Drugs Used in Disorders of the Cardiovascular System
Overview
The heart muscle pumps blood through the circulatory system. Each day, the heart beats 100,000 times and pumps 2000 gal of blood. The heart is composed of 4 chambers (divisions): the upper two, the right and left atria; the lower two, the right and left ventricles. Blood is pumped through the chambers, in only 1 direction, via 4 valves: the tricuspid, located between the right atrium and the right ventricle; the pulmonary, between the right ventricle and the pulmonary artery; the mitral, between the left atrium and the left ventricle; and the aortic, between the left ventricle and the aorta. Dark blood, low in oxygen, returns from body tissues through veins, enters the right atrium, and then flows to the right ventricle, the pulmonary artery, and the lungs, where it is oxygenated. Blood returns by pulmonary veins to the left atrium and goes through the mitral valve into the left ventricle, which pumps oxygen-rich, bright-red blood through the aortic valve into the aorta and then into the circulation.
Cardiac output is the total blood volume pumped by ventricles per minute (heart rate × stroke volume). Stroke volume is the blood pumped by the left or right ventricle per beat; in a resting adult, it averages 60 to 80 mL of blood. Systole is the contraction phase of the cardiac cycle, when ventricles pump stroke volumes. Diastole is the resting phase of the cycle, which occurs between heartbeats. End-diastolic volume is the blood volume in each ventricle at the end of diastole: 120 mL at rest. End-systolic volume is the blood volume in each ventricle after contraction: 50 mL at rest. To maintain equal flow through pulmonary and systemic circuits, the left and right ventricles maintain the same cardiac output. The resting cardiac output is 4.8 to 6.4 L/min. Cardiac output increases (20-85%) during intense exercise to transport more oxygen to muscles. This greater blood flow is caused by higher blood pressure and arteriolar vasodilation in muscles, which is due to smooth muscle relaxation.
Norepinephrine and epinephrine (EPI), major catecholamine regulators of heart function, are released by the adrenal medulla after activation of preganglionic sympathetic nerves, which occurs during stress (eg, exercise, heart failure, pain). More EPI (85%) than NE (15%) is released. A second source of NE is that from sympathetic nerves, especially those innervating cardiac pacemaker cells. The sympathetic effects increase heart rate and contraction force by activating β1 adrenoceptors; vasoconstriction in systemic arteries and veins by activating α-adrenoceptors; vasodilation in skeletal muscle at low concentrations by activating β2 receptors; and vasoconstriction at high concentrations by activating α1 receptors. The overall cardiovascular response is greater cardiac output plus a small mean arterial pressure change. EPI release has similar cardiac effects. Heart rate, first increased by NE, usually decreases because of baroceptor activation and vagal-mediated heart rate slowing.
Sympathetic and parasympathetic systems innervate the heart and regulate function. Activation of the former increases heart rate and contraction force by increasing EPI and NE release. The latter system stimulates ACh release and reduces heart rate. The pacemaker cells of the SA node depolarize and promote atrial contraction. Ventricular contraction is due to impulses going from the AV node to the AV bundle to Purkinje fibers. Increased sympathetic drive activates β1 receptors in the SA node and increases pacemaker cell depolarization rate, heart rate, and contraction strength. Parasympathetic impulses (through vagus nerves) reduce heart rate, AV node conduction, and contraction force. Increased ACh release and muscarinic M2 receptor activation mediate these effects. M2 receptor activation reduces cellular cAMP levels and increases K+ conductance, which leads to pacemaker cell hyperpolarization. Reduced heart rate and contraction force result.
Norepinephrine synthesis starts with the amino acid tyrosine. Catecholaminergic nerves obtain it by active transport; tyrosine hydroxylase adds a hydroxyl group to form the catechol part of the molecule. Tyrosine hydroxylation is the rate-limiting step in catecholamine synthesis and is regulated by feedback inhibition. The product dihydroxyphenylalanine (dopa) is converted by aromatic amino acid decarboxylase into dopamine, one of 3 naturally occurring catecholamines. Dopamine enters synaptic vesicles via a catecholamine pump and is converted to NE by addition of a hydroxyl group. Synaptic vesicle catecholamine levels are much higher than surrounding cytosolic levels. Reserpine is a drug that inhibits the vesicular catecholamine pump, thus stopping vesicular catecholamine uptake and reducing catecholamine levels. The low cytosolic catecholamine level in nerves is maintained by the vesicular amine uptake pump and by mitochondrial monoamine oxidase, which degrades catecholamines.
Vesicular release of NE depends on depolarization of the nerve terminal and the influx of Ca2+ ions. The influx of Ca2+ promotes the docking of synaptic vesicles at the plasma membrane and subsequent exocytosis of the vesicles. In the adrenal medulla, ACh acting as the neurotransmitter of the sympathetic ganglion acts on nicotinic receptors and promotes the release of catecholamines into the circulation. Certain drugs can also promote catecholamine release. Under certain experimental conditions, it is possible to mimic this nicotinic effect of ACh not only at the adrenal medulla, but at also at the sympathetic ganglia. Thus, activation of cholinergic receptors by nicotinic agonists evokes substantial catecholamine release from postganglionic neurons and the adrenal medulla.
The primary NE inactivation mechanism is reuptake via a plasma membrane amine transporter, the amine uptake pump. This transporter is a member of a family of membrane proteins that transport different transmitter substances across the plasma membrane of the nerve terminal. The amine uptake transporter is driven indirectly by a sodium gradient, is selective for NE and EPI, and is inhibited by cocaine and tricyclic antidepressants such as imipramine. NE uptake is a major mechanism for ending sympathetic nerve transmission. Inhibitors of the amine transporter potentiate responses to stimulation of the sympathetic nervous system or to injected compounds that are taken up by sympathetic nerve terminals. In a sympathetically innervated tissue, such as the heart, the major uptake of catecholamines is neuronal uptake.
Cholesterol, a simple lipid found in cell membranes, is a precursor of steroids, bile acids, and vitamin D and a major part of atherosclerotic plaques. Most circulating blood cholesterol is synthesized from liver acetyl CoA and is excreted as bile salts. Only 25% of blood cholesterol is from the diet, but high-fat diets increase liver cholesterol production and blood cholesterol levels. HMG-CoA formation from HMG-CoA reductase, the rate-determining step in cholesterol synthesis, is regulated via feedback inhibition. When cholesterol uptake is low, the liver and small intestine increase cholesterol synthesis. The plaque-forming ability of cholesterol is related to LDLs, which promote plaque formation; HDLs remove cholesterol from arteries and transport it to the liver. HDLs remove cholesterol from plaques and slow atherosclerosis. Control of cholesterol and LDL levels is a major goal in heart disease therapy.
Primary goals of therapy are lower LDL levels and higher HDL levels. The best drugs for such therapy are statins: lovastatin, fluvastatin, pravastatin, simvastatin, and atorvastatin. They interfere with the cholesterol production of the liver by blocking HMG-CoA synthesis, so the liver can better remove cholesterol from circulating blood. Statins lower LDL cholesterol by 60%; side effects can occur. Nicotinic acid (or niacin) lowers total and LDL cholesterol and raises HDL cholesterol levels, but it can be toxic because the therapeutic dose is 100-fold greater than the recommended daily allowance. Resins (eg, cholestyramine and colestipol) bind intestinal bile acids and prevent recycling through the liver. The liver needs cholesterol to make bile, so it increases uptake of cholesterol from blood. Fibric acid derivatives decrease triglyceride and increase HDL levels. Low doses of aspirin block platelet thromboxane A2 synthesis, which leads to reduced platelet aggregation and blood viscosity.
Angina, or angina pectoris, is a gripping pain felt in the center of the chest that may move to the neck, jaw, and arms and is caused most often by exercise; emotion, eating, and cold weather are other causes. It occurs when the heart receives deficient oxygen because of blood vessel narrowing, which results mainly from aging and also from cigarette smoking, high cholesterol levels, obesity, and diabetes. The 3 types are stable angina (exertional or typical angina), caused by atherosclerosis, with treatment to reduce cardiac load and increase myocardial blood flow; vasospastic angina (variant or Prinzmetal angina), caused by severe coronary vessel contraction, with chest pain at rest and drugs aimed to stop vasospasm; and unstable angina (crescendo angina), in which pain occurs without stress. Nitrates and β blockers are used, as are calcium channel antagonists if the mechanism is vasospasm. Reducing platelet function and thrombotic episodes helps decrease mortality in unstable angina.Stay updated, free articles. Join our Telegram channel

Full access? Get Clinical Tree
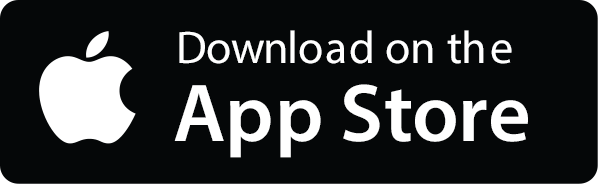
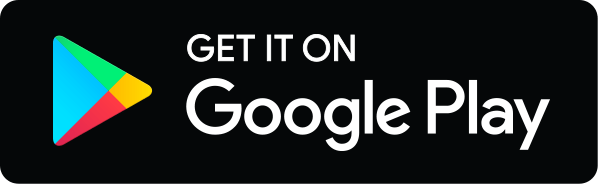