Glucose homeostasis
Blood [glucose] is maintained within a narrow range imposed at the lower limit by the undesirable effects of hypoglycaemia, and at the upper limit by the potential for loss in the urine if the renal threshold is exceeded. The liver plays a key role in maintaining blood [glucose]. After a carbohydrate-containing meal, it removes about 70% of the glucose load that is delivered via the portal circulation. Some of the glucose is oxidised and some is converted to glycogen for use as a fuel under fasting conditions. Glucose in excess of these requirements is partly converted by the liver to fatty acids and triglycerides, which are then incorporated into very low density lipoproteins (VLDLs) and transported to adipose tissue stores.
In the fasting state, blood [glucose] is maintained by glycogen breakdown in the liver in the short term, while glycogen stores last, and then by gluconeogenesis (from glycerol, lactate and pyruvate and from the gluconeogenic amino acids), occurring mostly in the liver, but also in the kidneys. Glucose is spared, under fasting conditions, by the ability of muscle and other tissues to adapt to the oxidation of fatty acids, and by the ability of the brain and some other organs to utilise ketone bodies that are formed under these conditions.
The hormones mainly concerned with regulating glucose metabolism in the fed and fasting states are insulin, glucagon, GH, adrenaline and cortisol. Of these, insulin has the most marked effects in humans (Table 6.1), and is the only hormone that lowers blood [glucose]. Glucagon, GH, adrenaline and cortisol all tend, in general, to antagonise the actions of insulin (Table 6.2).
Insulin secretion
Insulin is synthesised in the β-cells of the islets of Langerhans in the pancreas. It is formed as prepro-insulin, which is rapidly cleaved to pro-insulin. The pro-insulin is packaged into secretory granules in the Golgi apparatus and cleaved to insulin and C peptide. Insulin and C peptide are later released into the circulation in equimolar amounts.
Table 6.1 The effects of insulin on cellular metabolism
Tissue | Processes activated by insulin | Processes inhibited by insulin |
Liver | Uptake of amino acids and glycerol | Glycogenolysis |
Synthesis of glycogen, proteins, triglycerides and VLDLs | Gluconeogenesis | |
Ketone body formation | ||
Muscle | Uptake of glucose and amino acids | Triglyceride utilisation |
Synthesis of glycogen | Lipolysis | |
Adipose | Uptake of chylomicrons and VLDLs and of glucose | |
Utilisation of glucose |
Table 6.2 The effects on glucose metabolism of hormones that antagonise the actions of insulin
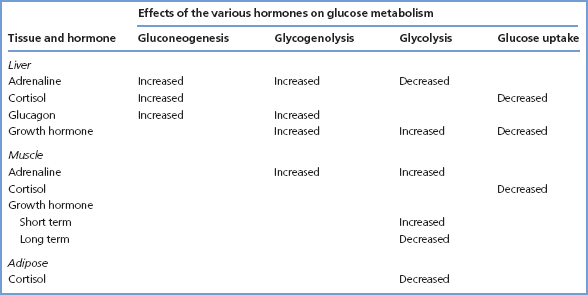
A rise in blood [glucose] is the main stimulus for insulin secretion. Some amino acids (e.g. leucine), fatty acids and ketone bodies also promote insulin secretion. Vagal stimulation also promotes insulin release. The release of insulin in response to hyperglycaemia is enhanced by the presence of incretin hormones released from the gastrointestinal tract (glucagon-like polypeptide-1 (GLP-1) and glucose-dependent insulinotropic polypeptide (GIP)). Incretins explain the larger release of insulin that occurs in response to an oral glucose load, compared with the same dose of glucose given intravenously. GLP-1 and GIP are inactivated in the circulation by the enzyme dipeptidyl peptidase-4 (DPP-4). Some new drugs for the treatment of type 2 diabetes enhance glucose-dependent endogenous insulin secretion by targeting this system. Both GLP-1 agonists and DPP-4 inhibitors are available.
The insulin receptor is located on the cell surface and is internalised after insulin binding. Within different organs, target enzymes have been identified that serve to explain the known effects of insulin on intermediary metabolism. For instance, activation of glucose transport, induction of hexokinase (or glucokinase) and activation of phosphofructokinase, pyruvate kinase and pyruvate dehydrogenase in the liver are all consistent with the actions of insulin in promoting increased glucose uptake and glycolytic breakdown. Stimulation of glycogen synthase accords with the effects of insulin on glycogen formation in the liver.
Diabetes mellitus
Diabetes is common, affecting 1–2% of Western populations, and population screening programmes reveal that many patients are previously unrecognised. It results in chronic hyperglycaemia, usually accompanied by glycosuria and other biochemical abnormalities, expressed as a wide range of clinical presentations ranging from asymptomatic patients with relatively mild biochemical abnormalities to patients admitted to hospitals with severe metabolic decompensation of rapid onset that has led to coma. Long-term complications may develop, including retinopathy, neuropathy and nephropathy. It is a major risk factor for cardiovascular disease.
Diabetes may be a secondary consequence of other diseases. For example, in diseases of the pancreas, such as pancreatitis or haemochromatosis, there is a reduction in insulin secretion. In some endocrine disorders, such as acromegaly or Cushing’s syndrome, there is antagonism of insulin action by abnormal secretion of hormones with opposing activity. Several drugs adversely affect glucose tolerance. Table 6.3 summarises these different causes. Secondary diabetes is, however, not common.
Most cases of diabetes are not associated with other conditions, but are primary, and fall into two distinct types. In type 1 diabetes, there is essentially no insulin secretion, whereas in type 2 diabetes insulin is secreted, but in amounts that are inadequate to prevent hyperglycaemia, or there is resistance to its action.
- Type 1 diabetes usually presents acutely over a period of days or a few weeks in young non-obese subjects, but can occur at any age. In addition to polyuria, thirst and glycosuria, there is often marked weight loss and ketoacidosis. Insulin is required for its treatment. Type 1 diabetes is an autoimmune condition with genetic and environmental precipitating factors in its pathogenesis. Islet-cell antibodies that react with the β-cells of the pancreas have been demonstrated in serum from over 90% of patients with newly diagnosed type 1 diabetes. They have also been demonstrated occasionally in serum several years before clinical and biochemical features of diabetes were developed. Most of the islet-cell antibodies are directed against the enzyme glutamic acid decarboxylase (GAD). Individuals with certain human leucocyte antigens (HLAs) have been shown to have a particularly high risk of developing type 1 diabetes. There is a well-recognised association between type 1 diabetes and other autoimmune endocri-nopathies, such as hypothyroidism and Addison’s disease, and also with pernicious anaemia.
- Type 2 diabetes generally presents in a less acute manner in older (>40 years) patients who are obese; many patients have clearly had the condition for some time (even years) before diagnosis. Rarely does type 2 diabetes occur in young patients. Measurable levels of insulin are present, and the metabolic defect appears to lie either in defective insulin secretion or in insulin resistance. In general, insulin is not required for the prevention of ketosis, as these patients are relatively resistant to its development. However, insulin may be needed to correct abnormalities of blood [glucose]. There appears to be no association between type 2 diabetes and either the HLA system or the development of autoimmunity. However, there is a strong genetic element to the disorder.
Table 6.3 Examples of causes of secondary diabetes or of IGT
Category of cause | Examples |
Drugs | Oestrogen-containing oral contraceptives, corticosteroids, salbutamol and some other catecholaminergic drugs, thiazide diuretics |
Endocrine disorders | Acromegaly, Cushing’s syndrome and Cushing’s disease, glucagonoma, phaeochromocytoma, prolactinoma, thyrotoxicosis (occasionally) |
Insulin receptor abnormalities | Autoimmune insulin receptor antibodies, congenital lipodystrophy |
Pancreatic disease | Chronic pancreatitis, haemochromatosis, pancreatectomy |
In established diabetic patients who become pregnant, poor blood glucose control is associated with a higher incidence of intrauterine death and foetal malformation. In addition, abnormal glucose tolerance or diabetes mellitus may develop during pregnancy, a condition referred to as ‘gestational diabetes mellitus’.
Monogenic forms of diabetes may account for 1–2% of cases of diabetes. Clinical features may overlap with those of type 1 or type 2 diabetes. Forms include:
- Neonatal diabetes is diabetes diagnosed within the first 6 months of life, and may be transient or permanent. Up to 60% of patients with permanent neonatal diabetes have a mutation in one of the potassium channel genes, which results in failure of insulin production. This is an important diagnosis since 90% of these patients can stop insulin and achieve good control on sulphonylureas.
- Maturity onset diabetes of the young (MODY) may be better called familial diabetes and may be suspected in a patient with a strong family history of diabetes or when apparent type 2 diabetes occurs at a relatively young age, or in the absence of typical type 2 features such as insulin resistance. Patients may be on insulin. A number of subtypes are recognised, the more common ones being caused by mutations in:
- Glucokinase. This often causes mild asymptomatic hyperglycaemia typically only picked up during screening. Diabetic complications are very unusual and treatment can usually be stopped.
- Hepatic nuclear factor 1 alpha (HNF-1α). This is the most common cause of MODY. [Glucose] deteriorates with age and becomes symptomatic in adolescence or young adulthood. Diabetic complications occur, so treatment is needed. Low dose sulphonylureas achieve excellent [glucose] lowering and in patients less that 45 years old may even replace insulin if that has been started before the correct diagnosis was made.
- Glucokinase. This often causes mild asymptomatic hyperglycaemia typically only picked up during screening. Diabetic complications are very unusual and treatment can usually be stopped.
Diagnosis
The diagnosis of diabetes mellitus has serious consequences. It confers a risk of long-term diabetic complications, including blindness, renal failure and amputations, as well as an increased risk of cardiovascular disease. It also means a lifetime of dietary restriction and medications and can seriously curtail lifestyle and employment prospects. The diagnosis may be suggested by the patient’s history, or by the results of dipstick tests for glucose on urine specimens. However, urine glucose measurements by themselves are inadequate for diagnosing diabetes. They potentially yield false-positive results in subjects with a low renal threshold for glucose, and in a patient with diabetes they may yield false-negative results if the patient is fasting. A provisional diagnosis of diabetes mellitus must always be confirmed by glucose measurements on blood specimens.
The most recent criteria for the diagnosis of diabetes mellitus have been laid down by the World Health Organization (WHO) in 2006, and by the American Diabetes Association in 2003. These are broadly similar but differ in some details. It is likely that the precise requirements for the diagnosis of diabetes and the states of impaired glucose regulation will continue to evolve as knowledge of the relationship between glucose regulation and the future development of complications accumulates. The following descriptions adhere to the WHO recommendations. Separate criteria are described depending on whether venous or capillary whole blood, or venous or capillary plasma specimens are used. In practice, results for this important diagnosis will usually come from a clinical laboratory and use venous plasma. According to the criteria, a random venous plasma [glucose] of 11.1 mmol/L or more, or a fasting plasma [glucose] of 7.0 mmol/L or more, establishes the diagnosis. A single result is sufficient in the presence of typical hyperglycaemic symptoms of thirst and polyuria. In their absence, a venous plasma [glucose] in the diabetic range should be detected on at least two separate occasions on different days. Where there is any doubt, an OGTT should be performed (see below), and if the fasting or random values are not diagnostic, the 2-h value should be used. In practice, the diagnosis is often obvious clinically, and [glucose] is only needed for confirmation and is unequivocally high. The diagnosis should never be made on the basis of a single test in a patient without symptoms.
A raised glycated haemoglobin (HbA1c) should not be used to make a diagnosis of diabetes since it is influenced by red cell lifespan as well as by [glucose].
Blood and plasma glucose
Most laboratory instruments measure plasma [glucose], but some use whole blood. Plasma [glucose] is 10–15% higher than whole blood [glucose], since red cells contain less water per unit volume than plasma. The discrepancy can be greater than this if [glucose] changes rapidly, because glucose will not have reached equilibration across the red cell membrane. Plasma, therefore, yields more reliable results. At normal plasma [glucose], there is little difference between results obtained on capillary and venous blood. However, at hyperglycaemic levels, capillary plasma [glucose] may be significantly higher than venous plasma [glucose]. These factors are important in the interpretation of glucose tolerance tests.
If there is likely to be any delay in measuring [glucose] in blood specimens, it is essential either to separate the plasma immediately or to inhibit glycolysis in blood by using a sodium fluoride-containing collection tube. This stabilises the [glucose] for several hours. Measurements of blood [glucose] that are to be performed using a ‘stick’ test must be carried out without delay on specimens that do not contain sodium fluoride.
Oral glucose tolerance test (OGTT)
The OGTT remains the ‘gold standard’ for the diagnosis of diabetes and should be performed when random [glucose] falls into a range where the diagnosis is uncertain. Several precautions must be taken in preparing for and performing the test. It should not be performed on patients suffering from an intercurrent infection or the effects of trauma, or those recovering from a serious illness. Drugs such as corticosteroids and diuretics may impair glucose tolerance and should be stopped before the test if possible. The patient should have been on an unrestricted diet containing at least 150g of carbohydrate/day for at least 3 days, and should not have indulged in unaccustomed amounts of exercise. The patient must not smoke before or during the test, nor eat or drink anything other than as specified below.
An OGTT is usually performed after an overnight fast, although a fast of 4–5h may be enough. The patient is allowed to drink water during the fast. A standard dose of glucose (82.5g of glucose monohydrate or 75g of anhydrous glucose) dissolved in 250–300 mL of water is given by mouth over a 5-min period. Smaller amounts of glucose (1.92 g of glucose monohydrate or 1.75 g of anhydrous glucose/kg body weight) are given to children. During the test, the patient should be sitting up or lying down on the right side so as to facilitate rapid emptying of the stomach. Blood specimens are collected before giving the glucose load and after 2h.
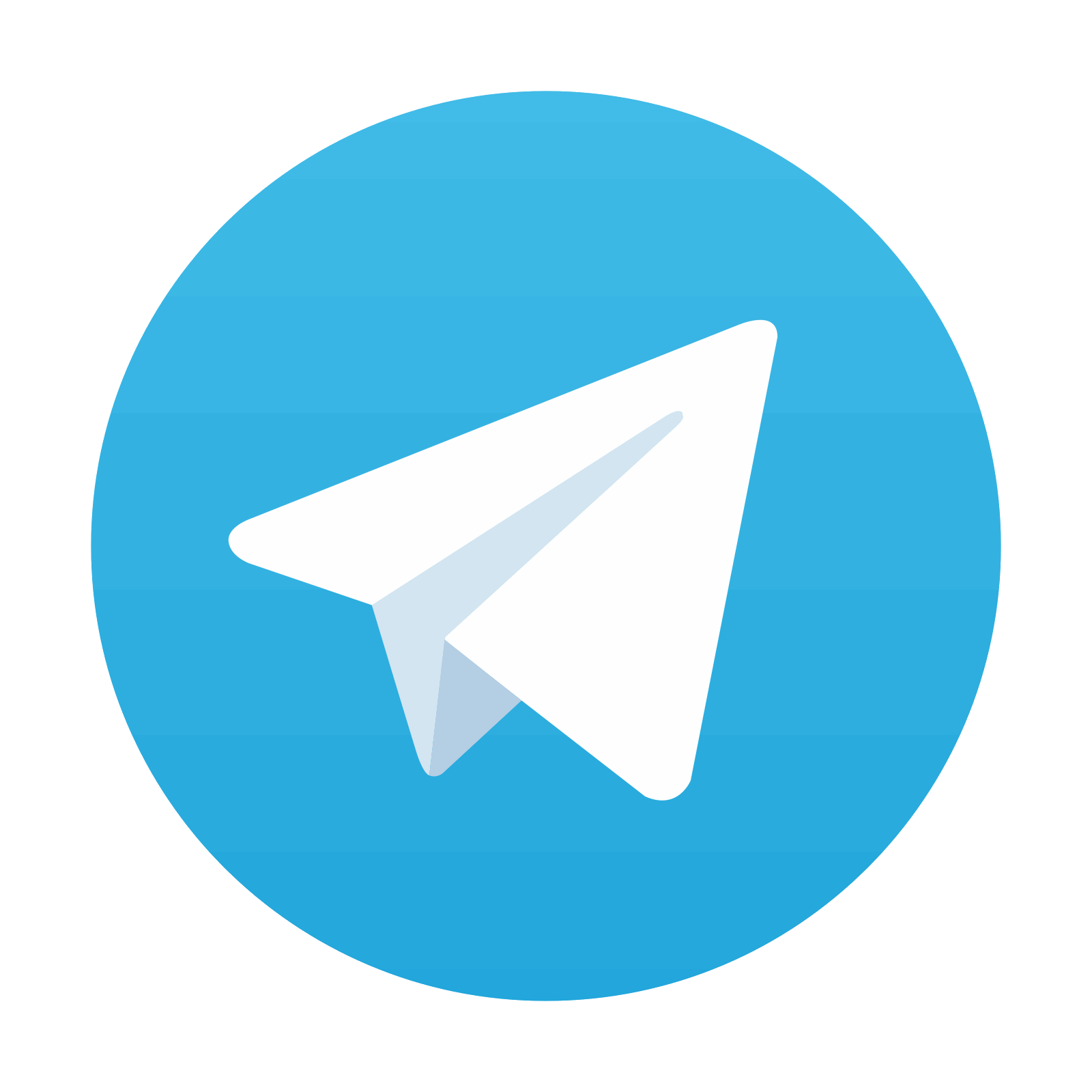
Stay updated, free articles. Join our Telegram channel

Full access? Get Clinical Tree
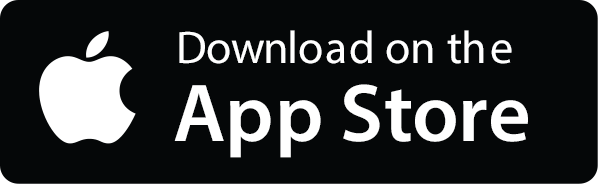
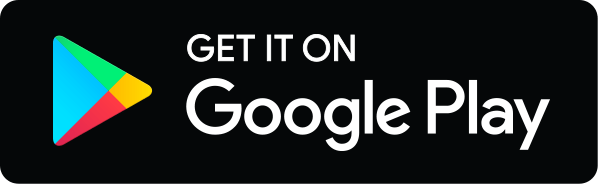