Disorders of Potassium and Magnesium Homeostasis
KEY CONCEPTS
Potassium regulates many biochemical processes in the body and is a key cation for electrical action potentials across cellular membranes.
In patients with concomitant hypokalemia and hypomagnesemia, it is imperative to correct the hypomagnesemia before the hypokalemia.
Potassium chloride is the preferred potassium supplement for the most common causes of hypokalemia.
Hyperkalemia is a common occurrence in patients with acute or chronic kidney disease.
Hypomagnesemia is commonly caused by excessive GI or renal magnesium wasting.
Hypermagnesemia is predominantly observed in patients with acute or chronic kidney disease.
Potassium and magnesium are electrolytes that are responsible for numerous metabolic activities. Disorders of these electrolytes are frequently seen in both the acute care and community ambulatory care settings. Therefore, clinicians need a firm understanding of the etiology, pathophysiology, symptoms, pharmacotherapy, and monitoring of these disorders. This chapter describes the homeostatic mechanisms that are responsible for the maintenance of normal potassium and magnesium serum concentrations. The clinical disorders responsible for the development of hyperkalemia, hypermagnesemia, hypokalemia, and hypomagnesemia are also reviewed.
POTASSIUM
Potassium is the most abundant cation in the body, with estimated total-body stores of 3,000 to 4,000 mEq (3,000 to 4,000 mmol).1 Ninety-eight percent of this amount is contained within the intracellular compartment, and the remaining 2% is distributed within the extracellular compartment. The sodium-potassium adenosine triphosphatase (Na+-K+-ATPase) pump located in the cell membrane is responsible for the compartmentalization of potassium. This pump is an active transport system that maintains increased intracellular stores of potassium by transporting sodium out of the cell and potassium into the cell at a ratio of 3:2. Consequently, the pump maintains a higher concentration of potassium inside the cell.
The normal serum concentration range for potassium is 3.5 to 5 mEq/L (3.5 to 5 mmol/L), whereas the intracellular potassium concentration is usually approximately 150 mEq/L (150 mmol/L).2 Approximately 75% of the intracellular potassium is located in skeletal muscle; the remaining 25% is located in the liver and red blood cells. Extracellular potassium is distributed throughout the serum and interstitial space. Potassium is dynamic in that it is constantly moving between the intracellular and extracellular compartments according to the body’s needs. Thus, the serum potassium concentration alone does not accurately reflect the total-body potassium content.
Potassium has many physiologic functions within cells, including protein and glycogen synthesis and cellular metabolism and growth. It is also a determinant of the electrical action potential across the cell membrane.1 The ratio of the intracellular-to-extracellular potassium concentration is the major determinant of the resting membrane potential across the cell membrane. Thus, the resting membrane potential is greatly affected by variations in extracellular potassium concentration. Serum potassium concentrations outside the normal range can have disastrous effects on neuromuscular activity, in particular cardiac conduction. Hypo- and hyperkalemia are both associated with potentially fatal cardiac arrhythmias, along with other neuromuscular disturbances. Finally, potassium is integral to maintaining healthy blood pressure balance, prevention of stroke, and potentially other cardiovascular diseases.3 Both the National High Blood Pressure Education Program and the Institute of Medicine recommend potassium supplementation as strategies for preventing and treating hypertension.4–6
Control of Potassium Homeostasis
Potassium homeostasis, the maintenance of serum potassium within the normal range, is affected by dietary intake, GI and urinary excretion, hormones, acid-base balance, body fluid tonicity, and a highly integrated feedback mechanism.7,8 The recommended daily allowance for dietary potassium intake in the United States is approximately 50 mEq/day (50 mmol/day); however, the Seventh Report of the Joint National Committee on Prevention, Detection, Evaluation, and Treatment of High Blood Pressure guidelines recommends a daily intake of 100 mEq (100 mmol) for prevention of hypertension and other cardiovascular complications.9 Potassium is found in abundance in fruits, vegetables, and meats. The typical American ingests approximately 50 to 150 mEq (50 to 150 mmol) of potassium daily. Nearly all of this is absorbed, with only 10 to 20 mEq/day (10 to 20 mmol/day) eliminated in feces. The amount eliminated in the feces increases, however, in patients with diarrhea and in those with chronic kidney disease (CKD).8
The kidney is the primary route of potassium elimination. Potassium is freely filtered, but almost all of it is reabsorbed passively in the proximal tubule and the thick ascending limb of the loop of Henle.10 Therefore, urinary potassium excretion is primarily determined by potassium secretion from the luminal cells of the distal tubule and collecting duct. Although the amount of potassium filtered by the glomerulus approaches 700 mEq (700 mmol) per day, only approximately 10% to 20% is actually excreted in the urine.10 However, this amount can vary based on dietary intake, serum potassium concentration, and aldosterone activity. For example, more potassium is renally excreted in conditions that result in high aldosterone activity (e.g., dehydration) when the body is attempting to conserve sodium or when there is an increase in dietary potassium intake.
Hormones such as insulin, catecholamines, and aldosterone dramatically affect potassium homeostasis. Insulin is the most important hormonal mediator of potassium balance because it stimulates the cellular Na+-K+-ATPase pump to increase transport of potassium into liver, muscle, and adipose tissue.7 There is a complex negative feedback loop in which insulin secretion tightly regulates serum potassium concentrations: an increase of only a few tenths of a milliequivalent (mmol) of potassium stimulates pancreatic insulin secretion in an attempt to prevent hyperkalemia from developing.1 If hyperkalemia does occur, glucagon is released from the liver to protect against insulin-induced hypoglycemia. Conversely, hypokalemia inhibits insulin secretion, a finding that explains why some patients receiving diuretics develop hyperglycemia.
An elevation in circulating catecholamines such as epinephrine usually results in the intracellular movement of potassium by two mechanisms.10 They stimulate the β-receptor, which directly activates the Na+K+-AT Pase pump. Second, they stimulate glycogenolysis, which raises blood glucose concentrations, thereby increasing insulin secretion. This dual mechanism is often used therapeutically in patients with hyperkalemia to normalize serum potassium concentrations.
Aldosterone, a mineralocorticoid that is secreted from the adrenal glands in response to high serum potassium concentrations, promotes urinary potassium excretion. Aldosterone works in the distal tubule and collecting duct to promote the reabsorption of sodium and water in exchange for potassium. Aldosterone may also have extrarenal activity by stimulating cellular Na+-K+-ATPase pump activity.10
Changes in acid-base status significantly affect the serum potassium concentration. For example, the infusion of metabolic inorganic acids, such as hydrochloric acid, results in an increase in serum potassium. The body compensates for excessive hydrogen ions by moving them from the serum into the cell in exchange for intracellular potassium, to maintain electroneutrality. The processes by which this occurs are highly complex, and involve cellular H+-K+-ATPase pumps and both Na+-HCO3– and K+-HCO3– cotransporters.11 The efflux of potassium into the serum can result in hyperkalemia. A commonly quoted approximation of the pH effect is that for every 0.1 unit decrease in pH, there is a corresponding increase in serum potassium of 0.6 to 0.8 mEq/L (0.6 to 0.8 mmol/L) (with a wide range of 0.2 to 1.7).6 This is often referred to as false hyperkalemia because there is not a true excess of total-body potassium. Metabolic acidosis associated with lactic acidosis and ketoacidosis does not result in hyperkalemia, because both cations and anions enter the cell, thus maintaining electroneutrality.1 Respiratory acidosis also does not significantly affect the serum potassium concentration.
Conversely, metabolic alkalosis has been associated with hypokalemia. As a result of a net loss of hydrogen ion from the serum, intracellular hydrogen ions enter the serum to increase the acidity of the blood. To maintain electroneutrality, extracellular potassium ions are shifted intracellularly. This creates a relative deficiency of potassium in the serum. Serum potassium decreases approximately 0.6 mEq/L (0.6 mmol/L) for each 0.1 unit increase in blood pH. This is frequently termed false hypokalemia because there is not a true deficiency in total-body potassium.
Finally, hyperosmolality can result in enhanced movement of potassium from the cell into the extracellular fluid. This occurs most likely because of the associated cell shrinkage and water loss, which increases the intracellular-to-extracellular potassium gradient.4 This is seen most commonly in conditions such as diabetic ketoacidosis. Conversely, hypoosmolality does not seem to affect potassium distribution.
HYPOKALEMIA
Epidemiology
Hypokalemia (defined as a serum potassium concentration <3.5 mEq/L [<3.5 mmol/L]) is a commonly encountered electrolyte abnormality in clinical practice. Hypokalemia can be categorized as mild (serum potassium 3.1 to 3.5 mEq/L [3.1 to 3.5 mmol/L]), moderate (serum potassium 2.5 to 3 mEq/L [2.5 to 3 mmol/L]), or severe (<2.5 mEq/L [<2.5 mmol/L]).12 When hypokalemia is detected, a diagnostic workup that evaluates the patient’s comorbid disease states and concomitant medications should be initiated. Hypokalemia is virtually nonexistent in healthy adults. This is due in part to the relatively high potassium content in the typical Western diet as well as the body’s effective potassium-sparing mechanisms, which tightly regulate the serum potassium concentration. However it has been estimated that as many as 50% of patients who receive thiazide or loop diuretics have serum potassium concentrations less than 3.5 mEq/L (3.5 mmol/L).13
While hypokalemia may be thought of as merely a laboratory abnormality, there are serious potential consequences associated with persistent hypokalemia. Recent data suggest that hypokalemia increases mortality in patients with chronic heart failure and in those with CKD, a population typically thought to be more sensitive to the effects of hyperkalemia.14 In fact, even mild hypokalemia in patients with CKD appears to confer a greater risk of dying compared with those with mild to moderate hyperkalemia.15
Etiology and Pathophysiology
Hypokalemia results when there is a total-body potassium deficit, or when serum potassium is shifted into the intracellular compartment. Total-body deficits occur in the setting of poor dietary intake of potassium, or when there are excessive renal and GI losses of potassium. Maintaining a consistent dietary intake of potassium is important because the body has no effective method for storing potassium. At steady state, potassium excretion matches potassium intake; approximately 90% of ingested potassium is renally excreted, whereas 10% is excreted in feces.10 This underscores the importance of eating a well-balanced diet. Elderly patients with chronic diseases and those undergoing surgery are at increased risk for developing hypokalemia because of insufficient intake or losses resulting from surgery.
Many drugs can cause hypokalemia by a variety of mechanisms including intracellular potassium shifting and increased renal or stool losses (Table 36-1). The most common cause of drug-induced hypokalemia is loop and thiazide diuretic administration as these agents inhibit renal sodium reabsorption, which results in increased sodium delivery to the distal tubule. Consequently, hypokalemia develops because the distal tubule selectively reabsorbs sodium, and excretes potassium down its concentration gradient. Second, because diuretics result in volume contraction, aldosterone is secreted that further promotes the renal excretion of potassium. If concomitant potassium supplements are not provided to patients receiving loop and thiazide diuretics, mild to moderate hypokalemia is inevitable.
TABLE 36-1 Mechanism of Drug-Induced Hypokalemia
The second most common etiology of hypokalemia is excessive loss of potassium-rich GI fluid as a result of diarrhea and/or vomiting. The typical potassium loss in feces is approximately 10 mEq (10 mmol) per day.8 In diarrheal states, this amount increases proportionally with the volume of stool output. Vomiting also accounts for substantial potassium losses, which have been estimated to be as high as 30 to 50 mEq (30 to 50 mmol) per liter of vomitus.13 Metabolic alkalosis can also occur in cases of severe diarrhea and vomiting as a result of loss of these bicarbonate-rich fluids. This causes an intracellular shifting of potassium, which lowers the serum concentration of potassium even further. Prolonged diarrhea and vomiting can significantly affect children and elderly patients because their kidneys are unable to effectively maintain adequate fluid status.
Hypomagnesemia, which is present in more than 50% of cases of clinically significant hypokalemia, contributes to the development of hypokalemia because it reduces the intracellular potassium concentration and promotes renal potassium wasting.16 While the precise mechanism of the accelerated renal loss is unknown, many believe that the intracellular potassium concentration may decrease because hypomagnesemia impairs the function of the Na+-K+-ATPase pump thereby promoting K+ wasting. Alternatively, the combination of increased sodium delivery to the distal tubule, elevated aldosterone concentrations, and hypomagnesemia may cause the renal outer medullary potassium channels to excrete potassium.16 What is clear is that hypokalemia and hypomagnesemia often coexist as a result of drugs (diuretic administration) or disease states (diarrhea). When concomitant hypokalemia and hypomagnesemia occur, the magnesium deficiency should be corrected first, otherwise full repletion of the potassium deficit is difficult.
CLINICAL PRESENTATION Hypokalemia
TREATMENT
Desired Outcome
The goals of hypokalemia management are to prevent and/or treat serious life-threatening complications, normalize the serum potassium concentration, identify and correct the underlying cause of hypokalemia, and finally prevent overcorrection of the serum potassium concentration.
General Approach to Therapy
The general approach to therapy depends on the degree and rapidity with which hypokalemia developed and the presence of symptoms. Serum potassium concentrations between 3.5 and 4 mEq/L (3.5 and 4 mmol/L) are a sign of early potassium depletion. No pharmacologic therapy is recommended at this point; however, these patients should be encouraged to increase their dietary intake of potassium-rich foods. When the serum potassium concentration is between 3 and 3.5 mEq/L (3 and 3.5 mmol/L), the patient’s concomitant conditions and therapies will largely determine whether pharmacologic therapy should be initiated. Oral potassium supplementation should be initiated in patients with underlying cardiac conditions that predispose them to cardiac arrhythmias. This includes patients receiving concomitant digoxin therapy. Patients with serum potassium concentrations below 3 mEq/L (3 mmol/L) should always be treated to achieve values between 4 and 4.5 mEq/L (4 and 4.5 mmol/L). In asymptomatic patients, oral therapy is the preferred route of administration. IV potassium can be necessary in symptomatic patients with severe depletion, or in patients who are intolerant to oral supplementation. In patients with concomitant moderate to severe hypomagnesemia, the magnesium deficit should be corrected before potassium supplementation.10,12
Nonpharmacologic Therapy
The best and most abundant source of potassium supplementation comes from dietary sources, in particular, fresh fruits and vegetables, fruit juices, and meats. Table 36-2 lists foods that are excellent sources of potassium. Salt substitutes that contain potassium chloride are another effective, inexpensive source of potassium. Increased dietary intake of foods with high potassium content, however, is not recommended long term because it can add unwanted calories to the patient’s diet. Moreover, dietary potassium is almost entirely coupled with phosphate, rather than chloride, so it is not as effective in correcting potassium loss associated with hypochloremic conditions such as vomiting, nasogastric suctioning, and diuretic therapy.
TABLE 36-2 Foods that are High in Potassium
Pharmacologic Therapy
Formal guidelines for potassium supplementation were last published by the National Council on Potassium in Clinical Practice in 2000 (Table 36-3).17 These guidelines provided a comprehensive framework for potassium administration as a prophylactic and therapeutic replacement in many distinct patient populations. When deciding how to design the optimal regimen, one must consider: (a) the patient’s normal baseline potassium concentration; (b) underlying medical conditions that can affect potassium balance; (c) concomitant medications that can affect potassium balance; (d) the patient’s dietary and salt intake; and (e) the patient’s ability to comply with the therapeutic regimen.17
TABLE 36-3 General Consensus Guidelines for Potassium Replacement
A general rule for potassium replacement is that for every 1 mEq/L (1 mmol/L) decrease of potassium below 3.5 mEq/L (3.5 mmol/L), there is a corresponding total-body potassium deficit of 100 to 400 mEq (100 to 400 mmol). Because of the wide variance in projected deficits, each patient’s therapy must be individualized and adjustments made on the basis of the patient’s signs, symptoms, and frequent measurements of serum potassium. In patients receiving chronic loop or thiazide diuretic therapy, 40 to 100 mEq (40 to 100 mmol) of oral potassium supplementation can correct mild to moderate potassium deficits. Doses up to 120 mEq (120 mmol) can be required in more severe deficiencies. When providing oral potassium supplementation, the total daily dose should be divided into three to four doses to minimize the development of GI side effects. Patients receiving diuretics can become chronically hypokalemic and can benefit from combination potassium-sparing diuretic therapy.
Whenever possible, potassium supplementation should be administered by mouth. Three salts are available for oral potassium supplementation: chloride, phosphate, and bicarbonate. Potassium phosphate should be used when patients are both hypokalemic and hypophosphatemic; potassium bicarbonate is most commonly used when potassium depletion occurs in the setting of metabolic acidosis. Potassium chloride, however, is the primary salt form used because it is the most effective treatment for the most common causes of potassium depletion (i.e., diuretic-induced and diarrhea-induced) as these conditions are associated with potassium and chloride losses.
Potassium chloride can be administered in either tablet or liquid formulations (Table 36-4). The liquid forms are generally less expensive; however, patient compliance can be low because of their strong, unpleasant taste. Two sustained-release solid dosage forms are currently available in the United States: a wax-matrix formulation, and a microencapsulated formulation. The microencapsulated tablet is generally preferred because it disintegrates better in the stomach and is associated with less GI irritation. IV potassium use should be limited to: (a) severe cases of hypokalemia (serum concentration <2.5 mEq/L [<2.5 mmol/L]); (b) patients exhibiting signs and symptoms of hypokalemia such as electrocardiogram (ECG) changes or muscle spasms; or (c) patients unable to tolerate oral therapy. IV supplementation is more dangerous than oral therapy because it is more likely to result in hyperkalemia, phlebitis, and pain at the site of infusion.
TABLE 36-4 Differentiation of Available Potassium Supplements
The vehicle in which IV potassium is administered is important. Whenever possible, potassium should be prepared in saline-containing solutions (e.g., 0.9% or 0.45% sodium chloride [NaCl]). Dextrose-containing solutions stimulate insulin secretion, which can cause intracellular shifting of potassium, worsening the patient’s hypokalemia, and should be avoided whenever possible. Generally, 10 to 20 mEq (10 to 20 mmol) of potassium is diluted in 100 mL 0.9% NaCl for IV administration. These concentrations are safe when administered through a peripheral vein over 1 hour. When infusion rates exceed 10 mEq/h (10 mmol/h) ECG monitoring should be performed to detect cardiac changes. The serum potassium concentration should be evaluated following the infusion of each 30 to 40 mEq (30 to 40 mmol) to direct further potassium replacement requirements. Multiple doses of potassium can be repeated as needed until the serum potassium concentration normalizes. To allow adequate time for the potassium to equilibrate between the intra- and extracellular spaces, the clinician should wait at least 30 minutes from the end of each infusion before obtaining a serum concentration. Care should be taken to avoid sampling from the same line in which the potassium was infused, as this can result in a spuriously high potassium concentration.
In cases of severe potassium depletion, patients can require as much as 300 to 400 mEq/day (300 to 400 mmol/day). In this instance, it is common practice to dilute 40 to 60 mEq (40 to 60 mmol) in 1,000 mL 0.45% NaCl and infuse at a rate not exceeding 40 mEq/h (40 mmol/h). This should be performed in an intensive care unit under continuous ECG monitoring. Because of the high potassium concentration, and the risk for burning pain and peripheral venous sclerosis, the infusion should be through a central venous catheter into a large vein (e.g., superior vena cava) but care must be taken not to place the tip of the catheter into the right atrium.18 Directly delivering high potassium concentrations into the heart can result in cardiac arrhythmias. Given the volume required to infuse this dose of potassium, this infusion strategy might be impractical in certain clinical situations (e.g., patients requiring fluid restriction). A reasonable approach is to split the potassium dose between the oral and IV routes. For example, if a symptomatic patient requires 120 mEq (120 mmol) of potassium, the clinician can give 60 mEq (60 mmol) as the immediate-release potassium liquid, and the other 60 mEq (60 mmol) can be given through the IV route (20 mEq/100 mL/h [20 mmol/100 mL/h] in three doses). When giving large potassium doses, serum monitoring should be performed following the administration of half the dose to guide the clinician as to the need for additional potassium. This can also help avoid the development of hyperkalemia.
In the rare circumstances when cardiac arrest from hypokalemia is imminent, IV bolus dosing of potassium 10 mEq (10 mmol) over 5 minutes can be initiated and repeated once, if necessary.18
Alternative Therapies
Potassium-sparing diuretics are an alternative to chronic exogenous potassium supplementation, especially when patients are concomitantly receiving drugs that are known to deplete potassium (e.g., diuretics or amphotericin B). Spironolactone inhibits the effect of aldosterone in the distal convoluted tubule, thereby decreasing potassium elimination in the urine. Spironolactone is especially effective as a potassium-sparing agent in patients with primary or secondary hyperaldosteronism. Amiloride and triamterene act by an aldosterone-independent mechanism; however, the precise mechanism of their potassium sparing is unknown.
Spironolactone is available as 25-, 50-, and 100-mg tablets. The usual starting dose is 25 to 50 mg daily, and can be titrated to a maximum dose of 400 mg/day. The potassium-retaining effects generally take approximately 48 hours to occur. Important side effects include hyperkalemia, gynecomastia, breast tenderness, and impotence in men. Triamterene is available as 50- and 100-mg capsules. The usual starting dose is 50 mg twice daily, which can be titrated to 100 mg twice daily. Triamterene 50 mg is available as a combination product with hydrochlorothiazide 25 mg and is commonly used for the treatment of hypertension. Common side effects include hyperkalemia, sodium depletion, and metabolic acidosis. Amiloride is available as a 5-mg tablet. The usual starting dose is 5 mg daily; however, 10 mg can be given in those with severe hypokalemia. This is also available as a combination product with hydrochlorothiazide 50 mg. The most common side effects are hyperkalemia and metabolic acidosis.
Concomitant use of potassium supplementation with potassium-sparing diuretics is not necessary. There is a significant risk of hyperkalemia during combination therapy, especially in patients with underlying renal insufficiency or diabetes mellitus.
Evaluation of Therapeutic Outcomes
Serum potassium concentrations should be monitored regularly while the patient is receiving potassium supplementation. For patients receiving prophylactic potassium supplementation during diuretic therapy, the serum potassium and magnesium concentrations, as well as renal function should be monitored every 1 to 2 months in stable patients. In hospitalized patients receiving oral therapy for mild hypokalemia, the potassium concentration should be monitored every 2 to 3 days. Generally, the potassium concentration begins to increase within 72 hours. If it does not increase by at least 1 mEq/L (1 mmol/L) within 96 hours, the clinician should suspect concomitant magnesium depletion. Patients receiving IV potassium supplementation require close ECG monitoring if the infusion rate is greater than 20 mEq/h (20 mmol/h): doses greater than this should be administered only in the presence of continuous ECG monitoring. Additionally, the patient should have potassium concentrations obtained halfway through, and 30 minutes following completion of the total potassium dose to guide further potassium dosing. Finally, the patient should be assessed for adverse effects such as pain at the infusion site or phlebitis.
Clinical Bottom Line
Hypokalemia is a frequent medical condition caused by both biological processes as well as drug therapy. While mild hypokalemia is frequently asymptomatic, severe hypokalemia can cause fatal cardiac dysrhythmias, particularly in patients receiving concomitant medications such as digoxin. Patients receiving drugs that cause potassium wasting, e.g., thiazide or loop diuretics, should be closely followed for the development of hypokalemia and appropriate potassium supplementation should be started when necessary. Generally oral potassium is sufficient for mild hypokalemia; IV potassium is reserved for severe deficiency, and its use should be monitored closely.
HYPERKALEMIA
Hyperkalemia, defined as a serum potassium concentration greater than 5 mEq/L (5 mmol/L), can be further classified according to its severity: mild hyperkalemia (5.1 to 5.9 mEq/L [5.1 to 5.9 mmol/L]), moderate hyperkalemia (6 to 7 mEq/L [6 to 7 mmol/L]), and severe hyperkalemia (above 7 mEq/L [7 mmol/L]).17
Epidemiology
Hyperkalemia is much less common than hypokalemia. In fact, if all patients with acute and chronic kidney disease were excluded, the true prevalence of hyperkalemia would be insignificant. The incidence of hyperkalemia in hospitalized patients is highly variable, and reports have ranged from 1.4% to 10%.17 Most cases of hyperkalemia are the result of overcorrection of hypokalemia with IV potassium supplements. Severe hyperkalemia occurs more commonly in elderly patients with renal insufficiency who receive chronic oral potassium supplementation.
Etiology and Pathophysiology
Hyperkalemia develops when potassium intake exceeds excretion (true hyperkalemia) (i.e., elevated total-body stores), or when the transcellular distribution of potassium is disturbed (i.e., normal total-body stores). The four primary causes of hyperkalemia—(a) increased potassium intake, (b) decreased potassium excretion, (c) tubular unresponsiveness to aldosterone, and (d) redistribution of potassium into the extracellular space—are discussed below.
Hyperkalemia Associated with Increased Potassium Intake
Hyperkalemia in this setting is almost always associated with renal insufficiency. Patients with stage 4 or 5 CKD and dialysis patients who are noncompliant with dietary potassium restrictions often present with life-threatening hyperkalemia. Many of these patients do not realize that fresh fruits and vegetables contain large amounts of potassium. Anecdotally, in many dialysis centers the incidence of hyperkalemia peaks during the summer months, when fresh garden produce is available. Another common dietary source associated with the development of hyperkalemia is potassium chloride salt substitutes. Many dialysis patients are instructed to use salt substitutes to avoid excessive sodium intake in an attempt to control volume overload. These patients unwittingly become hyperkalemic because these products contain approximately 10 to 15 mEq (10 to 15 mmol) potassium per gram, or 200 mEq (200 mmol) per tablespoon. Finally, many over-the-counter herbal and alternative medicine products may contain significant concentrations of potassium. It is essential for patients with CKD to receive education regarding dietary sources of potassium as well as information on the potassium content of herbal products because the ingestion of these can lead to hyperkalemia.
Hyperkalemia Associated with Decreased Renal Potassium Excretion
Normally functioning kidneys excrete 80% of the daily potassium intake. Therefore, when the kidney is unable to excrete potassium appropriately, as in acute kidney injury (AKI) and stage 4 to 5 CKD, potassium is retained and often results in hyperkalemia. Finally, many drugs can inhibit the kidney’s ability to excrete potassium by inhibiting aldosterone and thus contribute to an increase in serum potassium concentrations.
Severe hyperkalemia is more common in AKI than in CKD because patients are often hypercatabolic and can have underlying disorders, such as rhabdomyolysis or tumor lysis syndrome, which result in release of potassium from injured or lysed cells.19 Severe hyperkalemia is rare in stable CKD patients, perhaps because of enhanced GI and renal potassium excretion.20 Data suggest that hyperkalemia directly stimulates renal K+ excretion through an effect that is independent of, and additive to, that of aldosterone.20 Although the overall incidence of hyperkalemia is higher in patients with CKD when compared with patients without CKD, due to these adaptive mechanisms and their decreased susceptibility to cardiac effects of chronic hyperkalemia, it has been associated with a lower mortality rate.21 Renal excretion of potassium is also inhibited by various endocrinologic disorders, including adrenal insufficiency, Addison’s disease, and selective hypoaldosteronism. All of these disorders involve a decreased production of aldosterone, which results in the retention of potassium.
Several drugs have profound effects on the kidney’s ability to regulate potassium. Five drug classes in particular have specific effects on the kidney: angiotensin-converting enzyme inhibitors (ACEIs), angiotensin-II receptor blockers (ARBs), direct renin inhibitors, potassium-sparing diuretics, and prostaglandin inhibitors such as nonsteroidal antiinflammatory drugs (NSAIDs). Although hyperkalemia with these drugs is typically dose dependent, the rates of hyperkalemia have been reported to range from 5% to 10% in most clinical trials. Other commonly used drugs that can cause hyperkalemia are digoxin, cyclosporine, tacrolimus, trimethoprim-sulfamethoxazole, heparin, and pentamidine.
Tubular Unresponsiveness to Aldosterone
Certain medical conditions, such as sickle cell anemia, systemic lupus erythematosus, and amyloidosis, can produce a defect in tubular potassium secretion, possibly as the result of an alteration in the aldosterone-binding site.
Redistribution of Potassium into the Extracellular Space
The efflux of potassium from within the cell into the extracellular fluid, which is associated with no change in total-body potassium stores, is to be expected in the presence of metabolic acidosis, diabetes mellitus, chronic renal failure, or lactic acidosis. β-Blockers can also result in a transcellular potassium shift.
The serum potassium concentration can also be falsely elevated in some conditions, and not reflect the actual in vivo potassium concentration, that is, pseudohyperkalemia. Pseudohyperkalemia occurs most commonly in the setting of extravascular hemolysis of red blood cells. When a blood specimen is not processed promptly and cellular destruction occurs, intracellular potassium is released into the serum. It can also occur in conditions of thrombocytosis or leukocytosis. If severe hyperkalemia is found in a patient who is asymptomatic with an otherwise normal laboratory report, the hyperkalemia is most likely pseudohyperkalemia, and a repeat blood sample should be evaluated. Truly elevated potassium concentrations are normally associated with other laboratory abnormalities, such as low carbon dioxide (acidosis) or elevated blood urea nitrogen and creatinine concentrations (indicating renal insufficiency).
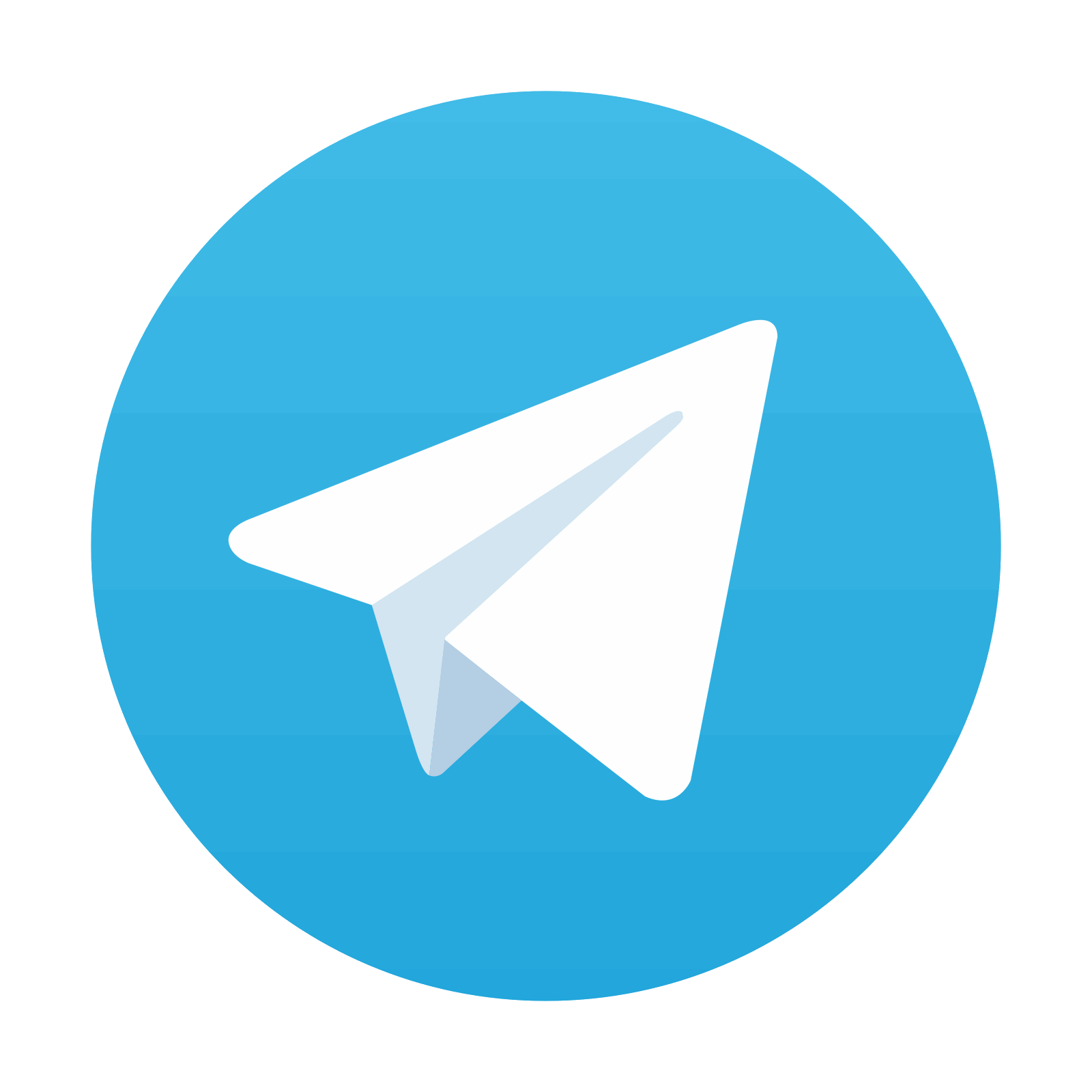