Calcium homeostasis
Calcium balance
In adults, calcium intake and output are normally in balance (Figure 5.1). External balance is largely achieved through the body normally matching net absorption over 24h closely with the corresponding 24-h urinary excretion; this varies with the diet. On a normal diet, urinary calcium excretion in healthy adults may overlap with the output in some patients who are renal stone-formers. In infancy and childhood, there is normally a positive balance, especially at times of active skeletal growth. In older age, calcium output may exceed input, and a state of negative balance then exists; this negative external balance is particularly marked in women after menopause, and is important in the development of post-menopausal osteoporosis. In women, the mother loses calcium to the foetus during pregnancy, and by lactation.
Figure 5.1 Summary of the typical daily movements of calcium between ECF, gut, bone and kidney.
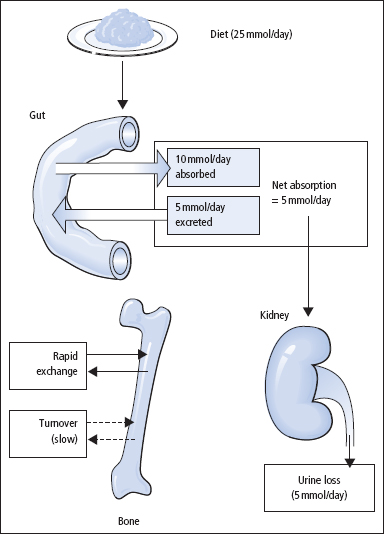
Biological function of calcium
Calcium is a major mechanical constituent of the bone. Bone by itself is a specialised mineralised connective tissue containing cellular elements (bone-forming osteoblasts and bone-resorbing osteoclasts), organic matrix (type I collagen, proteoglycan, etc.) and the calcium-containing mineral hydroxyapatite. Calcium salts in bone have a mechanical role, but are not metabolically inert. There is a constant state of turnover in the skeleton associated with deposition of calcium in sites of bone formation and release at sites of bone resorption (~5% per year of the adult skeleton is remodelled). Calcium in the bone also acts as a reservoir that helps to stabilise ECF [Ca2+].
Maintenance of extracellular [Ca2+] within narrow limits is necessary for normal excitability of nerve and muscle. An increase in [Ca2+] raises the threshold for the nerve action potential, and vice versa. The ion is also required in the activation of the clotting and complement cascades.
While the ECF [Ca2+] is approximately 1 mmol/L (10–3 M), cytosolic [Ca2+] is much lower, approximately 100 nmol/L (10–7 M). Cells possess a number of transport mechanisms for Ca2+ that allow maintenance of this large gradient across the cell membrane.
An increase in cytosolic [Ca2+] serves as a signal for several cell processes, which include cell shape change, cell motility, metabolic changes, secretory activity and cell division. Many intercellular signals, including several hormones, bring about an increase in cytosolic [Ca2+] by opening plasma membrane Ca2+ channels, or by releasing intracellular stores of Ca2+, or by a combination of these effects.
Control of calcium metabolism
Calcium is present in plasma in three forms (Table 5.1), in equilibrium with one another. Plasma [Ca2+] is the physiologically important component, and is closely regulated in humans by PTH and 1:25-dihydroxycholecalciferol (DHCC): both act to increase plasma [Ca’+] and hence plasma [calcium]. The body’s responses to a fall in plasma [Ca2+], in terms of changes in PTH and 1:25-DHCC production, are shown in Figure 5.2. Growth hormone (GH), glucocorticoids (e.g. cortisol), oestrogens, testosterone and thyroid hormones (thyroxine (T4) and tri-iodothyronine (T3)) also influence calcium metabolism.
Parathyroid hormone (PTH)
PTH is the principal acute regulator of plasma [Ca2+]. Plasma PTH levels exhibit a diurnal rhythm, being highest in the early hours of the morning and lowest at about 9 am. The active hormone is secreted in response to a fall in plasma [Ca2+], and its actions are directed to increase plasma [Ca2+]. An increase in plasma [Ca2+] suppresses PTH secretion.
- In bone, PTH stimulates bone resorption by osteoclasts, with a requirement for osteoblasts to mediate this effect. Biochemical measures of both increased osteoblast activity (e.g. increased serum ALP activity) and increased osteoclast activity (e.g. raised urinary hydroxyproline and deoxypyridino-line excretion) may be evident. In severe hyperparathyroidism, radiological demineralisation may be seen, including subperiosteal resorption of the terminal phalanges, bone cysts and pepper skull.
- In the kidney, PTH increases the distal tubular reabsorption of calcium. It also reduces proximal tubular phosphate reabsorption and promotes activity of the 1α-hydroxylation of calcidiol (see below). Renal loss of HCO3– also increases, which may lead to a mild metabolic acidosis. Formation of 1:25-DHCC indirectly increases the absorption of calcium from the small intestine.
Table 5.1 The components of calcium in plasma
Calcium component | Percentage of plasma [calcium] |
Ionised calcium, Ca2+ | 50–65 |
Calcium bound to plasma proteins – mainly albumin | 30–45 |
Calcium complexed with citrate, etc. | 5–10 |
1:25-Dihydroxycholecalciferol (1:25-DHCC, or calcitriol)
Most vitamin D3 (cholecalciferol) is synthesised by the action of ultraviolet light on the vitamin D precursor 7-dehydrocholesterol in the skin. Vitamin D3 is also present naturally in food (a rich source is fish oils), while vitamin D2 (ergosterol) is added to margarine.
Endogenous synthesis of vitamin D3 is important. Vitamin D deficiency can develop if exposure to sunlight is inadequate, or because of inadequate dietary intake, but is usually a result of the combined effects of these two factors. In the body, vitamin D3 and vitamin D2 undergo two hydroxylation steps before attaining full physiological activity (Figure 5.3):
- 25-Hydroxylation This occurs in the liver, with the production of 25-hydroxycholecalciferol (25-HCC, or calcidiol). Other inactive metabolites are formed, but are excreted in the bile. The main form of vitamin D circulating in the plasma is 25-HCC, bound to a specific transport protein; it is carried to the kidney for further metabolism. Plasma [25-HCC] shows marked seasonal variation, with levels highest in summer.
- 1 α-Hydroxylation of 25-HCC This takes place in the kidney, with the production of 1:25-DHCC, biologically the most active naturally occurring derivative of vitamin D. The kidney also contains other hydroxylases, such as 24-hydroxylase, which converts 25-HCC to 24:25-DHCC. Renal 1α-hydroxylation is increased by low plasma [phosphate], high [PTH] and where there is a tendency to hypocalcaemia, whatever the cause. The reverse circumstances direct metabolism of 25-HCC towards the formation of 24:25-DHCC, which has no clearly established physiological function.
Figure 5.2 Calcium homeostasis in man showing the main hormonal responses to a fall in plasma [Ca2+], and indicating the places where the negative feedback mechanism operates if plasma [Ca2+] becomes high. The effect of PTH on the renal tubules, causing increased reabsorption of calcium, is not shown.
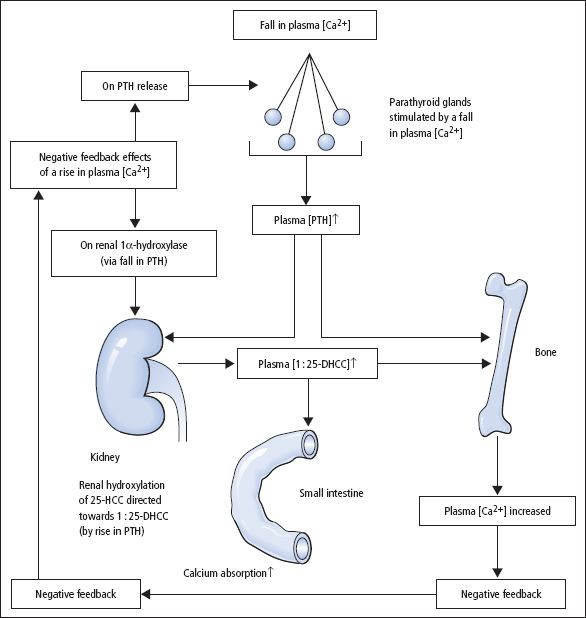
The principal action of 1:25-DHCC is to induce synthesis of a Ca2+-binding protein in the intestinal epithelial cell necessary for the absorption of calcium from the small intestine. Deficiency of 1:25-DHCC leads to defective bone mineralisation. Maintenance of both ECF [Ca2+] and ECF [phosphate] by 1:25-DHCC may be a key factor in normal mineralisation.
Figure 5.3 The formation of 1:25-DHCC, the most active form of vitamin D3, from pro-vitamin D3 (normally the main source of the vitamin in man) and from dietary vitamin D3. Vitamin D2 (ergosterol) undergoes similar hydroxylations. By the action of ultraviolet (UV) light, pro-vitamin D3 is converted in the dermis into pre-vitamin D3 (not shown) in which the B-ring of the steroid skeleton has been opened; pre-vitamin D3 then rearranges spontaneously to give vitamin D3. The factors that influence the hydroxylation of 25-HCC in the direction of 1:25-DHCC or 24:25-DHCC are described in the text.
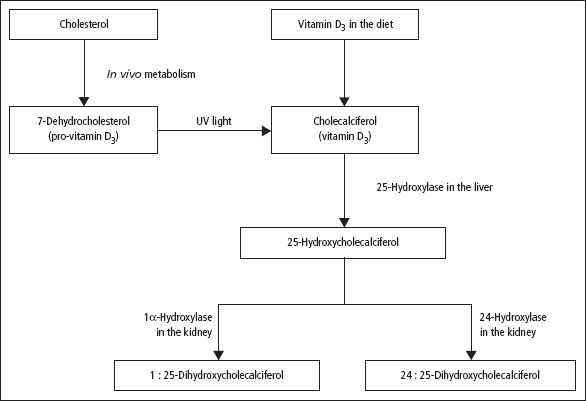
Calcitonin
Although calcitonin can decrease plasma [Ca2+] by reducing osteoclast activity and decreasing renal reabsorption of calcium and phosphate, its actions are transient, and chronic excess or deficiency is not associated with disordered calcium or bone metabolism. Its use as a tumour marker is discussed elsewhere (p. 251).
Investigation of abnormal calcium metabolism
Measurement of serum calcium and albumin, inorganic phosphate and ALP, and sometimes magnesium, PTH and vitamin D metabolites, underlie the diagnosis of most disorders of calcium metabolism.
Serum [calcium] (reference range 2.10–2.60 mmol/L)
Because of technical difficulties associated with the measurement of [Ca2+], clinical biochemistry laboratories only measure serum [calcium] routinely, even though the physiologically important fraction is plasma [Ca2+].
Effects of serum [albumin]
Because albumin is the principal binding protein for calcium, a fall in serum [albumin] will lead to a fall in bound calcium and a decrease in total [calcium] (and vice versa). Under these circumstances, the unbound plasma [Ca2+], the physiologically important fraction, will be maintained at normal levels by PTH. Modest but potentially misleading increases in serum [calcium] may also result from abnormal calcium binding, due to raised serum [albumin]. This is often due to faulty or non-standardised venepuncture technique (p. 242). To avoid misdiagnosis of hypocalcaemia or hypercalcaemia, serum [albumin] should always be measured at the same time as serum [calcium]. The serum [calcium] (in mmol/L) can be approximately ‘corrected’ to take account of an abnormal albumin (in g/L) using a formula such as
Effects of plasma H+
In acidosis, the protonation of albumin reduces its ability to bind calcium, leading to an increase in unbound [Ca2+], and vice versa, without any change in total [calcium]. Thus, hyperventilation with respiratory alkalosis can reduce plasma [Ca2+], with the development of tetany. In chronic states of acidosis or alkalosis, PTH acts to readjust the plasma [Ca2+] back to normal.
Serum [phosphate] (reference range 0.8–1.4 mmol/L)
Serum [phosphate] shows considerable diurnal variation, especially following meals; the reference range relates to the fasting state. Different ranges should be used for different age groups. About 85% of serum phosphate is free and 15% protein bound.
Alkaline phosphatase (ALP)
Reference ranges for serum ALP activity are very method dependent. For physiological reasons, there are also considerable variations in this enzyme’s activity in childhood, adolescence and pregnancy. The bone isoenzyme of ALP activity is increased in serum from patients with diseases in which there is increased osteoblastic activity, for example hyperparathyroidism, Paget’s disease, rickets and osteomalacia, and carcinoma with osteoblastic metastases.
Hypercalcaemia
Increased plasma [Ca2+] is a potentially serious problem that can lead to renal damage, cardiac arrhythmias and general ill-health. The clinical features are listed in Table 5.2. The most common causes (Table 5.3) are primary hyperparathyroidism and malignant disease, although the likelihood of these diagnoses will vary depending on the patient population. In asymptomatic ambulatory patients with hypercalcaemia, primary hyperparathyroidism may account for up to 80% of cases whereas in sick hospitalised hypercalcaemic patients, malignancy-associated hypercalcaemia is more likely.
Primary hyperparathyroidism
Autonomous overproduction of PTH occurs typically from a single, parathyroid adenoma. Diffuse hyperplasia (involving all four glands) or, rarely, parathyroid carcinoma may be responsible.
The excess PTH leads to a raised [Ca2+], with the potential for clinical problems (Table 5.2). Both serum [calcium] and [albumin] should be measured, and may need to be repeated, since the hypercalcaemia can be intermittent. Serum [phosphate] is sometimes low as a result of the phosphaturic effect of PTH, though this is not a reliable finding. A mild metabolic acidosis may be present, since PTH increases urinary losses. Some patients develop bony problems as a consequence of the high plasma [PTH], especially if the problem becomes chronic. Markers of increased osteoblast and osteoclast activity may be increased (p. 82). Table 5.4 summarises the results of first-line chemical tests for the investigation of suspected hyperparathyroidism.
Table 5.2 Clinical consequences of high [Ca2+]
Neurological symptoms (inability to concentrate, depression, confusion) |
Generalised muscle weakness |
Anorexia, nausea, vomiting, constipation |
Polyuria with polydipsia |
Nephrocalcinosis, nephrolithiasis |
ECG changes (shortened Q–T interval), with bradycardia, first-degree block |
Pancreatitis, peptic ulcer |
Table 5.3 The causes of hypercalcaemia
Category | Examples |
Common | |
Parathyroid disease | Hyperparathyroidism, primary and tertiary; multiple endocrine neoplasia syndromes, MEN I and MEN IIa |
Malignant disease | Lytic lesions in bone: myeloma, breast carcinoma |
PTHrP: carcinoma of lung, oesophagus, head and neck, renal cell, ovary and bladder | |
Ectopic production 1:25-DHCC by lymphomas | |
Uncommon | |
Endogenous production of 1:25-DHCC | Sarcoidosis and other granulomatous diseases |
Excessive absorption of calcium | Vitamin D overdose (including self-medication); milk–alkali syndrome |
Bone disease | Immobilisation |
Drug induced | Thiazide diuretics, lithium |
Miscellaneous (mostly rare) | Familial hypocalciuric hypercalcaemia Hypercalcaemia in childhood(p. 292) Thyrotoxicosis Addison’s disease |
Artefact | Poor venepuncture technique (excessive venous stasis) |
Table 5.4 ‘First-line’ biochemical tests for investigating suspected hyperparathyroidism
Plasma or serum | Comments |
Calcium | If increased [calcium], supports the diagnosis |
Albumin | Should be performed as a check on plasma [calcium] |
Phosphate (fasting) | If decreased [phosphate], supports the diagnosis |
ALP | If enzymic activity increased, supports the diagnosis |
Total CO2 | If decreased, supports the diagnosis |
Creatinine and/or urea | Simple tests of renal function, needed in all patients with suspected abnormalities of calcium metabolism PTH |
PTH assay
The single most important test in the differential diagnosis of hypercalcaemia is the measurement of serum PTH (Figure 5.4). Immunometric (‘sandwich’) assays that measure serum [intact PTH] (reference range 10–55 ng/L) are now in widespread use. They have a high diagnostic sensitivity, and up to 90% of patients with primary hyperparathy roidism have an elevated level. Approximately 10% of patients with primary hyperparathyroidism may have serum [intact PTH] in the upper part of the reference range. However, in the setting of persistent hypercalcaemia, such levels are considered to be inappropriately high since serum [intact PTH] should be suppressed if hypercalcaemia is unrelated to increased parathyroid activity. If serum [intact PTH] is within reference limits or is only marginally elevated in an asymptomatic hypercalcaemic patient, the diagnosis of familial benign hypocalciuric hypercalcaemia (FBHH) should also be considered (see below).
Figure 5.4 Biochemical investigation of persistent hypercalcaemia.
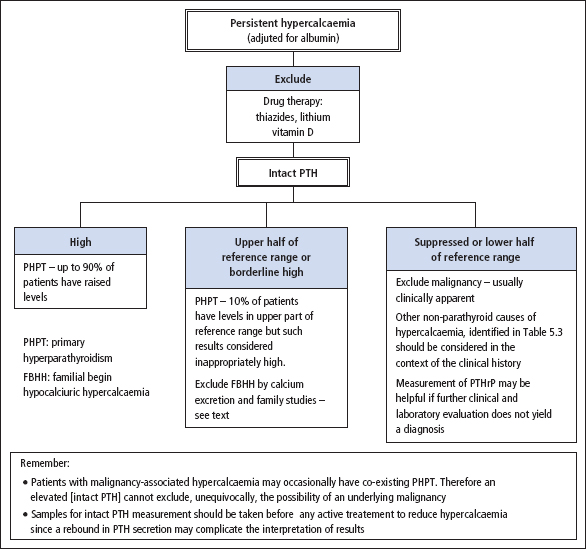
Sometimes PTH assays are required for the investigation of patients who also require urgent therapeutic intervention to reduce marked hypercalcaemia. Under these circumstances, it is important to obtain a sample for PTH analysis before treatment is initiated, since an acute fall in plasma [Ca2+] may trigger a rebound in PTH secretion and complicate the interpretation of the result.
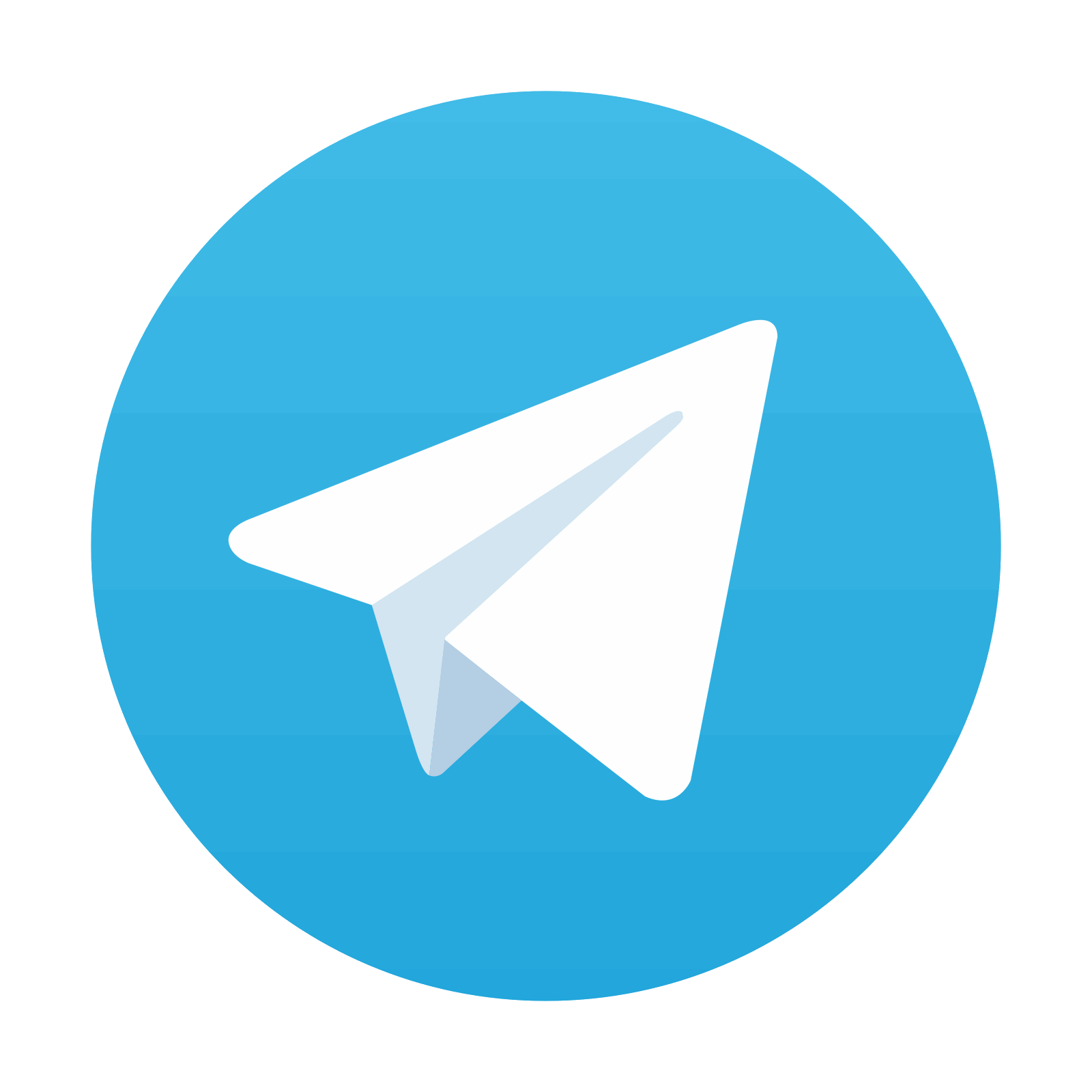
Stay updated, free articles. Join our Telegram channel

Full access? Get Clinical Tree
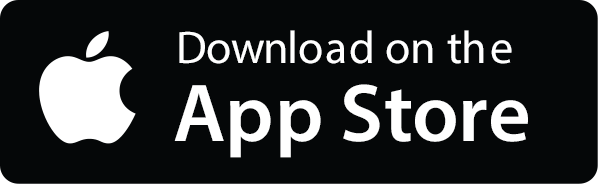
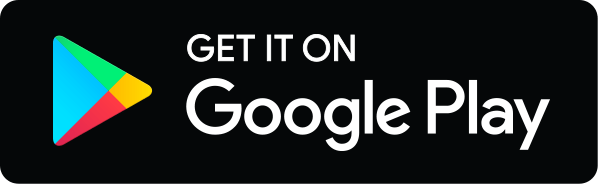