Drugs Used in Disorders of the Central Nervous System and Treatment of Pain
Overview
The nervous system, derived from ectoderm, begins with embryonic disk formation. The neural tube develops bulges, bends, and crevices that form mature brain structures and ventricles. Three major bulges appear by approximately day 28 of gestation: the forebrain (prosencephalon), midbrain (mesencephalon), and hindbrain (rhombencephalon). At approximately day 36, the posterior (caudal) portion of the forebrain develops into the diencephalon; the anterior part develops into the telencephalon (eventually cerebral hemispheres). The cerebral cortex has a specific outline by 6 months but develops sulci and gyri only in the 3 months before birth. The developing brain is affected, especially in the first trimester, to injuries caused by various chemicals such as drugs. Various neurotransmitters and growth hormones play critical roles in development of normal CNS function and restoration of function after injury. Efforts aimed to identify these substances and design drugs that will facilitate or enhance their actions are ongoing.
Cerebral hemispheres are separated by a fissure and falx cerebri but are connected by commissures and other structures. The medial brain surface reveals complex, highly organized, structures of the hemispheres. The spinal cord and the brain (ie, the CNS) merge at the level of the brainstem. The major connection between the 2 hemispheres is the corpus callosum. Important sites of CNS drug effects are in the limbic system—communicating structures involved with smell, memory, and emotion. Four communicating cavities (ventricles) in the brain contain CSF produced by choroid plexuses. CSF circulation—from ventricles to central canal of spinal cord to drainage in venous sinuses—provides protection against trauma and a way to communicate chemically. Structures respond to circulating substances (eg, neurotransmitters, neuropeptides, hormones), as evidenced by introducing substances into CSF. The central action of a drug is studied by direct injection into ventricles.
Although many, if not most, brain functions involve coordinated interaction among multiple brain structures and each portion of the brain is connected to almost every other portion, some functions are loosely associated with certain regions. For example, the somatosensory (motor-sensory and sensorimotor) regions of the frontal and parietal lobes and the premotor cortex of the frontal lobe are involved with initiation, activation, and performance of motor activity and reception of primary sensations. Interconnections among parietal (integration and interpretation of sensory information), temporal (reception and interpretation of auditory information), and occipital (vision) lobes provide an organized, integrated system. The prefrontal cortex is involved with higher mental functions. Association pathways provide added organized communication via intrahemispheric and interhemispheric connections.
The CNS comprises many types of neurons. In general, myelinated neurons conduct impulses more rapidly than do nonmyelinated neurons. The magnitude of the electrical potential difference across the neuronal membrane in the resting state, termed the resting membrane potential, depends on the relative intracellular and extracellular concentrations of Na+ and Cl− (higher on the outside) and K+ (higher on the inside). The cytoplasmic electrical potential is more negative than the extracellular fluid by approximately −70 mV. The potential difference is partly maintained by an Na+/K+ active transport exchange mechanism (ion pump). If the membrane is depolarized from its resting potential to approximately −40 mV (threshold potential), an action potential develops: the membrane potential continues to increase to approximately +20 to +30 mV and then returns to its resting level, in approximately one thousandth of a second. The frequency of a neuron’s firing is one mechanism by which information is encoded within the CNS.
Synaptic activation can either excite or inhibit a postsynaptic cell. During chemical synaptic transmission, neurotransmitters change postsynaptic membrane permeability to ions. For example, increased permeability to Na+ produces excitation, and increased permeability to K+ and Cl− produces inhibition. The former manifests as a depolarizing change in the transmembrane potential (EPSP), and the latter manifests as a hyperpolarizing change (IPSP). Each neuron receives input from many other neurons, so a membrane potential is a net influence of EPSPs and IPSPs. Excitatory neurotransmitters such as Glu and Asp produce EPSPs; inhibitory neurotransmitters such as GABA and Gly produce IPSPs. Drugs that enhance Glu or Asp action (or otherwise enhance EPSPs) (eg, low nicotine doses) have excitatory effects in the CNS; drugs that enhance GABA or Gly action (or otherwise enhance IPSPs) (eg, diazepam) have inhibitory CNS effects.
Many substances within the CNS modulate neurotransmitter actions. ACh and norepinephrine (NE), predominant in the PNS, also function in the CNS. Dopamine and 5-HT (serotonin)—more prominent in the CNS—and peptides such as endorphins are important in CNS function. Transduction mechanisms for neurotransmitter action are similar to those in the PNS: ionotropic types include voltage-gated ion channels (respond to membrane potential changes) and ligand-gated ion channels (alter membrane ion permeability in response to ligands such as neurotransmitters or drugs). Metabotropic types include GPCRs and involve second-messenger pathways (affect ion channels or biochemical reactions). Drugs affect various sites along neuronal pathways, including neurotransmitter synthesis, storage, and release; receptor activation and inhibition; modulation of intrasynaptic neurotransmitter metabolism or reuptake; and direct second-messenger pathway effects.
Many CNS depressants, including alcohols, barbiturates, benzodiazepines, and carbamates, produce sedation (reduction of anxiety) or hypnosis (induction of sleep). Sedative-hypnotics show considerable chemical diversity but share an ability to modulate Cl− influx via interaction with the GABAA receptor–Cl− channel complex, a heteroligomeric glycoprotein comprising 5 or more membrane-spanning subunits. Various subunit combinations give rise to multiple receptor subtypes. GABA enhances Cl− influx by binding to α or β subunits. Cl− influx hyperpolarizes the neuron and makes it less likely to fire in response to stimulation (EPSPs). Barbiturates depress neuronal activity by facilitating and prolonging inhibitory effects of GABA and Gly by interacting with Cl− channel sites and increasing the duration of GABA-mediated channel opening. Benzodiazepines (see Figure 3-9) bind to specific receptor sites on the complex and increase the frequency of GABA-mediated channel opening.
To experience anxiety is normal. However, clinical anxiety is tension or apprehension that is grossly disproportionate to an actual or perceived stimulus. The source of anxiety may not be apparent and indeed may not be external; an underlying biochemical defect and genetic predisposition are hypothesized. Clinical anxiety, whether chronic or in the form of a panic attack, often produces somatic symptoms, impedes normal functioning, and adversely affects the quality of life. The disorders are approximately twice as common (possibly more often reported) in women than in men. The age at onset is usually between 20 and 30 years. Both endogenous and external factors likely contribute to susceptibility and expression of the clinical problem. Common adult anxiety disorders include generalized anxiety disorder, social phobia, OCD, panic disorder, and posttraumatic stress syndrome. Drugs for treating anxiety disorders, or anxiolytics, include benzodiazepines and buspirone.
Two main categories of anxiolytics are benzodiazepines and miscellaneous (eg, buspirone, zolpidem, zaleplon). Subclassification of benzodiazepines is based on speed of onset or duration of action, metabolism, and adverse effects. Benzodiazepines cross the blood-brain barrier and bind to specific receptors on the GABAA complex; these receptors occur in many brain regions. The drugs do not bind to the same sites as does GABA but potentiate GABA action. Benzodiazepines are safer than barbiturates (largely obsolete); adverse effects include dependence, ataxia, and drowsiness. Diazepam, chlordiazepoxide, prazepam, and the prodrug clorazepate undergo hepatic metabolism to the intermediate oxazepam. Alprazolam, flurazepam, lorazepam, and triazolam directly undergo conjugation before excretion. Zolpidem and zaleplon resemble benzodiazepines in pharmacology but differ chemically. Buspirone (an azapirone) acts on 5-HT1A receptors. These last drugs have fewer adverse effects and less abuse potential.
Seizures have various causes, both internal (intracranial) and external (extracranial). However, many seizures, perhaps the majority, are idiopathic. Internal causes include congenital defects, inborn errors in metabolism, infection, trauma, fever, intracranial hemorrhage, and malignancy. External causes include metabolic, electrolyte, and other biochemical disorders; anoxia; and hypoglycemia as well as excess doses of drugs or abrupt cessation of drugs. Approximately 10% of the US population has a seizure by the age of 80 years. Epilepsy, a type of seizure disorder, is a heterogeneous symptom complex characterized by recurrent, unprovoked seizures and affects approximately 1% of the population. For optimal drug therapy, the specific type of epilepsy should be identified. The principal mechanism of action of most current antiepileptic drugs involves action on voltage-gated ion channels or on inhibitory or excitatory neurotransmitter function.Stay updated, free articles. Join our Telegram channel

Full access? Get Clinical Tree
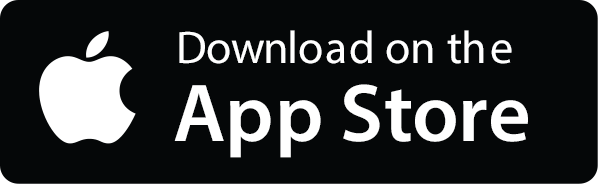
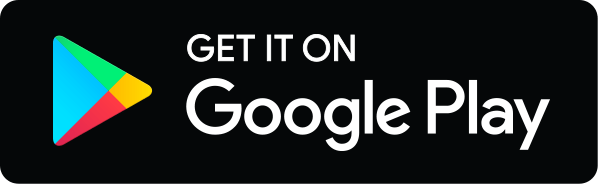