Can decrease or block METH exposure in the brain
Can be combined with behavioral therapy and other medications to:
Increase patient “willpower” to quit abusing METH
Aid in the initiation and continuation of more positive behaviors (i.e., not using drugs)
Longer term therapeutic effects compared to small-molecule medications due to lengthy half-life of IgG
9.2 Anti-METH Monoclonal Antibody Compared to Active Immunization with a METH-Conjugate Vaccine for the Treatment of METH Abuse
Medical treatment with anti-METH antibodies could be accomplished in several ways, but the two major methods currently in clinical testing or being prepared for testing are the administration of preformed anti-METH monoclonal antibodies (mAb, both singular and plural) or active vaccination with a METH-conjugate vaccine (MCV). Anti-METH IgG antibodies are large-molecular-weight proteins (~150 kDA) with two high-affinity METH binding sites (e.g., 7 nM Kd for METH). After intravenous administration of anti-METH mAb to animals, results show the antibody remains mainly confined to the blood plasma (not red blood cells) and extracellular fluid space of the body. For this reason it is not surprising that in vivo mAb binding of METH is very high in the blood plasma (Gentry et al. 2009; Owens et al. 2011).
Compared to production of anti-METH polyclonal antibodies generated by active immunization, mAb can provide protective effects against METH immediately after mAb treatment. Acute studies in rats show that the administration of anti-METH mAb immediately decreases METH-induced stimulant effects (Byrnes-Blake et al. 2003; Laurenzana et al. 2014). Active immunization with a MCV, however, requires approximately 9–12 weeks before the antibody titers and affinity for METH are high enough to offer meaningful pharmacological protection (Rüedi-Bettschen et al. 2013). Compared to MCVs, anti-METH mAb treatment can produce predictable and reproducible serum antibody concentrations. Because they can be administered intravenously in known doses, they circumvent the inherent individual patient variability in polyclonal immune response after immunization with a MCV. In humans taking immunosuppression medications (e.g., glucocorticoids) or with immune-compromised disease states (e.g., AIDS), it may not be possible to use active immunization for developing an anti-METH antibody response. This problem would not occur with mAb therapy since the antibodies are preformed and could be administered to most patients as needed. MAb therapy could also achieve much higher concentrations than produced by active vaccination and could provide the opportunity to dose to a desired effect level. Unfortunately, the much higher cost of a mAb is a significant factor in the amount that can be administered. In addition to the immediate and controllable anti-METH antibody concentrations, a mAb medication is a homogenous solution of the same high-affinity anti-METH antibody. Rather than the polyclonal antibody response from a MCV that generates antibodies with a variety of affinities and specificities for METH, each mAb has exactly the same amino acid sequence, affinity, and specificity for METH. This assures a more consistent treatment among patients.
Each type of immunotherapy has advantages and disadvantages, which will require testing in humans before they can be adequately judged. However, it is also possible that in certain clinical scenarios, mAb and vaccination could be used in combination (Carrera et al. 2000; Roiko et al. 2008; Cornish et al. 2011).
While our scientific team is working on both types of antibody-based therapy, we think anti-METH mAb therapy has the most advantages for treating patient addiction. These advantages and possible disadvantages will be discussed in detail in upcoming sections. Table 9.2 summarizes some of the key advantages and disadvantages of mAb therapy.
Table 9.2
Potential advantages and disadvantages of anti-METH mAb therapy
Advantages |
Immediate protection from METH overdose and METH-induced relapse to addiction |
Well-defined pharmaceutical preparation |
Dosing by established principles of clinical pharmacology |
Therapeutic levels are easily maintained |
MAb duration of action and affinity can be modified by protein engineering |
Because the biological half-life is very long in humans (e.g., 3 weeks), patient compliance and use are likely to be improved |
Nonaddictive |
Disadvantages |
Cost of the medications is high |
Eventual immune response against mAb could limit length of treatment |
9.3 The Mechanism of Action for Anti-METH mAb-Mediated Reductions in METH Effects
The most common reason suggested by patients for their persistent METH use and relapse to METH use is the positive-reinforcing effects of METH (Newton et al. 2009). This makes METH a disease target for antibody medications. Anti-METH mAb therapy is predicted to reduce the overall METH-induced effects including the reward type effects associated with continued or renewed use in addicted patients. Anti-METH mAb treatment shows efficacy in a variety of preclinical rat models of METH abuse including the reduction of METH brain concentrations, reduction of METH-induced locomotor effects, decreases in the duration of these effects (Byrnes-Blake et al. 2003; Laurenzana et al. 2014; Hambuchen et al. 2014), and the reduction of METH self-administration (McMillan et al. 2004).
Anti-METH mAb has dramatic effects on the disposition of in vivo METH. The apparent METH volume of distribution in humans is very high, at about 3.73 L/kg or 261 L in a 70 kg human (Cook et al. 1993), indicating extensive extravascular METH distribution. In stark contrast, the apparent volume of distribution of chimeric anti-METH mAb7F9 (ch-mAb7F9, to be discussed later in the chapter) is only 5–6 L in healthy adult male or female subjects following a single ch-mAb7F9 dose of 0.2–20 mg/kg (Stevens et al. 2014a, ClinicalTrials.gov Identifier: NCT01603147).
In an adult male or female of 70 kg, this is a volume of distribution of about 0.08 L/kg. This means that when METH is bound by high-affinity ch-mAb7F9 (METH K d = 6.9 nM), the distribution of METH out of the bloodstream will be substantially reduced (Stevens et al. 2014b). In rat studies of a 1 mg/kg METH dose in the presence of a mouse anti-METH mAb, there was a substantial increase in area under the METH serum concentration-time curve relative to control rats without antibody treatment (Laurenzana et al. 2003). Binding of METH to the antibody also alters the clearance of METH. The effects on METH clearance will be discussed later in this chapter.
As depicted in Fig. 9.1, anti-METH mAb binding decreases entry of METH across the blood-brain barrier to active sites in the brain (Byrnes-Blake et al. 2003; Laurenzana et al. 2003, 2009). For example, a continuous subcutaneous METH infusion of 5.6 mg/kg/day in rats followed by anti-METH mAb administration results in immediate reductions in brain METH concentrations (Laurenzana et al. 2009). As another component of the mechanism of action, these antibodies are thought to decrease the rate of entry of METH into the brain. Human subjective responses to cocaine (e.g., “high” and “liking”) are significantly lower when the drug is infused more slowly (Abreu et al. 2001). Based on data from tissue distribution studies in rats, anti-METH mAb reduces METH entry into a variety of other critical organs, including the fetal brain in utero (Laurenzana et al. 2003; White et al. 2014). These tissue changes in METH disposition lead to decreases in METH-induced pharmacological (e.g., psychomotor and cardiovascular) effects in animal models (Gentry et al. 2006; Laurenzana et al. 2014; Hambuchen et al. 2014).
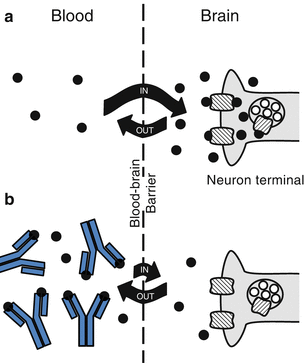
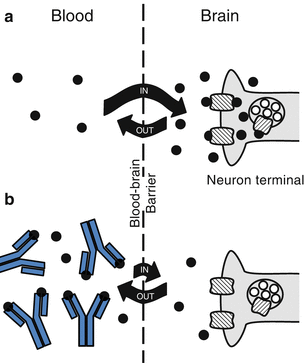
Fig. 9.1
Anti-METH mAb effects on METH brain concentrations. (a) METH (black circles) brain entry before anti-METH antibody administration leads to interaction with neurons resulting in the release of monoamines (open circles) and production of stimulant actions. (b) Anti-METH antibody (“Y”-shaped symbols) binds METH in the bloodstream and reduces the amount and rate (as denoted by the direction and length of the curved arrows) of METH brain entry
Anti-METH polyclonal antibodies resulting from active vaccination can block high-dose METH (3 mg/kg) inhibition of food-maintained behavior (Rüedi-Bettschen et al. 2013) despite the lower titers, affinity, and consistency of response compared to mAb treatment.
Inhibition of METH-induced locomotor activity is a frequently used preclinical measure of the effectiveness of candidate anti-METH mAb by our research group. It is often combined and correlated with pharmacokinetic changes in METH serum and brain concentrations in the same set of animals. Repeated METH administration, however, can produce sensitization and shifting baseline effects from dose to dose (despite complete elimination of METH between doses). While higher stimulant doses produce increased incidence of early locomotor activity suppressing stereotypic effects (Schiorring 1971), these effects also increasingly occur with repetitive administration of even low stimulant doses (Segal and Mandell 1974). While decreasing locomotor activity with an antibody is not a direct measurement of decreasing METH addiction, it does show the inhibition of a METH-induced effect which results from METH-induced dopamine release in the CNS (Sellings and Clarke 2003). Considering that anti-METH mAb act by restricting the entry of METH to the brain, this is likely a suitable measurement of an overall decrease in METH effects. The measurement is also noninvasive compared to directly measuring brain drug or neurotransmitter concentrations. Since it can be performed without significant training of animals, it allows for higher throughput testing of multiple key time points during an anti-METH mAb treatment regimen.
9.4 Anti-METH mAb Has Prolonged Duration of Action
An important potential therapeutic advantage of mAb over small-molecule medications is the extended duration of action and the likely long time periods between required mAb administrations. This is due to the very long elimination half-lives of mAb, which range from approximately 2–4 weeks in humans. The long half-life of these therapeutic proteins results from in vivo FcRn (Fc receptor of the neonate) recycling of IgG mAb proteins. This process protects IgG molecules from intracellular catabolism (Lobo et al. 2004; Wang et al. 2008).
Most small-molecule drugs used for the treatment of chronic diseases like high blood pressure or depression require patients to take their medication 1–3 times a day for extended or indefinite periods of time. This is manageable with self-motivated, compliant patients. However, patients being treated for METH addiction would likely have difficulty complying with frequent dosing on a daily basis.
Most small-molecule medications are dosed based on the drug’s half-life. For example, a drug with a 10 h half-life might be effectively dosed in patients twice a day. Assuming the same pharmacological principles apply to the dosing of anti-METH mAb medications, the mAb could be administered by an intravenous infusion once every 3 weeks if the half-life of the mAb is in the range of 18–24 days. We think this would offer patients a significant advantage because the protective anti-METH mAb medication would be present in the bloodstream during the extended periods of time when they are not under direct medical care or counseling for their addiction. The disadvantage would be that the patient might forget to be treated with such infrequent scheduled treatment periods.
While dosing with an anti-METH mAb could offer immediate therapeutic benefits, one of the many unknowns concerning the use of mAb to treat addiction is how long it will take to reach maximum effects. For most small-molecule medications, well-established principles of clinical pharmacokinetics show that the time needed to reach maximum steady-state concentrations in the body is only dependent on the half-life of the drug (Rowland and Tozer 1995). After 1, 2, 3, or 4 half-lives of the drug, 50, 75, 87.5, and 94 %, respectively, of the final steady-state concentrations are reached. Most clinical pharmacologists agree that after four half-lives of a medication, the maximum effects (and side effects) would be achieved. Thus, if the patient is dosed with an anti-METH mAb medication once every mAb half-life (e.g., once every 3 weeks), after 12 weeks the patient’s steady-state value of mAb concentration would be at ~94 % of the final value. To achieve steady-state concentrations more rapidly, a higher loading dose could be used at the start of the treatment. This treatment strategy will be demonstrated in a later section of this review.
9.5 Regeneration of METH Binding Capacity by In Vivo METH Elimination Processes
Another important aspect of the duration of action and efficacy of anti-METH antibodies is related to the fate of the antibody-METH complex. In vivo elimination processes play a major role in maintaining the functional capacity of the mAb to neutralize METH effects. As illustrated, METH is cleared in the male rat primarily by metabolism and in male humans primary by renal passive filtration along with some metabolism (Cook et al. 1993; Mendelson et al. 1995; Rivière et al. 1999). The pharmacokinetic values for the male rat and male human are, respectively, volume of distribution, 9.0 and 3.73 L/kg; clearance, 126 and 3.2 ml/min/kg; and t 1/2, 63 min and 13.1 h. As discussed earlier, a mAb IgG is cleared by intracellular catabolism (Lobo et al. 2004; Wang et al. 2008). Pharmacokinetic analysis of METH concentration-time data in the absence and presence of anti-METH ch-mAb7F9 (to be discussed later in this chapter) in male Sprague-Dawley rats shows the following results for the terminal elimination t 1/2 of ch-mAb7F9, METH, and ch-mAb7F9 plus METH (Table 9.3).
Table 9.3
Ch-mAb7F9 and METH t 1/2 values in the absence and presence of two different doses of anti-METH ch-mAb7F9 in male Sprague-Dawley rats
Rat treatment group (n = 12) | Saline or METH Dose (sc) | Ch-mAb7F9 Dose (iv) | t1/2 ch-mAb7F9 (Days) | t1/2 METH (h) |
---|---|---|---|---|
Ch-mAb7F9 only | Saline | 15 mg.kg | 10.9 | |
Ch-mAb7F9 only | Saline | 150 mg/kg | 11.5 | |
METH only | 1 mg/kg | 1.1 | ||
METH + Ch-mAb7F9 | 1 mg/kg | 15 mg/kg | 13.1 | 2.3 |
METH + Ch-mAb7F9 | 1 mg/kg | 150 mg/kg | 9.8 | 6.5 |
In the studies summarized in Table 9.3, the elimination half-life of METH in the presence of an anti-METH mAb (i.e., 2.3–6.5 h) is much closer to the value for the half-life of METH alone (1.1 h) than to the mAb half-life (9.8–13.1 days). We also find that even though METH binding in the presence of anti-METH murine mAb is very high, the unbound METH concentrations are also relatively high (Laurenzana et al. 2009). Considered together, these data show the capacity for mAb binding of METH in vivo can be regenerated (or restored) through mammalian in vivo METH elimination processes (see Fig. 9.2) in the rat. It is likely that the high-affinity binding of METH continuously works to redistribute the drug by lowering the volume of distribution of METH (discussed earlier). This redistribution makes greater amounts of unbound METH available in the systemic circulatory system for metabolic and renal clearance.
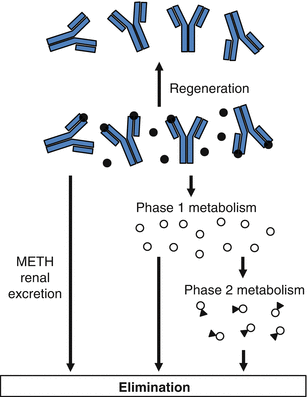
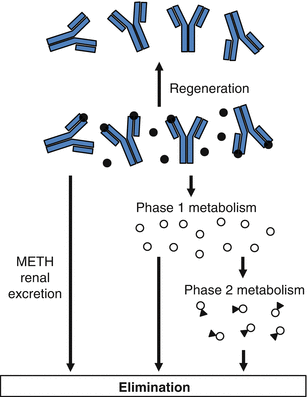
Fig. 9.2
Regeneration of anti-METH antibody binding capacity through continuous elimination of METH (black circles) from serum. Unbound METH is either excreted unchanged by the kidney or metabolized to Phase I (open circle) and Phase II METH metabolites (open circles with attached triangle). These in vivo METH elimination processes (e.g., metabolic and renal excretion) allow the long-acting mAb to become quickly available for more METH binding, which regenerates its capacity
This ability to restore METH binding capacity allows each molecule of anti-METH mAb to block or reduce the effects of many molecules of METH during its prolonged biological half-life. This mechanism of regeneration likely has major impact on the functional capacity of the mAb to neutralize METH effects, especially when METH is administered in excess of available mAb binding sites.
A clear understanding of how the antibodies function in vivo came through years of studying several prototype mAb medications. We will now discuss the discovery process and testing of these anti-METH mAb.
9.6 Selection of Anti-METH mAb Medications and Preclinical Testing
Our initial goal was to discover a METH-specific mAb with a K d value of ≤10 nM. We set this criteria based on success with the preclinical testing of an anti-phencyclidine mAb medication with a K d value of 1 nM for phencyclidine binding (Hardin et al. 2002). The first anti-METH mAb to achieve this criterion was mAb6H4. The lessons learned from the testing of this mAb (both good and bad) helped us establish many of the selection criteria needed to discover an optimized clinical candidate mAb.
The general methods and experimental strategies for selection and large-scale production of mAb are reported in previous publications (Peterson et al. 2007; Carroll et al. 2009). In this section, a brief overview of the process will be described. To produce anti-METH mAb, we start by immunizing female BALB/c mice with a METH-conjugate vaccine (MCV). This MCV is composed of a carefully designed METH-like hapten and antigenic carrier protein such as ovalbumin or bovine serum albumin. After several months of booster immunizations to maximize anti-METH titers and affinity, we select a single mouse for use in generating high-affinity mAb. The selection of the optimal mouse is based on immunochemical characteristics of the anti-METH polyclonal antibodies in their serum.
The initial immunochemical criterion for selection of a candidate mouse includes high-affinity METH binding of the mouse antiserum (as measured by the dissociation constant [K d] for METH), specificity for METH-like chemicals and drugs, and lack of significant cross-reactivity with other small-molecule ligands. Radioimmunoassays using a [3H]-METH radioligand are used for these immunochemical studies (Owens et al. 1988; Peterson et al. 2007).
Initially, the affinity of mAb6H4 for METH was determined to be K d = 11 nM (Byrnes-Blake et al. 2003), but with improvements in the radioimmunoassay determination, the K d value was found to actually be 4 nM (Owens et al. 2011). MAb6H4 was highly specific for METH with <0.1 % cross-reactivity with a wide range of test drugs and compounds, which included chemicals that were structurally related and structurally unrelated to METH. The only exception was methylenedioxymethamphetamine (MDMA, commonly known as ecstasy). MAb6H4 bound (+)-MDMA (a drug of abuse administered as a racemic mixture) with a marginally higher affinity than METH. Besides (+)-MDMA, mAb6H4 showed negligible affinity for drugs like amphetamine or pseudoephedrine and no affinity for endogenous monoamines like dopamine or norepinephrine.
MAb6H4 was designed to have specificity for the (+)-METH enantiomer. This enantiomeric specificity was created by connecting the carrier protein to the para-position on the phenyl ring of METH distal to the chiral portion of the (+)-METH molecule (Peterson et al. 2007). Much of the illicit METH currently being produced contains both (+)-METH and (−)-METH, because it is synthesized using the phenyl-2-propenone production method (Maxwell and Brecht 2011). While the (−)-enantiomer of METH has some stimulant effects, it has a shorter duration of action and is less desirable to users than (+)-METH (Mendelson et al. 2006). Therefore, development of an anti-(+)-METH-specific mAb is the best strategy since production of antibodies against a racemic form of METH would lead to mAb binding of the less active, less addicting (−)-METH enantiomer. Binding to both enantiomers with high affinity could have a negative impact on the capacity of the mAb to bind the more active and highly addictive (+)-METH isomer.
Extensive preclinical testing of anti-METH mAb6H4 in rat models of METH abuse demonstrated efficacy in acute treatment scenarios. For example, in a METH overdose model, mAb6H4 (367 mg/kg) was administered 30 min after a 0.3, 1, or 3 mg/kg iv METH dose (0.3, 1, or 3 METH molecules per mAb binding site) followed by measurements of changes in METH-induced rearing events and distance traveled (Byrnes-Blake et al. 2003). In the 0.3 and 1 mg/kg METH groups, the number of rearing events and total distance traveled were both significantly reduced when compared to the baseline level of each METH dose before mAb treatment. Surprisingly, the two behavioral activity measurements were significantly increased in the mAb6H4-treated animals following a 3 mg/kg METH dose. This was initially a major concern since it suggested an additive or synergistic effect of high METH doses in the presence of antibody or that the antibody binding was perhaps serving as a sustained-release formulation for the METH. While these findings were difficult to understand at the time of the studies, it made us seek better direct measures and criteria for screening of sustained in vivo mAb function. It was also an early indication that anti-METH mAb6H4 had problems binding METH in vivo.
On the positive side, these data showed the mAb was capable of substantially increasing serum METH concentrations and immediately decreasing brain METH concentrations for at least 2.5 h. In a model of acute METH overdose, high-affinity mAb6H4 administration (367 mg/kg) was then compared to a very high dose (1000 mg/kg) of a lower-affinity mAb6H8 (METH K d = 250 nM). The mAb dosing occurred 30 min after a 1 mg/kg METH dose (Byrnes-Blake et al. 2005). Despite the substantially larger mAb6H8 dose, the mAb6H4 treatment resulted in significantly greater reduction in both METH-induced rearing (78 % vs. 27 %) and distance traveled (74 % vs. 35 %). These experiments provided evidence of the anti-METH mAb mechanism of action, the immediate anti-METH effects upon mAb administration, and the importance of high anti-METH mAb affinity for the attainment of anti-METH effects.
In other studies, mAb6H4 was administered prior to METH administration in an attempt to understand mAb effects on METH when given as a pretreatment. This pretreatment model was intended to simulate the use of the antibody for the clinical treatment of relapse to METH use in addicted patients.
In a pharmacokinetic study, mAb6H4 (503 mg/kg) was administered 17 h before a 1 mg/kg iv METH dose. This mAb6H4 dose was calculated to be equimolar in available mAb binding sites to the total body burden of METH administered (Laurenzana et al. 2003). This treatment dramatically increased area under the serum METH concentration-time curve (AUC) from 0 to 4.5 h. When compared to the AUC of vehicle control-treated rats after a 1 mg/kg METH dose, the AUC was 6600 % greater in the mAb6H4-treated rats. Importantly, brain METH AUC values in mAb6H4-treated rats were decreased by more than 60 %. Additionally, mAb6H4 significantly reduced METH concentrations in the kidney. It also showed that anti-METH mAb can reduce tissue concentrations, even though the mAb treatment did not decrease free METH concentrations in the serum compared to vehicle control. This lack of substantive changes in serum-free drug concentrations is a consistent finding in all of our studies of mAb treatment of METH and phencyclidine in rats, even though brain concentrations (and pharmacological effects) of the drugs of abuse are significantly lowered. We now know this high free drug concentration allows for the antibody binding capacity to be regenerated, as discussed earlier.
The next study was in a mAb pretreatment model designed to test the ability of high- and low-affinity anti-METH mAb to both protect against METH-induced effects and constantly reduce these METH effects over time. In these behavioral studies, rats were first preconditioned to repeated METH dosing until stable baseline values of locomotor activity were achieved (Byrnes-Blake et al. 2005). MAb6H4 (503 mg/kg) was then administered, followed by three 1 mg/kg METH challenges 1, 4, and 7 days later. Measurements of distance traveled and rearing behavior were determined immediately after each METH challenge dose. The much lower-affinity mAb6H8 (K d for METH = 250 nM) was also tested in an identical manner. The dose of mAb6H8 (1000 mg/kg) was greater than the mAb6H4 dose in an attempt to partially compensate for its lower affinity. MAb6H4 treatment produced a significant reduction in METH-induced distance traveled on the first day after the first mAb administration, but it ceased to have a significant effect on post-mAb days 4 and 7. The mAb6H4 reduction in rearing was significant on all 3 days, but it slowly decreased over the course of the three METH administrations. As expected, the low-affinity mAb6H8 was less effective than mAb6H4, even though it was administered at a higher dose.
In a broader study of mAb6H4 efficacy, METH-induced locomotor activity and rearing were measured along with hemodynamics in a model of chronic METH use. The doses of mAb6H4 were 175, 300, or 525 mg/kg. The challenge dose of 1 mg/kg METH was administered 1 and 4 days after mAb6H4 (Gentry et al. 2006). The doses of mAb6H4 were equivalent to 0.32, 0.56, or 1 mol of mAb binding sites for each METH molecule, respectively. The highest mAb6H4 dose produced significant reductions in the duration of locomotor activity, blood pressure, and heart rate effects from 2 to 3 h to about 1 h, and there were significant reductions in locomotor activity and the heart rate and blood pressure area under the hemodynamic effect vs. time curves. In addition, the time to peak locomotor activity was decreased after the 1 mol-eq. mAb6H4 dose compared to the lower mAb6H4 doses. MAb6H4’s protective effects only lasted for one METH challenge.
While extensive early pharmacokinetics, behavioral, and hemodynamic studies of mAb6H4 demonstrated the importance of mAb affinity as a prerequisite for therapeutic success in acute situations (McMillan et al. 2004; Byrnes-Blake et al. 2005), this series of studies also demonstrated the need for an antibody with a long-acting (e.g., weeks not days) METH binding function in vivo.
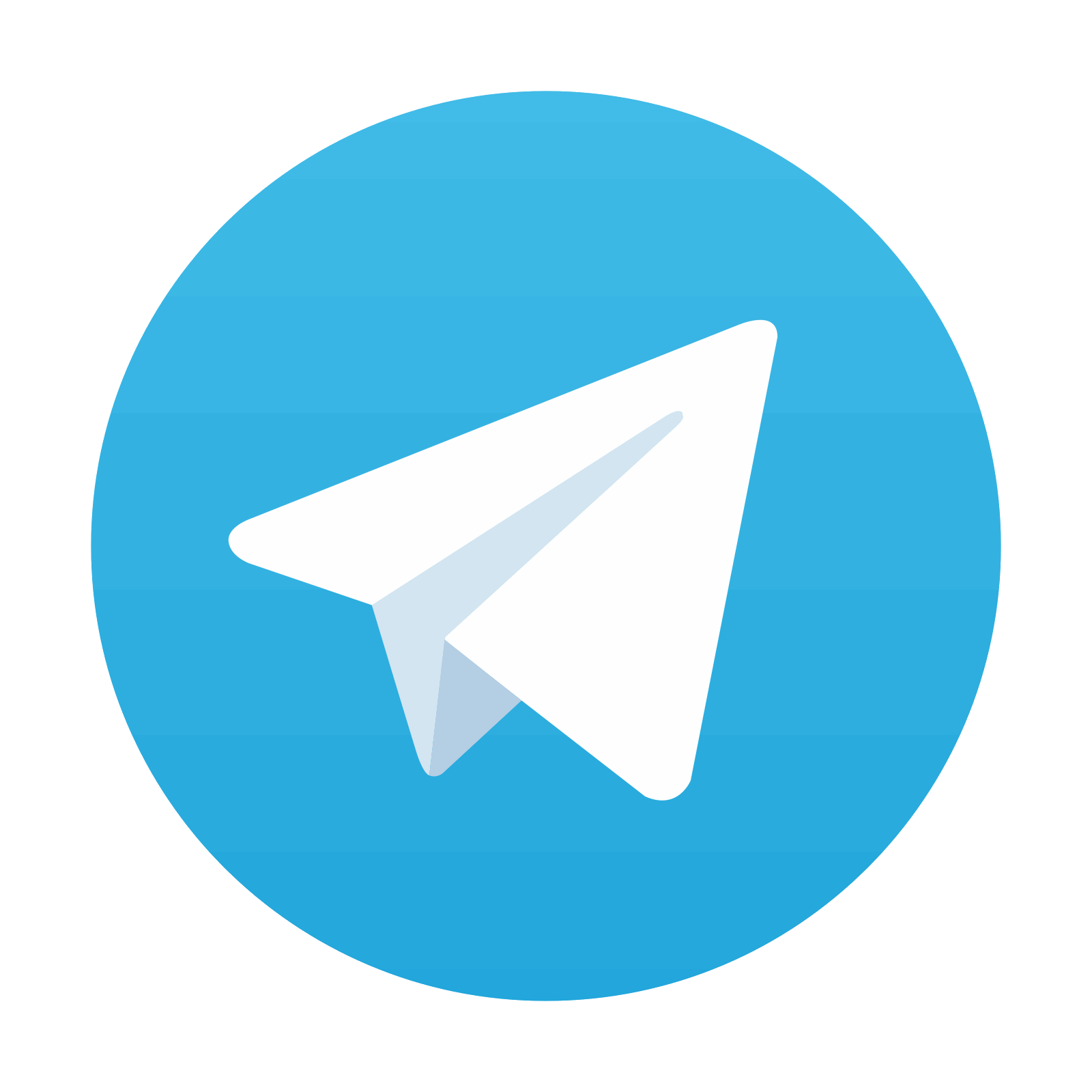
Stay updated, free articles. Join our Telegram channel

Full access? Get Clinical Tree
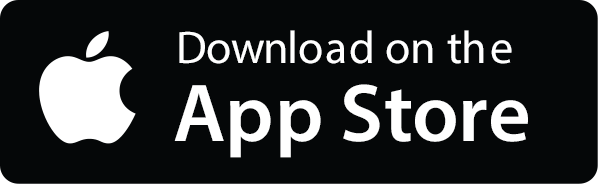
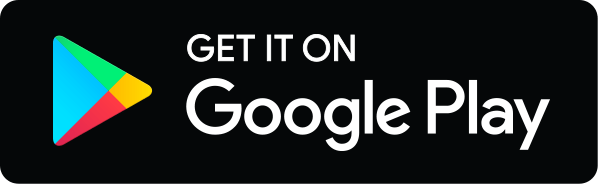