Water and sodium balance
Both the internal and external balance of any substance must be considered. The internal balance is the distribution between different body compartments, while the external balance matches input with output. The movements of Na+ and water that occur all the time between plasma and glomerular filtrate, or between plasma and gastrointestinal (GI) secretions, provide the potential for large losses, with consequent serious and rapid alterations in internal balance. For example, about 25 000 mmol of Na+ are filtered at the glomerulus over 24 h, normally with subsequent reabsorption of more than 99%. Likewise, 1000 of mmol Na+ enter the GI tract in various secretions each day, but less than 0.5% (5 mmol) is normally lost in the faeces.
Internal distribution of water and sodium
In a 70 kg adult, the total body water is about 42 L comprising about 28 L of intracellular fluid (ICF) and 14 L of extracellular fluid (ECF) water. The ECF water is distributed as 3 L of plasma water and 11 L of interstitial water. The total body Na+ is about 4200 mmol and is mainly extracellular–about 50% is in the ECF, 40% in bone and 10% in the ICF.
Two important factors influence the distribution of fluid between the ICF and the intravascular and extravascular compartments of the ECF:
Osmolality, osmolarity and tonicity
The osmolality is the number of solute particles per unit weight of water, irrespective of the size or nature of the particles. Therefore, a given weight of low molecular weight solutes contributes much more to the osmolality than the same weight of high molecular weight solutes. The units are mmol/kg of water. This determines the osmotic pressure exerted by a solution across a membrane. Most laboratories can measure plasma osmolality, but it is also possible to calculate the approximate osmolality of plasma using a number of formulae of varying complexity. The following formula has the benefit of being easy to calculate and performs as well as more complex versions (all concentrations must be in mmol/L):
This formula includes all the low molecular weight solutes contributing to plasma osmolality. Values for Na+ and K+ are doubled so as to allow for their associated anions, such as chloride. The formula is approximate and is not a complete substitute for direct measurement. Calculated osmolality is usually close to measured osmolality, but they may differ considerably for two different types of reason. First, there may be large amounts of unmeasured low molecular mass solutes (e.g. ethanol) present in plasma. These will contribute to the measured osmolality, but will obviously not be taken into account in the osmolality calculated from this formula. This will cause an ‘osmole gap’, with measured osmolality being greater than calculated osmolality. The other cause of a discrepancy is when there is a gross increase in plasma protein or lipid concentration, both of which decrease the plasma water per unit volume. This affects some methods of measurement of [Na+], giving an artefactually low result (‘pseudohyponatraemia’, see p. 23). This will result in an erroneously low calculated osmolality.
The osmolality of urine is usually measured directly, but is also linearly related to its specific gravity (which can be measured using urine dipsticks), unless there are significant amounts of glucose, protein or X-ray contrast media present.
The osmolarity is the number of particles of solute per litre of solution. Its units are mmol/L. Its measurement or calculation has been largely replaced by osmolality.
Tonicity is a term often confused with osmolality. However, it should only be used in relation to the osmotic pressure due to those solutes (e.g. Na+) that exert their effects across cell membranes, thereby causing movement of water into or out of the cells. Substances that can readily diffuse into cells down their concentration gradients (e.g. urea, alcohol) contribute to plasma osmolality but not to plasma tonicity, since after equilibration their concentration will be equal on both sides of the cell membrane. Tonicity is not readily measurable.
The tonicity of ICF and ECF equilibrate with one another by movement of water across cell membranes. An increase in ECF tonicity causes a reduction in ICF volume as water moves from the ICF to the ECF to equalise the tonicity of the two compartments, whereas a decrease in ECF tonicity causes an increase in ICF volume as water moves from the ECF to the ICF.
Colloid osmotic pressure (oncotic pressure)
The osmotic pressure exerted by plasma proteins across cell membranes is negligible compared with the osmotic pressure of a solution containing NaCl and other small molecules, since they are present in much lower molar concentrations. In contrast, small molecules diffuse freely across the capillary wall, and so are not osmotically active at this site, but plasma proteins do not readily do so. This means that plasma [protein] and hydrodynamic factors together determine the distribution of water and solutes across the capillary wall, and hence between the intravascular and interstitial compartments (Figure 2.1).
Regulation of external water balance
Typical daily intakes and outputs of water are given in Table 2.1. Water intake is largely a consequence of social habit and is very variable, but is also controlled by the sensation of thirst. Its output is controlled by the action of vasopressin, also known as antidiuretic hormone (ADH). In states of pure water deficiency, plasma tonicity increases, causing a sensation of thirst and stimulating vasopressin secretion, both mediated by hypothalamic osmoreceptors. Vasopressin then promotes water reab sorption in the distal nephron, with consequent production of small volumes of concentrated urine. Conversely, a large intake of water causes a fall in tonicity, suppresses thirst and reduces vasopressin secretion, leading to a diuresis, producing large volumes of dilute urine.
Figure 2.1 Movements of water and low molecular mass solutes across the capillary wall when the plasma [protein] is (a) normal and (b) low. The effects shown are: hydrostatic pressure, which drives water and low molecular mass solutes outwards and decreases along the length of the capillary; and plasma oncotic pressure, which attracts water and low molecular mass solutes inwards and is constant along the length of the capillary. The net movement of water and low molecular mass solutes across the capillary wall is governed by the net effect of hydrostatic and plasma oncotic pressures.
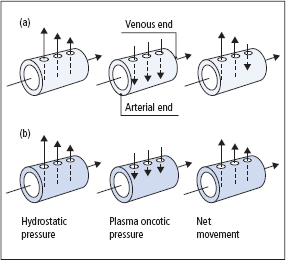
Table 2.1 Average daily water intake and output of a normal adult in the UK
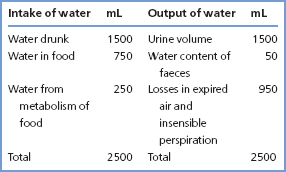
Secretion of vasopressin is normally controlled by small changes in ECF tonicity, but it is also under tonic inhibitory control from baroreceptors in the left atrium and great vessels on the left side of the heart. Where haemodynamic factors (e.g. excessive blood loss, heart failure) reduce the stretch on these receptors, often without an accompanying change in ECF tonicity, a reduction in tonic inhibitory control stimulates vasopressin secretion. The resulting water retention causes hyponatraemia and is relatively ineffective in expanding the intravascular compartment, since water diffuses freely throughout all compartments (Figure 2.2).
Regulation of external sodium balance
Dietary intakes of Na+ (and Cl–) are very variable worldwide. A typical ‘Western’ diet provides 100200 mmol of both Na+ and Cl– daily, but the total body Na+ can be maintained even if intake is less than 5 mmol or greater than 750 mmol daily. Urinary losses of Na+ normally closely match intake. There is normally little loss of these ions through the skin or in the faeces, but in disease the GI tract can become a major source of Na+ loss.
The amount of Na+ excreted in the urine controls the ECF volume since, when osmoregulation is normal, the amount of extracellular water is controlled to maintain a constant concentration of extracellular Na+. A number of mechanisms are important regulators of Na+ excretion:
- The renin–angiotensin–aldosterone system Renin is secreted in response to a fall in renal afferent arteriolar pressure or to a reduction in supply of Na+ to the distal tubule. It converts angiotensinogen in plasma to angiotensin I (AI), which in turn is converted to angiotensin II (AII) by angiotensin-converting enzyme (ACE). Both AII and its metabolic product angiotensin III (AIII) are pharmacologically active, and stimulate the release of aldosterone from the adrenal cortex. Aldosterone acts on the distal tubule to promote Na+ reabsorption in exchange for urinary loss of H+ or K+. Since Na+ cannot enter cells freely, its retention (with iso-osmotically associated water) contributes solely to ECF volume expansion, unlike pure water retention (Figures 2.2 and 2.3). Although the reninangiotensin-aldosterone system causes relatively slow responses to Na+ deprivation or Na+ loading, evidence suggests that this is the main regulatory mechanism for Na+ excretion.
- The glomerular filtration rate (GFR) The rate of Na+ excretion is often related to the GFR. When the GFR falls acutely, less Na+ is filtered and excreted, and vice versa. However, this only becomes a limiting factor in Na+ excretion at very low levels of GFR.
- Atrial natriuretic peptide (ANP) This peptide secreted by cardiocytes of the right atrium of the heart promotes Na+ excretion by the kidney, apparently by causing a marked increase in GFR. The importance of the ANP regulatory mechanism is not yet clear, but it probably only plays a minor role. Other structurally similar peptides have been identified, including brain or B-type natriuretic peptide (BNP), secreted by the cardiac ventricles and with similar properties to ANP. BNP is increasingly being used in the assessment of patients suspected of having cardiac failure (see p. 182).
Figure 2.2 Different effects on the body’s fluid compartments of fluid gains of 3 L of (a) water and (b) isotonic saline. The volumes shown relate to a 70 kg adult.
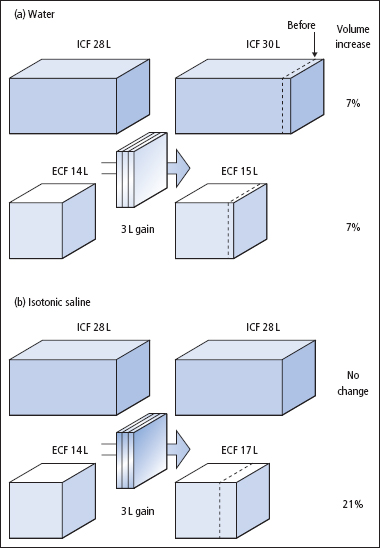
Figure 2.3 Different effects on the body’s fluid compartments of fluid losses of 3 L of (a) water and (b) isotonic saline. The volumes shown relate to a 70 kg adult.
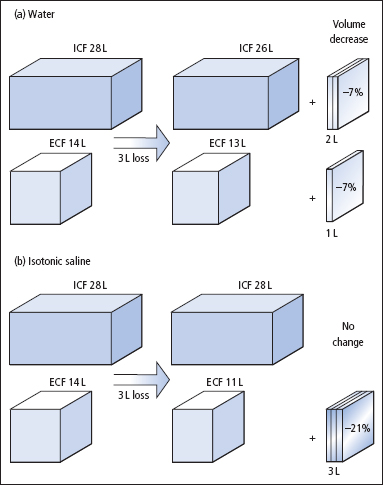
Disorders of water and sodium homeostasis
It is important to remember that the concentration of any substance is a consequence of the amount both of the solute (here Na+) and of the solvent (here water). The concentration of the solute may change because of changes in either the amount of solute, the amount of solvent, or both. Although the physiological control mechanisms for water and for Na+ are distinct, they need to be considered together when seeking an understanding of a patient’s Na+ and water balance, and of the plasma [Na+].
Whereas losses or gains of pure water are distributed across all fluid compartments, losses or gains of Na+ and water, as isotonic fluid, are borne by the much smaller ECF compartment (Figures 2.2 and 2.3). Thus, it is usually more urgent to replace losses of isotonic fluid than losses of water. For the same reason, circulatory overload is more likely with excessive administration of isotonic Na+– containing solutions than with isotonic dextrose (the dextrose is metabolised, leaving water behind).
Plasma [Na+] cannot be used as a simple measure of body Na+ status since it is very often abnormal as a result of losses or gains of water rather than of Na+. The plasma [Na+] must be interpreted in relation to the patient’s history and the findings on clinical examination, and if necessary backed up by other investigations.
Table 2.2 Causes of depletion of and excess water
Categories | Examples |
Depletion of water | |
Inadequate intake | Infants, patients in coma or who are very sick, or have symptoms such as nausea or dysphagia |
Abnormal losses via | |
Lungs | Inadequate humidification in mechanical ventilation |
Skin | Fevers and in hot climates |
Renal tract | Diabetes insipidus, lithium therapy |
Excess water | |
Excessive intake | |
Oral | Psychogenic polydipsia |
Parenteral | Hypotonic infusions after operations |
Renal retention | Excess vasopressin (SIADH, Table 2.5), hypoadrenalism, hypothyroidism |
The main causes of depletion and excess of water are summarised in Table 2.2, and of Na+ in Table 2.3. Although some of these conditions may be associated with abnormal plasma [Na+], it must be emphasised that this is not necessarily always the case. For example, patients with acute losses of isotonic fluid (e.g. plasma, ECF, blood) may be severely and dangerously hypovolaemic and Na’ depleted, and very possibly in shock, but their plasma [Na+] may nevertheless be normal or even raised.
Hyponatraemia
Hyponatraemia is the most common clinical biochemical abnormality. Most patients with hyponatraemia also have a low plasma osmolality. Unless an unusual cause of hyponatraemia is suspected (see ‘Other causes of hyponatraemia’, p. 23), measurement of plasma osmolality contributes little or no extra information.
Patients with hyponatraemia can be divided into three categories, on the basis of the ECF volume being low, normal or increased. These categories in turn reflect a total body Na+ that is low, normal or increased, respectively. The value of this classification is two-fold. First, the clinical history and examination often indicate the ECF volume and therefore the total body Na+ status. Secondly, treatment often depends on the total body Na+ status rather than the [Na+]. One possible way of narrowing the differential diagnosis of a patient with hyponatraemia, based on this subdivision, is shown in Figure 2.4.
Table 2.3 Causes of depletion of and excess sodium
Categories | Examples |
Depletion of sodium | |
Inadequate oral intake | Rare, by itself |
Abnormal losses via | |
Skin | Excessive sweating, dermatitis, burns |
GI tract | Vomiting, aspiration, diarrhoea, fistula, paralytic ileus, blood loss |
Renal tract | Diuretic therapy, osmotic diuresis, renal tubular disease, mineralocorticoid deficiency |
Excess of sodium | |
Excessive intake | |
Oral | Sea water (drowning), salt tablets, hypertonic NaCl administration (this is rare) |
Parenteral | Post-operatively, infusion of hypertonic NaCl |
Renal retention | Acute and chronic renal failure, primary and secondary hyperaldosteronism, Cushing’s syndrome |
Hyponatraemia with low ECF volume
The patient has lost Na+ and water in one or more body fluids (e.g. GI tract secretions, urine, inflammatory exudate) or may have been treated with a diuretic (Table 2.4). The low ECF volume leads to tachycardia, orthostatic hypotension, reduced skin turgor and oliguria. The hypovolaemia causes secondary aldosteronism with a low urinary [Na+] (usually <20 mmol/L), unless diuretic treatment is the cause when urinary [Na+] remains high. The hypovolaemia also provides a ‘volume stimulus’ to vasopressin secretion, resulting in oliguria and a concentrated urine. The consequent water retention can further contribute to the hyponatraemia.
Treatment requires administration of isotonic saline.
Figure 2.4 Schematic diagram to assist in the diagnosis of some of the more common causes of hyponatraemia. In practice more than one cause may be present, and the findings may be influenced by the recent clinical history and oral or IV fluid intake. (CCF = congestive cardiac failure; CRF = chronic renal failure; GI = gastrointestinal; SIADH, syndrome of inappropriate secretion of antidiuretic hormone.)
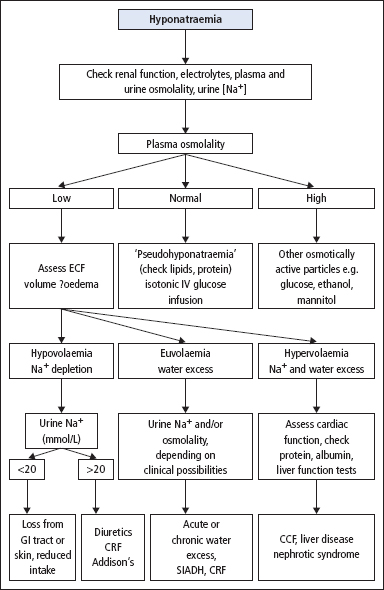
Hyponatraemia with normal ECF volume
The hyponatraemia results from excessive water retention, due to inability to excrete a water load. This may develop acutely, or it may be chronic (Table 2.4).
- Acute water retention Plasma [vasopressin] is acutely increased after trauma or major surgery, as part of the metabolic response to trauma, and during delivery and postpartum. Administration of excessive amounts of water (e.g. as 5% dextrose) in these circumstances may exacerbate the hyponatraemia and cause acute water intoxication.
- Chronic water retention Perhaps the most widely known chronic ‘cause’ of this form of hyponatraemia is the syndrome of inappropriate secretion of ADH (SIADH) (Table 2.5). Whether this concept is of value in understanding its aetiology, or valid in terms of altered physiology, is uncertain. As the name implies, ADH (or rather vasopressin) is being secreted in the absence of an ‘appropriate’ physiological stimulus, of either fluid depletion or hypernatraemia. As water is retained, the potential for expansion of the ECF volume is limited by a reduction in renin and an increase in sodium excretion. A new steady state is achieved, with essentially normal, or only mildly increased, ECF volume. If the causative disorder (Table 2.5) is transient, plasma [Na+] returns to normal when the primary disorder (e.g. pneumonia) is treated. However, in patients with cancer, the hyponatraemia is presumably due to production of vasopressin or a related substance by the tumour, and is usually persistent. If symptoms are mild, they may be treated by severe fluid restriction (i.e. ≤500 mL/ day), but if this is ineffective, treatment with a drug that antagonises the renal effects of vasopressin (e.g. demeclocycline) may be tried.
Table 2.4 Causes of hyponatraemia
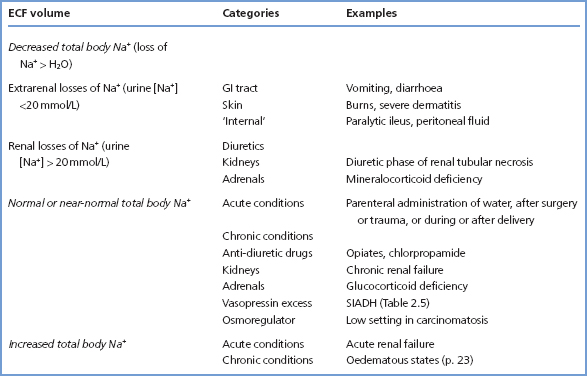
Table 2.5 SIADH
Characteristics of the syndrome | Causes (and examples) |
Low plasma [Na+] and osmolality | Malignant disease of the bronchus, prostate, pancreas, etc. |
Inappropriately high | Chest diseases, e.g. |
urine osmolality | pneumonia, bronchitis, tuberculosis |
Excessive renal excretion of Na+ | Central nervous system (CNS) diseases, including brain trauma, tumours, meningitis |
No evidence of volume depletion |