
measures are implemented. Salmonellosis, brucellosis, and leptospirosis as well as parvovirus, herpesvirus, and rotavirus infections are examples of diseases in which extensive environmental contamination occurs. Depending on their predilection sites in the host animal’s body, pathogenic microorganisms may be shed in secretions, fluids, excretions and animal products such as meat, eggs, and offal (Figure 52.1). For some infectious agents, aerosol transmission is an important mode of transfer among susceptible animals. Avian chlamydiosis, caused by Chlamydia psittaci, occurs in a wide range of both wild and domestic species. The organism, which is present in respiratory discharges and feces of infected birds, is usually acquired by inhalation or ingestion. In addition to its importance in avian species, C psittaci is recognized as a cause of sporadic disease in the human population, often with respiratory symptoms.


![]() FIGURE 52.1 Modes of transmission of infectious agents from infected to susceptible animals and relevant control measures. |
by vectors, and from environmental sources. An effective biosecurity program has many components, all aimed at ensuring that the risks of healthy animals acquiring infection are minimized (Table 52.2). Design considerations for farms engaged in the implementation of biocontainment policies are illustrated in Figure 52.4.
TABLE 52.1 Methods for the prevention, treatment, and control of particular infectious agents | |||||||||||||||||||||||||||||||||||||||||||||||||||||||||||||||||||||||||||||
---|---|---|---|---|---|---|---|---|---|---|---|---|---|---|---|---|---|---|---|---|---|---|---|---|---|---|---|---|---|---|---|---|---|---|---|---|---|---|---|---|---|---|---|---|---|---|---|---|---|---|---|---|---|---|---|---|---|---|---|---|---|---|---|---|---|---|---|---|---|---|---|---|---|---|---|---|---|
|
Chemical disinfection of
buildings, bedding, and equipment
transport vehicles
footwear and clothing of workers
Chemical treatment of water supply, following disinfection of building
Chemical treatment of fluids, excretions, secretions
Heat treatment of milk and milk products; mandatory boiling of waste food if swill feeding to pigs is permitted
For buildings with slatted floors, animal waste is stored in slurry tanks. These tanks should be constructed to high specifications and have ample capacity to avoid overflowing of contents. Slurry spreading is usually restricted to defined times of the year when ground conditions are suitable for slurry tankers and when the risk of runoff is low. An interval of at least 2 months should elapse between the application of slurry to pasture and the commencement of grazing. A longer interval between the application of slurry and the commencement of grazing may be required if enteric pathogens such as Salmonella species or pathogenic acid-fast bacteria are likely to be present in the slurry.
TABLE 52.2 Components of a biosecurity program for farm animals, including poultry | ||||||||||||||||||||||||||||||
---|---|---|---|---|---|---|---|---|---|---|---|---|---|---|---|---|---|---|---|---|---|---|---|---|---|---|---|---|---|---|
|

been in continual use for decades, are gaining acceptance as effective disinfectants for infectious diseases of animals. Combinations of antimicrobial compounds that have received favorable evaluation in published reports include quaternary ammonium compounds (QACs) combined with glutaraldehyde (a proprietary disinfectant), accelerated hydrogen peroxide (containing a reduced concentration of hydrogen peroxide supplemented with acids, surfactants, wetting agents, and chelating agents), and hydrogen peroxide combined with silver nitrate. Characteristics of an ideal chemical disinfectant include
Broad antimicrobial spectrum with effective activity against vegetative bacteria (including mycobacteria), bacterial endospores, fungal spores, enveloped and nonenveloped viruses, oocysts of pathogenic protozoa, and prions
Absence of irritancy, toxicity, teratogenicity, mutagenicity, and carcinogenicity for personnel implementing disinfection programs and also for animals occupying buildings where the chemical disinfectant will be used
Stability, with a long shelf life at ambient temperatures
Effectiveness against bacteria in biofilms or pathogenic microorganisms dried on surfaces
Compatibility with a wide range of chemicals including acids, alkalis, and anionic and cationic compounds
Retention of antimicrobial activity in the presence of organic matter
Solubility in water to the concentration required for effective antimicrobial activity
Absence of corrosiveness or chemical interactions with metallic fittings, plastic, rubber, or synthetic structural materials
Absence of tainting or toxicity following application to surfaces or equipment in dairies, meat, plants, or food processing areas
Moderately priced and readily available
Nonpolluting for groundwater or air, nontoxic for aquatic species and biodegradable
relate to the chemical composition of prions, which are described as being composed of altered or denatured protein.

TABLE 52.3 General characteristics and antimicrobial spectrum of disinfectants commonly used in veterinary medicinea | ||||||||||||||||||||||||||||||||||||||||||||||||||||||||||||||||||||||||||||||||||||||
---|---|---|---|---|---|---|---|---|---|---|---|---|---|---|---|---|---|---|---|---|---|---|---|---|---|---|---|---|---|---|---|---|---|---|---|---|---|---|---|---|---|---|---|---|---|---|---|---|---|---|---|---|---|---|---|---|---|---|---|---|---|---|---|---|---|---|---|---|---|---|---|---|---|---|---|---|---|---|---|---|---|---|---|---|---|---|
|
of anthrax, were being buried. Current scientific observations indicate that lime, rather than acting as a disinfectant for endospores of B anthracis, may actually prolong their survival.8
and potassium dichloroisocyanurate are examples of organic chlorine compounds. The antimicrobial activity of these compounds is reported to be slower than that of hypochlorites, but some of these organic chloride compounds are less susceptible to inactivation by organic matter than sodium hypochlorite. Chlorine dioxide, which is a gas at room temperature, is being increasingly used for chlorination of potable water, also in swimming pools and for treatment of wastewater. This gas, which is soluble in water, forming a stable solution in the dark, decomposes slowly when exposed to light. At concentrations above 10% in air, chlorine dioxide is unstable and explosive. However, it is an effective antimicrobial compound and nonflammable and not explosive at concentrations used for sterilization. Chlorine dioxide has a broad antimicrobial spectrum. It is bactericidal, fungicidal, virucidal, and sporicidal and reported to be capable of inactivating prions. The sterilizing capability of chlorine dioxide is reported to be equivalent to EO. The antimicrobial activity of chlorine and its compounds is due to the formation of hypochlorous acid, which forms when free chlorine is added to water. Hypochlorites and chloramines undergo hydrolysis when added to water, leading to the formation of hypochlorous acid. This acid releases nascent oxygen, a powerful oxidizing agent. Chlorine compounds combine directly with bacterial cytoplasmic proteins and with viral capsid proteins. Sodium hypochlorite and other chlorine compounds are most effective at pH values below 7, and their antimicrobial activity is inversely proportional to the pH of the environment in which they are used. As diluted chlorine disinfectants lose their potency, fresh solutions should be prepared for use. Hypochlorites are among the most widely used disinfectants. Sodium hypochlorite is fast acting, nonstaining, and inexpensive. Its use in veterinary medicine, however, is limited by its corrosiveness, its inactivation by organic matter, and its relative instability.
bacteria by the presence of organic matter and inorganic material or by biofilm formation on the items being treated. Addition of alcohol improves the antimicrobial activity of iodophors. In some countries, alcoholic solutions of iodophors are widely used for disinfection of hands and sites prior to surgical procedures. Acidic iodophor solutions are used as sanitizers in the food industry. When employed as disinfectants in dairy plants, the pH of iodophors is kept acidic by the addition of phosphoric acid to ensure the removal of dried milk residues. Effective postmilking teat dipping is an important control measure for contagious mastitis in dairy cattle, and iodophors are among the common teat dips used for this purpose.
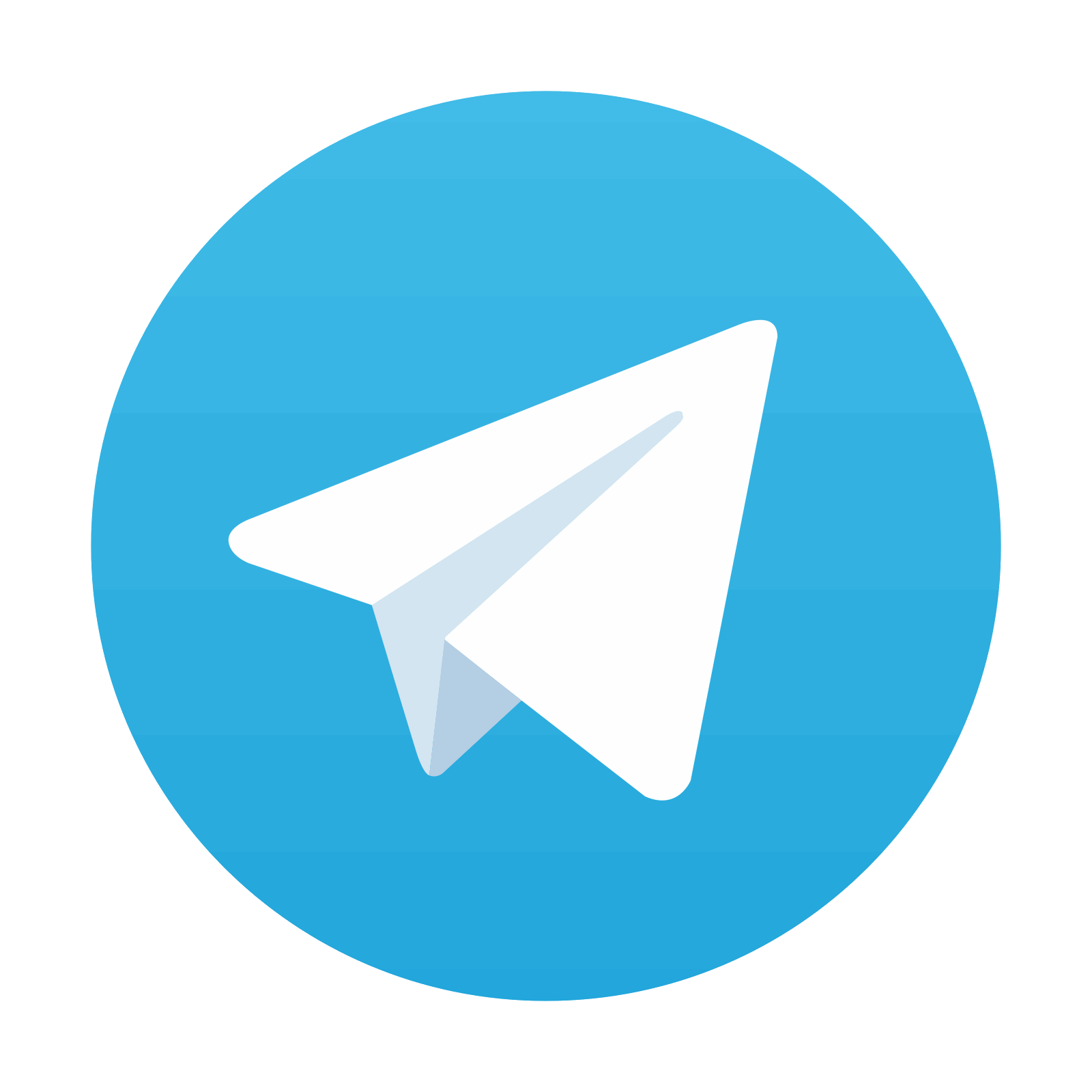
Stay updated, free articles. Join our Telegram channel

Full access? Get Clinical Tree
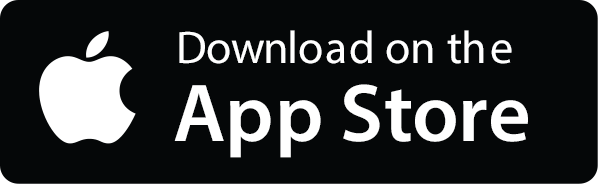
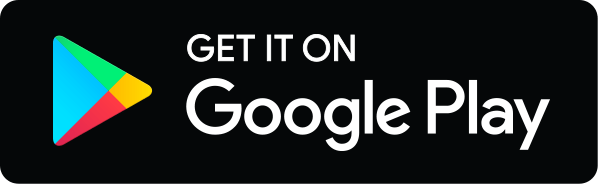