of ingested lipids, and B-100, which is found in endogenously secreted VLDL-C and associated with the transport of lipids from the liver (Fig. 24.1).
Reverse cholesterol transport pathway
The reverse cholesterol transport pathway (Fig. 24.2) controls the formation, conversion, transformation and degradation of HDL-C and is the target site for a number of new, novel drugs and has recently been described (Chapman et al., 2010).
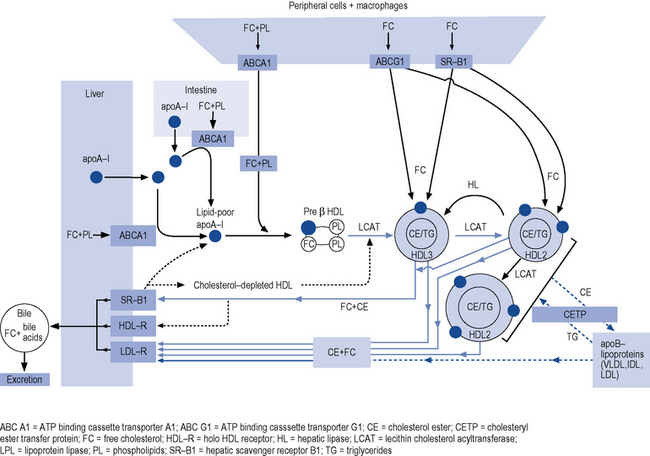
Fig. 24.2 Pathways of reverse cholesterol transport in man (Chapman et al., 2010 with kind permission from Oxford University Press, Oxford).
Triglycerides
The role of hypertriglyceridaemia as an independent risk factor for coronary heart disease (CHD) is unclear because triglyceride levels are confounded by an association with low HDL-C, hypertension, diabetes and obesity, and a synergistic effect with LDL-C and/or low HDL-C. An isolated elevation of triglyceride may be the consequence of a primary disorder of lipid metabolism, it may be secondary to the use of medicines or it may be a component of the metabolic syndrome or type 2 diabetes mellitus. Many individuals have a mixed dyslipidaemia that includes elevated levels of triglycerides and LDL-C, but reduction of LDL-C normally remains the primary focus of treatment. A recent analysis, in over 73,000 individuals, of a genetic variant which regulates triglyceride concentrations has demonstrated a causal association between triglycerides and CHD (Triglyceride Coronary Disease Genetics Consortium and Emerging Risk Factors Collaboration, 2010).
Aetiology
Primary dyslipidaemia
Familial hypercholesterolaemia
Heterozygous familial hypercholesterolaemia (often referred to as FH) is an inherited metabolic disease that affects approximately 1 in 500 of the population. In the UK, this represents about 110,000 individuals. Familial hypercholesterolaemia is caused by a range of mutations, which vary from family to family, in genes for the pathway that clear LDL-C from the blood. The most common mutation affects the LDL receptor gene. Given the key role of LDL receptors in the catabolism of LDL-C, patients with FH may have serum levels of LDL-C two to three times higher than the general population. It is important to identify and treat these individuals from birth, otherwise they will be exposed to high concentrations of LDL-C and will suffer the consequences. Familial hypercholesterolaemia is transmitted as a dominant gene, with siblings and children of a parent with FH having a 50% risk of inheriting it. It is important to suspect FH in people that present with TC >7.5 mmol/L, particularly where there is evidence of premature CV disease within the family. Guidance on diagnosis, identifying affected relatives and management are available but it is important to seek specialist advice for this group of high-risk patients (National Institute of Health and Clinical Excellence, 2008b).
Lipoprotein(a)
Concentrations of Lp(a) above 0.3 g/L occur in about 20% of caucasians and increase the risk of coronary atherosclerosis and stroke. Under a wide range of circumstances, there are continuous, independent, and modest associations of Lp(a) concentration with the risk of CHD and stroke (Emerging Risk Factors Collaboration, 2009).
Secondary dyslipidaemia
Dyslipidaemias that occur secondary to a number of disorders (Box 24.1), dietary indiscretion or as a side effect of drug therapy (Table 24.2) account for up to 40% of all dyslipidaemias. Fortunately, the lipid abnormalities in secondary dyslipidaemia can often be corrected if the underlying disorder is treated, effective dietary advice implemented or the offending drug withdrawn.
Some of the more common disorders that cause secondary dyslipidaemias include the following.
Diabetes mellitus
Obesity
Chronic, excessive intake of calories leads to increased concentrations of triglycerides and reduced HDL-C. Obesity per se can exacerbate any underlying primary dyslipidaemia. Individuals with central obesity appear to be at particular risk of what has become known as the metabolic or DROP (dyslipidaemia, insulin resistance, obesity and high blood pressure) syndrome which represents a cluster of risk factors. Obesity and sedentary lifestyle coupled with inappropriate diet and genetic factors interact to produce the syndrome (Kolovou et al., 2005).
Risk Assessment
Primary prevention
In patients with no evidence of CHD or other major atherosclerotic disease, there are a number of CVD risk prediction charts, including those produced by the Joint British Societies (JBS2) (British Hypertension Society, 2009) for males (Fig. 24.3) and females (Fig. 24.4). JBS2 recommends that all adults from the age of 40 years, with no history of CVD or diabetes, and not receiving treatment for raised blood pressure or dyslipidaemia, should receive opportunistic screening every 5 years in primary care. The cardiovascular risk calculated using the JBS2 charts is based on the number of cardiovascular events expected over the next 10 years in 100 women or men with the same risk factors as the individual being assessed. Those with a cardiovascular risk >20% over 10 years are deemed to require treatment according to current national and international guidelines, although individuals with a risk as low as 8% over 10 years will gain some benefit, this will be small.