Fig. 9.1
TAM-regulated suppression of inflammatory response in sentinel cells of the innate immune system. Activation of TLR results in the secretion of pro-inflammatory cytokines, which are in positive feed-forward loop with their receptors (a). TAM-mediated activation of SOCS1 (b) and SOCS3 (c) inhibits signaling of MAL and TRAF6, subsequently resulting in immunosuppressive environment
Furthermore, SOCS1 and SOCS3 can inhibit JAK/STAT1 signaling and subsequently impair synthesis of pro-inflammatory cytokines, such as IL-1, IL-6, IL-12, CCL9, CCL10, and TNF-α [23]. Additionally, the activation of Axl and/or Mer stimulates Twist activation, which transcriptionally suppresses TNF-α expression [24, 25]. Thus, as a consequence of the interaction with SOCS proteins, TAM receptors govern the repression of the pro-inflammatory cascade by blocking TLR signaling and hindering cytokine-receptor feed-forward signaling loops.
TAM signaling is also essential for efferocytosis, the phagocytic clearance of apoptotic cells [26]. Efferocytosis initiates an immunosuppressive signal in phagocytic cells, which is a critical defense against autoimmunity and is required for the maintenance of tissue homeostasis [27]. However, tumors are able to co-opt this biologic process to evade immune surveillance [28]. TAM receptors regulate phagocytosis of apoptotic cells and inhibit inflammation and immune activation; however, the exact mechanism remains unclear.
As described above, TAM signaling profoundly influences the function of sentinel cells. Specifically, TAM activation has an inhibitory effect on the innate immune response, and the downregulation of TAM signaling promotes autoimmunity. TAM receptors, despite their similarity, are likely to perform distinct functions. Axl and Mer were identified as central in the control of the innate immune system, yet they exhibit divergent expression and activity depending on the condition of surrounding tissue. While both are immunosuppressive phagocytic receptors, Mer appears to be a principal RTK in the tolerogenic environment, whereas Axl operates during inflammation [29]. Furthermore, Axl and Mer are differentially dependent on their ligands. Axl is activated by dimerization through binding to Gas6 in a 2:2 stoichiometry [30]. Gas6 is a ligand for each TAM receptor, whereas Tyro3 and Mer are also activated by protein S [31]. Gas6 is required for the activation of Axl, whereas Axl maintains Gas6 expression in vivo [29]. This codependence and continuous presence of Gas6-Axl complex suggest that there may be another trigger of full Axl activation. Indeed, it was shown that basal Gas6-Axl activity was greatly enhanced upon exposure to apoptotic cells with membranes rich in externalized PtdSer, and therefore PtdSer appears to be a critical stimulus driving Axl activation [29]. How PtdSer contributes to Gas6-induced Axl activation is still not clear. The immunoglobulin-like (Ig) domains of Axl function as a docking site for the laminin G-like (LG) domains of Gas6, which drives Axl dimerization [30]. PtdSer appears to stabilize Axl dimerization and allows optimal Axl signaling [32]. Furthermore, Axl can be activated through ligand-independent dimerization with other TAM receptors or members of other RTK families, such as ErbB [33, 34]. In contrast Mer has only one docking site for Gas6 and exhibits a much lower affinity for its ligands.
TAM signaling is also important for the maturation and differentiation of NK cells [35]. NK cells are an essential component of the innate immune system that recognizes infected or malignant cells in the absence of “education” or priming [36]. In the presence of Gas6 or protein S, TAM receptors expressed by immature NK cells are activated. TAM signaling stimulates the acquisition of inhibitory, e.g., members of Ly49 and CD94 families, or activating receptors (CD69) required for target-cell recognition [37, 38]. Thus, TAM-deficient NK cells lack inhibitory and activating receptors and fail to secrete certain cytokines, such as macrophage-inducing IFNγ even though they produce normal levels of perforins and granzymes [35]. This results in NK cells that have a tenfold lower activity against target cells [35].
The signaling of principal TAM receptors, Axl and Mer, is particularly important in sentinel cells of the immune system [12]. Signaling of either receptor drives an intrinsic negative feedback for the immune activation. Importantly, activation of Axl and Mer is specific for different environments, where Axl is specialized to function in inflammatory and Mer in tolerogenic settings [29]. Accordingly, the functional divergence of these receptors can have adverse repercussions for the development of new therapies. TAM activation can be beneficial for patients suffering from autoimmune diseases [39]. Long-term inhibition of Mer could possibly disturb homeostasis of healthy tissues and may result in many adverse side effects. On the other hand, prolonged Axl inhibition may be less harmful and potentially advantageous for cancer patients. Hence, antibody-based [40] or highly selective targeted treatments [41] are promising approaches to treat human disease through modulation of TAM signaling.
Phosphatidylserine in Inflammation
PtdSer is an anionic phospholipid that is actively segregated to the inner leaflet of the plasma membrane. In apoptotic cells PtdSer is flipped to the outer leaflet of the membrane where it functions as a signal for macrophages to engulf the cell [42]. PtdSer is externalized on the vascular endothelial cells in tumors [43] and is constitutively present in the outer leaflet of some tumor cells [44]. PtdSer externalization on tumor cells is significantly increased when they undergo apoptosis upon chemo- or radiotherapy [45, 46]. PtdSer is an evolutionarily conserved driver of immunosuppression in the tumor microenvironment [47], a topic that has recently been reviewed [48]. As mentioned above, PtdSer is required for full activation of TAM receptors [29, 32], whose signaling has an inhibitory effect on the innate immune response [12]. Specifically, low basal activity of Axl-Gas6 complex is significantly reinforced upon bridging with PtdSer [29]. Furthermore, formation of the Axl-Gas6-PtdSer complex is necessary for phagocytosis of apoptotic cells by macrophages [10].
PtdSer-dependent efferocytosis triggers secretion of anti-inflammatory mediators TGFβ and IL-10 by macrophages [49]. Furthermore, tumor microenvironments rich in PtdSer are characterized by diminished adaptive immune response. For instance, intratumoral dendritic cells, upon engulfment of PtdSer-opsonized cells, maintain an immature phenotype, resulting in failed antigen presentation [50]. Also, PtdSer has been linked to suppression of cytotoxic T-cell responses [51]. PtdSer-rich microvesicles, released by lymphocytes upon TCR activation, also transmit cell-death signals to activated T cells [52, 53]. Lymphocyte-derived microvesicles may induce apoptosis and nonreversible inhibition of T-cell function by suppression of CD3-ζ chain T-cell receptor, which plays an important role in antigen recognition [53] or by expression of apoptosis-inducing ligands, such as Fas ligand (FasL), programmed death ligand (PDL), and TNF-related apoptosis-inducing ligand (TRAIL) [54]. Further, PtdSer present on the lymphocyte-derived microvesicles has been postulated to modulate T-cell activation in a reversible fashion [51]. As such, PtdSer seems to enhance the activity of diacylglycerol kinase-α (DGK-α) [51], which are found in excessive amounts in anergic T cells [55]. Although primarily reversible, suppression of T-cell function through contact with PtdSer may become nonreversible, as a consequence of chronic exposure to PtdSer-rich membranes and result in T-cell exhaustion [51, 56].
PtdSer also activates a second class of receptors, T-cell immunoglobulin and mucin receptors (TIMs) [57]. There are three subtypes of TIM receptors (TIM-1, TIM-3, and TIM-4). All TIM family members have been shown to recognize PtdSer through a conserved N-terminal IgV extracellular domain [58–60]; however, their expression varies between different immune cells [57]. TIM-1 is highly expressed on T helper 2 (TH2) cells and functions as a co-stimulatory receptor important for T-cell activation [57]. TIM-3 is preferentially expressed on TC1 (a subset of CD8+ lymphocytes producing IFNγ) and TH1 cells and mediates apoptosis of those cells [57]. TIM-3 expressed by dendritic cells and macrophages enhances phagocytosis of apoptotic cells and antigen presentation [57]. TIM-4 is important for the maintenance of a tolerogenic state and phagocytosis of apoptotic cells and is solely expressed by antigen-presenting cells [57, 60].
TIM-3 is known to be immunosuppressive when activated and is thought to be a biomarker for T-cell exhaustion [61]. Hence, its function in cancer has been studied extensively. Recent studies on TIM-3 signaling revealed that TIM-3 abrogates Lck function and prevents TCR signal transduction [62]. Lck-mediated phosphorylation can be however rescued by HLA-B-associated transcript 3 (Bat3), which was postulated to positively regulate catalytic activity of Lck and, as a result, prevent the induction of T-cell exhaustion [63]. Consequently, the shortage of Bat3 expression in T cells causes accumulation of inactive Lck [63], which reduces the efficacy of the T-cell receptor (TCR) complex.
In summary, PtdSer, by impinging upon multiple innate and adaptive pathways, functions as a negative regulator of immune responses in the tumor microenvironment. It has shown that PtdSer can serve as a versatile biomarker of tumor cells [64] and tumor vasculature [43], as well as a novel target for therapeutic approaches [47].
Epithelial-Mesenchymal Transition
Epithelial-mesenchymal transition (EMT) is a biological process in which epithelial cells adopt a mesenchymal phenotype [65]. EMT endows epithelial cells that normally are polarized and interact with a basement membrane, with motile and invasive properties, and increased production of extracellular matrix components (ECM) [65].
EMT is critical for morphogenesis in the developing embryo [66]. In the adult tissue, EMT is restricted and occurs primarily during wound healing or organ regeneration and fibrosis [67, 68]. More recently, EMT has been linked to cancer progression [69] and is thought to be a critical mechanism in the acquisition of a metastatic phenotype in epithelial tumors [70, 71].
Physiologically, regulatory networks exist that function to support the homeostasis of every cell; however, when these networks are disturbed, dedifferentiation may be triggered [72]. It was proposed that during primary tumor formation, tumor cells become receptive to EMT inducers whose source is often the tumor-associated stroma [65, 71]. Thus, changing microenvironmental conditions regulate epithelial-mesenchymal plasticity of tumor cells. There are several major players involved in EMT induction, such as HGF, EGF, PDGF, and TGF-β [73–76]. These in turn act on a range of transcription factors that directly or indirectly orchestrate EMT machinery [70]. EMT and its reverse process MET are regulated by at least four different regulatory networks, transcriptional control, noncoding RNA regulation, differential splicing and posttranslational control, where EMT-inducing transcription factors play a central role in this network, as they are interconnected with remaining regulatory layers [72, 77–79].
To undergo EMT, an epithelial cell must be in a permissive state, allowing it to circumvent normalizing cues from the microenvironment [72, 78]. In the case of tumors, inflammation and hypoxia are commonly known environmental factors that disturb the equilibrium between these regulatory networks [69] and promote cellular transition [78]. Response to oxygen levels is mainly regulated by hypoxia-inducible factor 1α (HIF1α) , expression of which correlates with metastasis in many cancers [80–82]. HIF1α upregulates expression of zinc transcript protein 1 (SNAI1) , which in turn functions as a negative regulator of transcription of the cell-cell adhesion molecule E-cadherin [83]. In consequence of disturbed formation of adherens junctions within the epithelium, cells become motile and able to avoid anoikis [84–86].
There are more transcription factors, such as zinc finger protein 2 (SNAI2), zinc finger E-box-binding homeobox 1 (ZEB1), zinc finger E-box-binding homeobox 2 (ZEB2) and Twist family BHLH transcription factor 1 (TWIST1) [87–89], that repress the expression of several junctional proteins such as E-cadherin, claudins or desmosomes and promote EMT [84–86]. EMT transcription factors also have exceptionally high potency in causing genome-wide changes in gene expression by their interaction with epigenetic modulators [90]. Among the epigenetic mechanisms, DNA methylation, histone modifications, and microRNA expression changes have been observed in cells undergoing EMT [91]. As an example SNAI1 expression is associated with methylation of regulatory regions of CDH1 [92], where methylation represses CDH1 expression and promotes EMT [93, 94]. SNAI1 was also found to stimulate histone deacetylase activity, which in turn silences the CDH1 promoter [95]. High SNAI1 expression also correlates with immunosuppression in melanoma, which is demonstrated by impaired DC recruitment and poor infiltration of cytotoxic T cells [96].
Among the microRNAs (miRNAs) that are implicated in EMT are members of the miR-200 and miR-34 families. They are in a reciprocal feedback loop with ZEB1/ZEB2 [97, 98] and SNAI1 [99], respectively, and are known to be drivers of an epithelial phenotype [100]. Therefore miR-200 and miR-34 family members are considered to be tumor suppressors [101]. A recent work by Chen et al. [102] suggests that the miR-200/ZEB1 axis regulates EMT and PD-L1 expression on tumor cells, and it is therefore an indirect cause of immunosuppression. Hence, miR-200 and ZEB1 are in a negative feedback loop, and, while tied to EMT regulation, they mediate CD8+ T-cell impairment via increased PD-L1 expression, which promotes tumor growth and metastasis.
Overexpression of EMT-related transcription factors, such as SNAI1, SNAI2, ZEB1 and ZEB2, and TWIST1, also leads to increased expression of Axl [14]. Moreover, high levels of Axl expression support the maintenance of a mesenchymal phenotype by stimulating SNAI1, SNAI2 and TWIST expression [14, 41]. Bearing in mind that Axl is involved in sustaining mesenchymal traits, such as motility and invasiveness, the essential role of Axl in tumor initiation and metastasis takes on additional clarity [41, 103]. The elevated Axl expression is linked with unfavorable overall prognosis in breast cancer [14], non-small cell lung carcinoma [104], pancreatic cancer [105], glioblastoma multiforme [106], esophageal adenocarcinoma [107], acute myeloid leukemia [108] and ovarian cancer [109]. Significantly, abundant expression of Axl is predominantly related to metastatic dissemination and survival, rather than primary tumor growth [14, 41, 103, 110].
Many recent studies have revealed elevated expression of Axl in tumors resistant to chemotherapies [34, 73, 111–114]. More importantly, these studies suggest that chemoresistance is a repercussion of EMT [115], while inducing MET restores sensitivity. Axl expression is associated with an aggressive mesenchymal phenotype and with immunosuppression. Thus inhibition of Axl signaling has the potential to reduce tumor invasion and immunosuppression. However, while high Axl levels have been correlated with poor clinical outcome, the predictive value of Axl expression for immune phenotype has not been yet evaluated.
Normal and neoplastic epithelial cells that undergo EMT acquire stem cell properties [116, 117]. Interestingly, EMT seems to serve as a universal mechanism for the acquisition of stem-like traits, and therefore epithelial cells within tumors appear to undergo the same program as normal antecedent cells [116, 118]. The mesenchymal phenotype is associated with increased motility, invasiveness, and resistance to apoptosis, all traits that predispose to metastatic dissemination [119, 120]. Furthermore, cancer stem cells (CSCs) are thought to have the ability to initiate tumors at distant sites upon MET [121] and form macrometastases through self-renewal [118]. The invasive phenotype of these cancer stem cells is a reflection of their plasticity and ability to reversibly change phenotype across the epithelial-mesenchymal axis [122]. Importantly CSCs are associated with drug resistance and tumor recurrence [121, 123–128]. As a result, CSCs are thought to be a major cause of treatment failure, and development of targeted therapeutics directed against them is an attractive concept [121]. However, while CSCs can give rise to a new tumor that includes non stem-like tumor cells, the reverse is also true, implying that CSCs may be regenerated from non-cancer stem cells within a treated carcinoma [129]. Therefore, propitious therapy should include agents targeting CSC and normal cancer cells, eliminating both CSCs and their descendants [130].
Immunoregulation
EMT, genotypic and phenotypic heterogeneity, and dynamic epigenetic interactions have been linked with tumor relapse and resistance to systemic therapy [131]. Also, reciprocal interactions between tumor cells and components of the surrounding microenvironment, such as soluble inflammatory mediators, can confer resistance to therapy [132]. Additionally, therapy-induced injury, which occurs in response to systemic treatment disrupting established tumor structure, causes secretion of pro-inflammatory cytokines, which can mediate tumor cell plasticity. For instance, chemotherapy, in parallel to killing cancer cells, stimulates macrophages to produce TNF-α and IL-6. TNF-α in turn promotes chemoresistance by activating its downstream effector nuclear factor-κΒ (NF-κΒ) and then CXCL1 and CXCL2 [133]. Interestingly, TNF-α also has a protective effect toward BRAF-mutant melanoma cells, in which case TNF-α and NF-κΒ signaling enable cancer cells to bypass apoptosis induced by BRAF inhibitors [134]. Furthermore, TNF-α and IL-6 were found to elicit transition of tumor cells to a mesenchymal phenotype by modulating the expression of EMT-related transcription factors, such as Twist, Snail, or Slug [135–139]. Accordingly, plasma TNF-α was proposed to be suitable as a biomarker, when combined with TNM classification of malignant tumors, for predicting survival of head and neck squamous cell carcinoma and may be useful for designing treatment strategies [140].
Also, T cells are part of the reciprocal interactions between immune and tumor cells. Melanoma-specific cytotoxic T leukocytes (CTLs) secrete IFNγ, which in turn induces expression of PD-L1 by tumor cells. PD-L1 inhibits the function of the effector T cells by binding to its receptor PD1 [141, 142]. It is, however, possible to block the formation of the PD1/PD-L1 axis by treatment with immune checkpoint inhibitors and thereby restore a pro-inflammatory environment with tumor-specific CTLs [141, 143]. The possibility of reactivation of CTLs in the tumor microenvironment is one of the indications of the functional plasticity of the tumor immune component.
Additionally, alterations in metabolic pathways contribute to the interplay between tumor and immune cells. For instance, hypoxia was proposed to stimulate the expression of CCL28, followed by recruitment of regulatory T cells (Treg) stimulating tumor tolerance [144]. Hypoxia-induced release of VEGF by tumor cells also contributes to the recruitment of Treg cells [145], indicating that immune tolerance and angiogenesis are closely related mechanisms mediating immune evasion [146].
VEGF and Inflammation
To maintain the dynamic interactions between tumor and immune cells, there is an extensive vascular network. The complex organization of blood vessels within the tumor may allow mesenchymal tumor cells to escape and encourage metastatic spread. On the other hand, dense vasculature favors the recruitment of the immune cells to the tumor environment. VEGF is a key inducer of the tumor neovascularization and is abundantly produced by tumor cells [147]. VEGF release is regulated by VEGF receptor 2 (VEGFR2)-dependent activation of mTOR. This autocrine feed-forward loop intensifies the primary angiogenic signal and leads to the formation of new blood vessels in cancer [148]. This step is essential for tumor growth, further expansion and metastatic dissemination [1, 149]. Moreover, it was suggested that CXCL1/CXCR2 stimulates VEGF release through the activation of its downstream JAK/STAT3 signaling cascade [150]. The CXCL1/CXCR2 axis amplifies VEGF signaling and possibly rescues the proangiogenic phenotype upon VEGF inhibition. It was also revealed that activation of Src homology 2 domain-containing protein tyrosine phosphatase 2 (SHP-2) by Axl inhibits vascular endothelial growth factor receptor 2 (VEGFR2) during morphogenesis of endothelial cells [151]. Interestingly VEGFR2 is expressed not only on the surface of endothelial cells but also on macrophages, neutrophils, myeloid-derived suppressor cells (MDSCs), DCs and T cells [152–156]. Hence, it is not surprising that antiangiogenic therapy affects the immune function in the tumor microenvironment. Further, anti-VEGF therapy can reduce macrophage and MDSCs infiltration and increase mature DCs in the tumor suggesting that anti-VEGF therapy can function to restore a pro-inflammatory tumor microenvironment [157–159].
Concluding Remarks
Tumors are a complex network of dependencies between tumor cells, immune cells and the microenvironment. The immune contexture varies between tumors and may be associated with different overall prognosis, depending on the histological and molecular type of the tumor, stage and organ-specific microenvironment. There are different approaches to modulate immune response. Certain approaches, for instance immune checkpoint blockade or adaptive cell transfer, can result in long-term durable efficacy. However, multiple evolutionarily conserved programs active in the tumor microenvironment promote immune suppression. For example, the processes of efferocytosis and EMT function to promote local immune suppression, and these programs are co-opted by the tumor to evade immune surveillance. These pathways exploit signaling modules including those driven by the TAM receptors, PtdSer and EMT-inducing growth factors, and as a result these molecules or pathways are attractive as targets for prognostic exploration.
References
1.
Hanahan D, Weinberg RA. Hallmarks of cancer: the next generation. Cell. 2011;144(5):646–74.PubMed
2.
Fridman WH, Pages F, Sautes-Fridman C, Galon J. The immune contexture in human tumours: impact on clinical outcome. Nat Rev Cancer. 2012;12(4):298–306.PubMed
3.
Jacobs JF, Idema AJ, Bol KF, Grotenhuis JA, de Vries IJ, Wesseling P, et al. Prognostic significance and mechanism of Treg infiltration in human brain tumors. J Neuroimmunol. 2010;225(1–2):195–9.PubMed
4.
Fu J, Xu D, Liu Z, Shi M, Zhao P, Fu B, et al. Increased regulatory T cells correlate with CD8 T-cell impairment and poor survival in hepatocellular carcinoma patients. Gastroenterology. 2007;132(7):2328–39.PubMed
5.
Katou F, Ohtani H, Watanabe Y, Nakayama T, Yoshie O, Hashimoto K. Differing phenotypes between intraepithelial and stromal lymphocytes in early-stage tongue cancer. Cancer Res. 2007;67(23):11195–201.PubMed
6.
Schleypen JS, Von Geldern M, Weiss EH, Kotzias N, Rohrmann K, Schendel DJ, et al. Renal cell carcinoma-infiltrating natural killer cells express differential repertoires of activating and inhibitory receptors and are inhibited by specific HLA class I allotypes. Int J Cancer. 2003;106(6):905–12.PubMed
7.
Wong SC, Puaux AL, Chittezhath M, Shalova I, Kajiji TS, Wang X, et al. Macrophage polarization to a unique phenotype driven by B cells. Eur J Immunol. 2010;40(8):2296–307.PubMed
9.
Iglesia MD, Vincent BG, Parker JS, Hoadley KA, Carey LA, Perou CM, et al. Prognostic B-cell signatures using mRNA-seq in patients with subtype-specific breast and ovarian cancer. Clin Cancer Res. 2014;20(14):3818–29.PubMedPubMedCentral
10.
Lemke G, Burstyn-Cohen T. TAM receptors and the clearance of apoptotic cells. Ann N Y Acad Sci. 2010;1209:23–9.PubMedPubMedCentral
11.
Lemke G, Rothlin CV. Immunobiology of the TAM receptors. Nat Rev Immunol. 2008;8(5):327–36.PubMedPubMedCentral
12.
Rothlin CV, Ghosh S, Zuniga EI, Oldstone MB, Lemke G. TAM receptors are pleiotropic inhibitors of the innate immune response. Cell. 2007;131(6):1124–36.PubMed
13.
Bhattacharyya S, Zagorska A, Lew ED, Shrestha B, Rothlin CV, Naughton J, et al. Enveloped viruses disable innate immune responses in dendritic cells by direct activation of TAM receptors. Cell Host Microbe. 2013;14(2):136–47.PubMedPubMedCentral
14.
Gjerdrum C, Tiron C, Hoiby T, Stefansson I, Haugen H, Sandal T, et al. Axl is an essential epithelial-to-mesenchymal transition-induced regulator of breast cancer metastasis and patient survival. Proc Natl Acad Sci U S A. 2010;107(3):1124–9.PubMed
15.
Schlegel J, Sambade MJ, Sather S, Moschos SJ, Tan AC, Winges A, et al. MERTK receptor tyrosine kinase is a therapeutic target in melanoma. J Clin Invest. 2013;123(5):2257–67.PubMedPubMedCentral
16.
Meyer AS, Miller MA, Gertler FB, Lauffenburger DA. The receptor AXL diversifies EGFR signaling and limits the response to EGFR-targeted inhibitors in triple-negative breast cancer cells. Sci Signal. 2013;6(287):ra66.PubMedPubMedCentral
17.
Beutler B, Jiang Z, Georgel P, Crozat K, Croker B, Rutschmann S, et al. Genetic analysis of host resistance: Toll-like receptor signaling and immunity at large. Annu Rev Immunol. 2006;24:353–89.PubMed
18.
Honda K, Takaoka A, Taniguchi T. Type I interferon [corrected] gene induction by the interferon regulatory factor family of transcription factors. Immunity. 2006;25(3):349–60.PubMed
19.
Camenisch TD, Koller BH, Earp HS, Matsushima GK. A novel receptor tyrosine kinase, Mer, inhibits TNF-alpha production and lipopolysaccharide-induced endotoxic shock. J Immunol. 1999;162(6):3498–503.PubMed
20.
Mansell A, Smith R, Doyle SL, Gray P, Fenner JE, Crack PJ, et al. Suppressor of cytokine signaling 1 negatively regulates Toll-like receptor signaling by mediating Mal degradation. Nat Immunol. 2006;7(2):148–55.PubMed
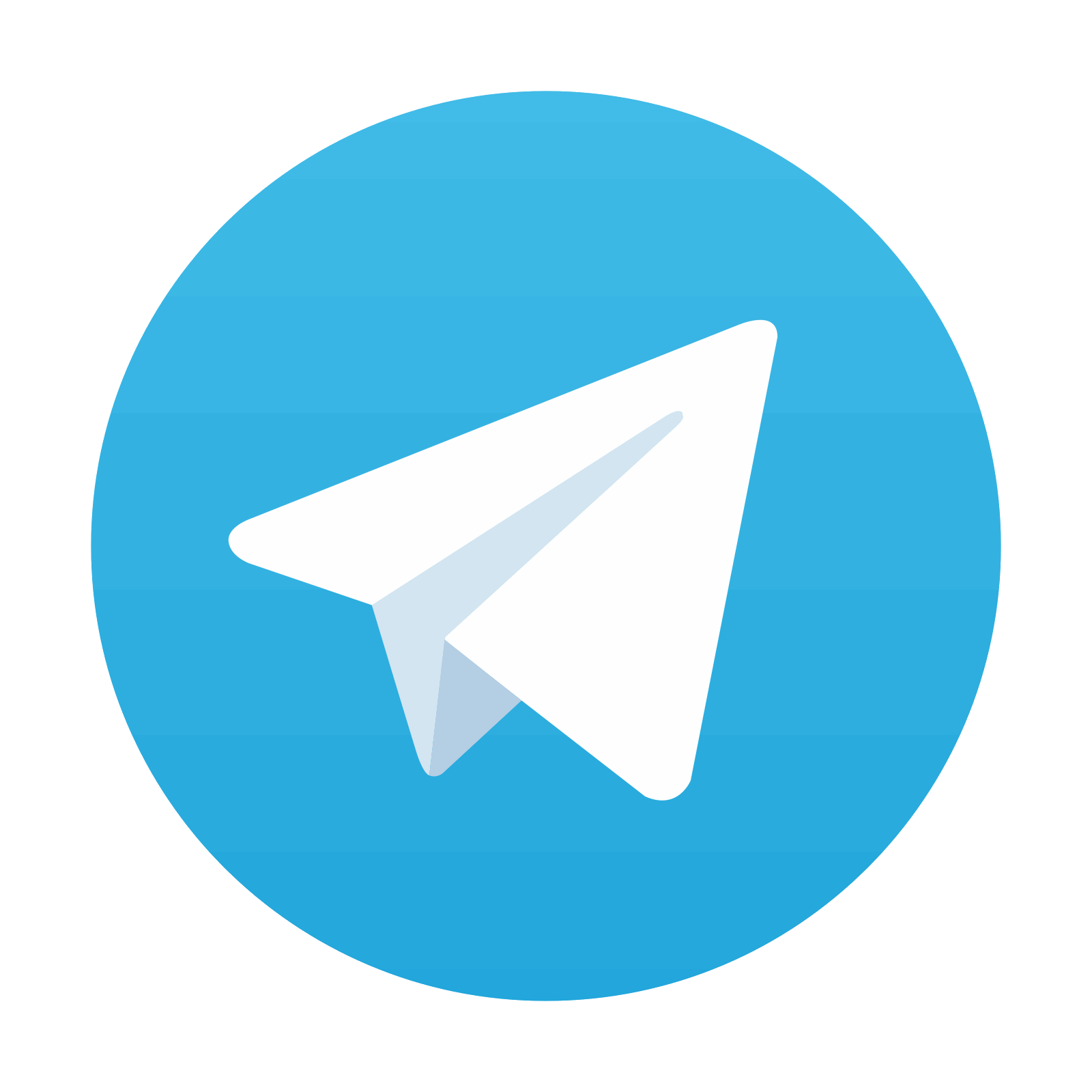
Stay updated, free articles. Join our Telegram channel

Full access? Get Clinical Tree
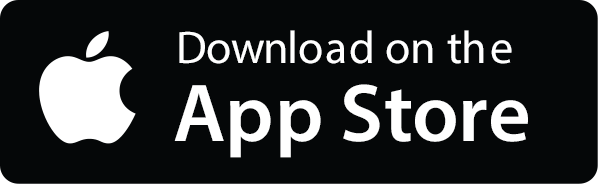
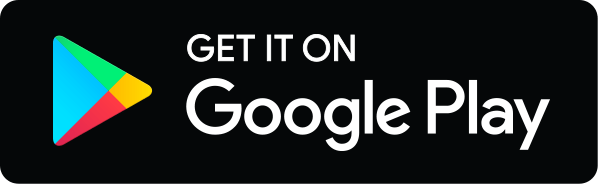