, Kyle John Wilby2 and Mary H. H. Ensom1
(1)
Faculty of Pharmaceutical Sciences, The University of British Columbia, Vancouver, Canada
(2)
College of Pharmacy, Qatar University, Doha, Qatar
In this chapter, we describe the potential for drug interactions for various antimalarial drugs based on their known metabolic properties. These antimalarials include the following: chloroquine, amodiaquine, sulfadoxine and pyrimethamine, mefloquine, primaquine, atovaquone, proguanil, quinine, artemisinin, artesunate, artemether, and dihydroartemisin.
3.1 Chloroquine
In vitro reaction phenotyping studies have been carried out to determine the CYP450 enzymes responsible for the N-dealkylation of chloroquine in the formation of its major metabolite, desethylchloroquine. Using a panel of recombinant human CYP450 enzymes, Projean et al. (2003) and Kim et al. (2003) demonstrated the catalytic activity of CYP1A2, CYP2C8, CYP2C19, CYP2D6, and CYP3A4 in the formation of desethylchloroquine. However, using regression analysis with marker reactions in human liver microsomes, it was determined that desethylchloroquine formation correlated only with marker reactions for CYP3A4 (midazolam 1-hydroxylation or testosterone-6β-hydroxylation) and CYP2C8 (paclitaxel α-hydroxylation) (Projean et al. 2003; Kim et al. 2003). The roles of CYP3A4 and CYP2C8 were further supported by chemical inhibition assays with probe-selective chemical modulators (i.e. quercetin for CYP2C8 and ketoconazole or troleandomycin for CYP3A4 (Projean et al. 2003; Kim et al. 2003). These findings were corroborated with the relative activity factor approach, which also suggested a role for CYP2D6 in addition to CYP2C8 and CYP3A4 in the formation of desethylchloroquine (Li et al. 2003). As enzyme-specific immunoinhibitory antibodies were not in widespread use at the time these studies were conducted, these findings were based on industry standard reaction phenotyping approaches and the reported results were consistent between the different investigative groups. Also, in addition to being a substrate for CYP450 enzymes, chloroquine itself acts as a relatively weak inhibitor for CYP2D6 (ki (inhibition constant) = 12.4–15 μM) based on in vitro experiments from two separate studies (Bapiro et al. 2001; Masimirembwa et al. 1995). Taken together, it may be proposed that the co-administration of drugs that modulate CYP2C8, CYP3A4, and CYP2D6 could have potential effects on the pharmacokinetics of chloroquine; but, chloroquine, given its weak inhibitory activities, is unlikely to have an effect on the pharmacokinetics of other CYP2D6 substrates.
3.2 Amodiaquine
The primary metabolite of amodiaquine in humans, N-desethylamodiaquine, is predominately generated by CYP2C8, as demonstrated by a series of systematic in vitro reaction phenotyping studies conducted by Li et al. (2002). Using cDNA-expressed CYP450 isoenzymes, it was determined that CYP2C8, CYP1A1, CYP1B1, CYP2D6, and CYP3A4 were capable of oxidizing amodiaquine. A high degree of correlation with the 6-alpha hydroxylation of paclitaxel, a known marker reaction of CYP2C8, and the selective inhibition by quercetin, a potent inhibitor of the same isoenzyme, indicated the primary role of CYP2C8 (Li et al. 2002). These observations were supported by relative activity factor calculations that also demonstrated CYP2C8 as the primary enzyme responsible for the N-desethylation of amodiaquine (Li et al. 2002, 2003). Because of these well-established metabolic properties, amodiaquine N-desethylation is currently used as a marker reaction for CYP2C8 (Walsky et al. 2005).
A predominant role by CYP2C8 suggests that genetic polymorphisms or concurrent medications that inhibit this isoenzyme can potentially affect the clearance of amodiaquine in humans. Parikh et al. (2007) studied metabolic properties of amodiaquine using polymorphic CYP2C8 in vitro and found significant reductions in intrinsic clearance and maximal velocity (Vmax) and increased Km (concentration of substrate that results in half Vmax) with the CYP2C8*2 allele. The same authors also reported potent inhibitory effects by efavirenz, saquinavir, lopinavir, tipranavir, and ritonavir, based on IC50 (half maximal inhibitory concentration) values, toward the oxidation of amodiaquine in cDNA-expressed CYP2C8 supersomes. The clinical significance of these effects, however, remains to be determined in humans. On the other hand, amodiaquine is not known to be a potent inhibitor of major CYP450 enzymes. As demonstrated by Bapiro et al. (2001), amodiaquine inhibited (minimally) marker reactions for CYP1A2, CYP2C9, and CYP2C19 with the inhibition constant ranging from 26 to 46 μM, indicating a low likelihood of a clinically significant drug-drug interaction with amodiaquine being the offending agent.
3.3 Sulfadoxine and Pyrimethamine
Sulfadoxine undergoes minimal hepatic biotransformation and is unlikely to be subjected to clinically significant drug-drug interactions involving biotransformation. On the other hand, pyrimethamine is predominately metabolized hepatically, although the exact biochemical pathways remain to be characterized thereby limiting the predictability of clinically relevant drug interactions.
3.4 Mefloquine
Mefloquine is predominately cleared by hepatic metabolism and in vitro experiments in human hepatocytes and microsomes (Fontaine et al. 2000; Na-Bangchang et al. 1992) have indicated CYP3A4 as the primary isoenzyme responsible for its biotransformation. Fontaine et al. (2000) demonstrated increased formation of carboxy- and hydroxy-metabolites of mefloquine in dexamethasone (inducer of CYP3A4) pre-treated human hepatocytes. In further support of a role of this specific metabolic pathway, Fontaine et al. (2000) also demonstrated potent inhibition of mefloquine oxidation by ketoconazole (a selective CYP3A4 inhibitor) in rifampin (CYP3A4 inducer)-pretreated human hepatocytes, and reported a high degree of correlation between mefloquine oxidation activity and that of erythromycin N-demethylation, a marker reaction for CYP3A4 in human liver microsomes. Similar findings were obtained by Na-Bangchang et al. (1992) in human liver microsomes where ketoconazole was shown to extensively inhibit (inhibition constant = 11.2 μM) the formation of carboxymefloquine. These findings suggest that co-administered CYP3A4 modulators can potentially affect the clinical pharmacokinetics of mefloquine. On the other hand, little is known of the potential for mefloquine to cause drug interactions. It can serve as a competitive inhibitor of CYP3A4 by virtue of being a substrate of this enzyme, but little in vitro or preclinical data are available on the effects of mefloquine on other enzyme systems/pathways in humans.
3.5 Primaquine
Primaquine is primarily metabolized to carboxyprimaquine in humans. Jin et al. (2014) conducted a reaction phenotyping study using cultured human hepatocytes, recombinant CYP450 enzymes, monoamine oxidases, and flavin-containing monooxygenases, in conjunction with chemical inhibition experiments using in vitro setups. In cultured human hepatocytes, fluvoxamine (CYP1A2 inhibitor), quinidine (CYP2D6 inhibitor), ketoconazole (CYP3A4 inhibitor), clogyline (monoamine oxidase-A inhibitor), deprenyl (monoamine oxidase-B inhibitor), and methimazole (flavin-containing monooxygenase inhibitor) were able to reduce (modestly) the degradation of primaquine under their experimental conditions. Incubations of primaquine with recombinant enzymes indicated that the same enzymes identified with chemical inhibition experiments (with more prominent effects from CYP2D6) were capable to catalyze the degradation of primaquine. A limitation, however, is that the formation of carboxyprimaquine was not determined; thus, one could not attribute the formation of this major metabolite to any of the identified metabolic pathways. In support of these findings, Na-Bangchang et al. (1992) also demonstrated, an extensive reduction of carboxyprimaquine formation by ketoconazole (CYP3A4 inhibitor) in human liver microsomes, further strengthening the role of CYP3A4 in this process. Taken together, these findings suggest that CYP2D6 and CYP3A4 may be the primary enzymes responsible for the metabolism (and the formation of carboxyprimaquine) in humans, although further reaction phenotyping studies using industry standard complementary approaches such as immunoreactive antibodies, correlational analyses, and relative activity factor determination are also needed to establish definitive conclusions. Furthermore, by virtue of primaquine being a substrate for CYP2D6 and CYP3A4, it may serve as a competitive inhibitor of these enzymes. In addition, there is suggestion that primaquine may activate CYP1A1, via the aryl hydrocarbon receptor (Fontaine et al. 1999), although further mechanistic studies are needed and it is unknown whether this inductive property of primaquine is associated with clinically relevant drug interactions.
3.6 Atovaquone
Atovaquone undergoes minimal hepatic/extra-hepatic biotransformation and is predominately excreted unchanged in feces (Rolan et al. 1997). These properties make it unlikely to be affected by interacting drugs and the available data also indicate that it does not affect the pharmacokinetics of other agents (Bapiro et al. 2001; Trapnell et al. 1998).
3.7 Proguanil
Proguanil in primarily metabolized to cycloguanil in humans. In vitro reaction phenotyping studies have been conducted by Birkett et al. (1994), Lu et al. (2000), and Coller et al. (1999) using human liver microsomes, cDNA-expressed supersomes, enzyme-selective chemical inhibitors, and enzyme-specific antibodies. All three studies were consistent in reporting, via their chemical inhibition, antibody inhibition, and correlational analysis experiments, a major role of CYP2C19 in the metabolism of proguanil. However, the same cannot be said for CYP3A4, where Lu et al. (2000) and Birkett et al. (1994) both reported significant reductions in cycloguanil formation in the presence of troleandomycin (potent CYP3A4 inhibitor) whereas Coller et al. (1999) demonstrated little inhibition of proguanil metabolism in the presence of a CYP3A4-specific immunoantibody in human liver microsomes. Likewise, Lu et al. (2000) showed little effects of furafylline (CYP1A2 inhibitor) on the formation of cycloguanil, whereas Coller et al. (1999) demonstrated a significant decrease in biotransformation of proguanil using the same chemical inhibitor in human liver microsomes. The discrepancies with respect to CYP1A2 and CYP3A4 may be attributed to differences in in vitro experimental conditions or to differences between the ethnicity of donors of human liver microsomes (Lu et al. (2000) used liver microsomes from Chinese subjects). Taken together, these data suggest that concurrent medications that can modulate CYP2C19 may cause a clinically significant change in the pharmacokinetics of proguanil, but the roles of other CYP450 enzymes need to be clarified further with mechanistic studies. On the other hand, little data are available documenting the effects of proguanil as a causative agent of drug interactions. In an in vitro experiment, proguanil has been shown to lack inhibitory effects toward major CYP450 enzymes in humans (Bapiro et al. 2001).
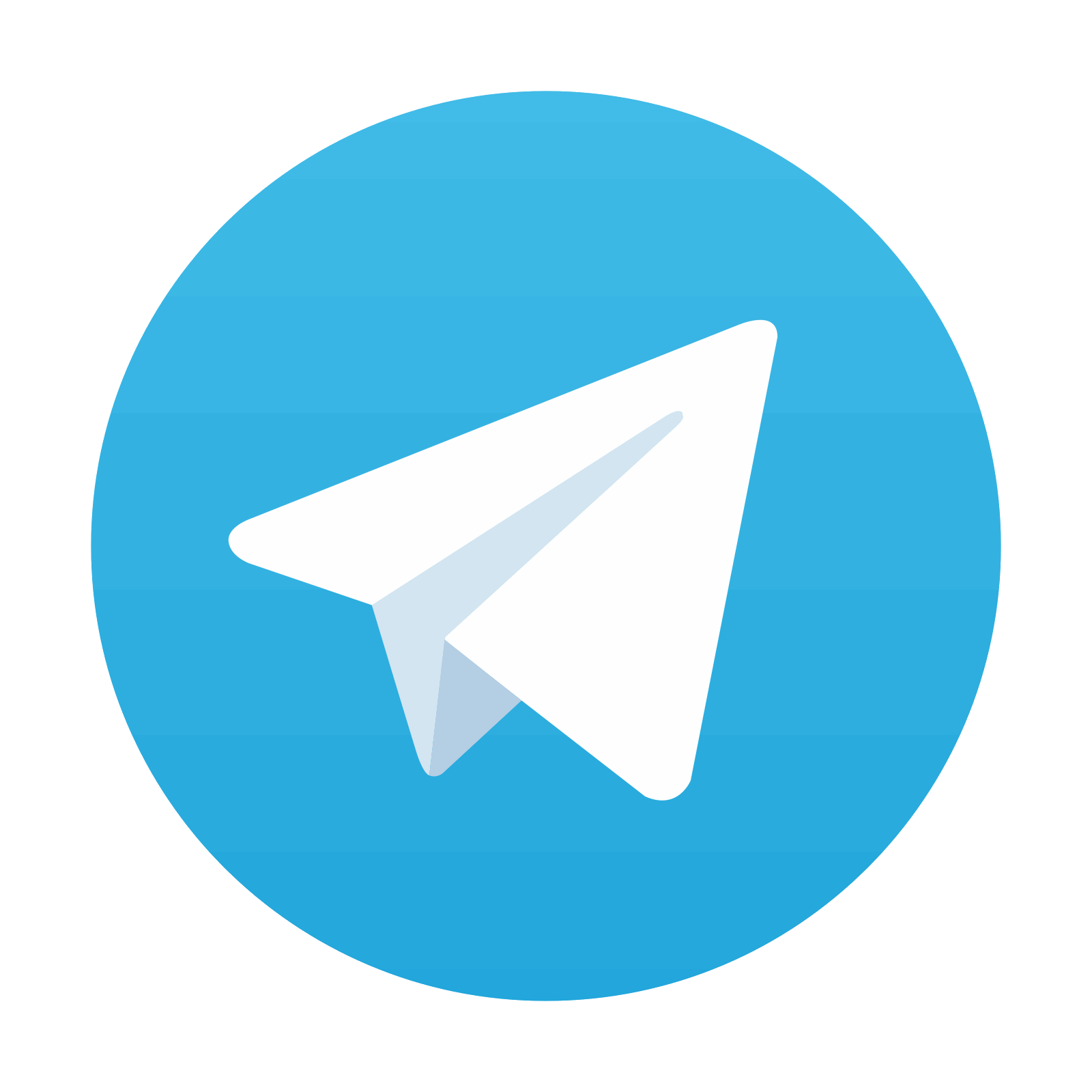
Stay updated, free articles. Join our Telegram channel

Full access? Get Clinical Tree
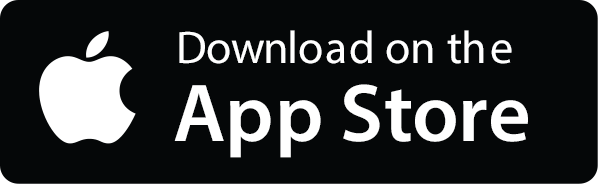
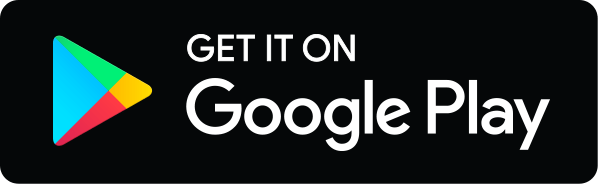