enhanced glucose-dependent insulin secretion; incretins are probably responsible for about 60% of the insulin that is secreted in response to a meal,
The actions of GLP-1 are brief as it has a very short plasma half-life of 1–2 min due to rapid degradation by dipeptidyl peptidase-4 (DPP-4).
Insulin is secreted into the blood under fasting conditions, with pulses every 10–14 min, and a slower cycle of release every 105–120 min. In response to a rise in plasma glucose (both the actual concentration and the rate of change) there is a superimposed biphasic pattern of insulin release.

Insulin secretion from pancreatic β-cells is modulated by K+ channels in the cell membrane that are sensitive to ATP (KATP channels). The KATP channel has subunits known as sulfonylurea receptors (SURs), various isoforms of which act as regulatory proteins in the response to ATP in different tissues. First-phase insulin release is triggered when glucose enters the β-cell via the GLUT2 glucose transporter, and undergoes glycolysis with generation of intracellular ATP. Activation of the SUR1 receptor isoform by ATP closes the KATP channel, which reduces membrane K+ efflux and depolarises the β-cell. Depolarisation opens voltage-gated L-type Ca2+ channels in the cell membrane, and an influx of Ca2+ ions into the cell triggers second messengers that lead to exocytosis of insulin granules. (See Fig. 5.4 for description of the KATP channel in vascular smooth muscle.) In addition to glucose, many other factors influence insulin secretion (Table 40.1).
Peripheral tissues express specific cell surface insulin receptors that are linked to a tyrosine kinase (insulin receptor kinase) (Ch. 1). Stimulation of these receptors leads to translocation of the GLUT4 glucose transporter to the cell surface, allowing glucose uptake, and activates pathways involved in glycogen synthesis, glycolysis and fatty acid synthesis. Metabolic effects of insulin include the following.


The effects of insulin on different tissues are summarised in Table 40.2.
Table 40.2
Site | Effect |
Liver | Increased glucose storage as glycogen |
Decreased protein catabolism | |
Increased protein synthesis | |
Decreased gluconeogenesis | |
Muscle | Increased protein synthesis |
Increased glycogen synthesis | |
Increased glucose uptake | |
Increased amino acid uptake | |
Adipose tissue | Increased triglyceride storage |
Increased triglyceride synthesis | |
Decreased lipolysis |
Several hormones inhibit the anabolic actions of insulin, particularly on carbohydrate metabolism, although effects on protein metabolism vary. These include glucagon, growth hormone, cortisol and catecholamines. Most of these hormones are released in stressful situations that require the breakdown of glycogen reserves to provide energy.
Diabetes mellitus
Failure to secrete sufficient insulin to control the normal level of blood glucose results in diabetes mellitus. The condition is diagnosed when the fasting plasma glucose concentration exceeds 7 mmol⋅L−1. The long-term consequences include increased risk of the development of vascular and neuropathic disease (Table 40.3). Two patterns of diabetes mellitus are recognised: type 1 and type 2. There is still dispute over whether they represent distinct entities, or different manifestations of the same disease process. There is a strong genetic predisposition for both conditions.
Type 1 diabetes mellitus
Type 1 diabetes represents a severe deficiency of insulin production caused by autoimmune destruction of pancreatic β-cells, and usually presents in younger people. Type 1 diabetes typically presents with a short history of feeling tired and unwell, together with weight loss, polyuria and polydipsia. There is a high risk of ketoacidosis because of breakdown of fatty acids and amino acids in the liver to provide an energy source, which generates ketone bodies.
Type 2 diabetes mellitus
Type 2 diabetes, which usually presents later in life, is the consequence of a relative deficiency of insulin. It accounts for 90% of cases of diabetes in the Western world. In established type 2 diabetes the first phase of insulin secretion is absent or attenuated, and the second phase is slowed.
People with type 2 diabetes are often overweight (the average body mass index at diagnosis is 30 kg⋅m−2). This increases cellular resistance to insulin in the liver, muscles and adipose tissue so that less glucose is transported into cells. In addition to reduced tissue uptake of glucose, glycogen in the liver is broken down and glucose is released into the circulation. In adipose tissue, triglycerides are broken down to free fatty acids which are released into the circulation.
In type 2 diabetes there is reduced or absent GLP-1 secretion in response to oral glucose, and a reduced sensitivity to the peptide at pancreatic β-cells. In addition, high circulating free fatty acids and the production of reactive oxygen species in response to a sustained high plasma glucose concentration reduce insulin secretion.
Insulin resistance characteristically precedes overt diabetes by several years, but for some time insulin secretion by the pancreas is sufficient to overcome cellular resistance. Eventually, β-cell dysfunction with loss of the first-phase insulin response to a glucose load results in loss of compensation for insulin resistance. In people with type 2 diabetes who are not obese the major defect is inadequate insulin secretion.
In type 2 diabetes, postprandial hyperglycaemia is the major defect in blood glucose control, with excess glucose outside the cells rather than a shortage inside. People with type 2 diabetes do not usually develop ketoacidosis, because sufficient glucose enters cells to permit adequate energy production for most situations. The ideal approach to treatment would be an intervention that restores the early phase of insulin secretion in response to a glucose load.
Insulins and insulin analogues
Normal insulin secretion from the pancreas is into the portal circulation and is strictly regulated to meet metabolic needs. Sixty per cent of the insulin that is released from the pancreas is extracted by the liver before it reaches the systemic circulation. In contrast, therapeutic delivery of insulin is to the systemic circulation, and the relationship to metabolic needs can only be approximated by the dosages used and their timing in relation to meals.
Natural insulin formulations
Insulins for therapeutic administration were originally extracted from either bovine or porcine pancreas. Bovine insulin differs chemically from human insulin in three amino acid residues, and porcine in one, but their actions are very similar to human insulin. These insulins are now rarely used.
Human-sequence insulin is produced either by enzymatic modification of porcine insulin, or by recombinant DNA technology using bacteria or yeast. All current insulin preparations have a low impurity content, which has caused problems in the past, and have low immunogenic potential.
All insulins (and insulin analogues; see below) are formulated at a standard strength of 100 units⋅mL−1 to reduce confusion over doses. The choice of injection device, usually a form of prefilled syringe, is important to facilitate use.
Pharmacokinetics
Currently available insulins must be given parenterally, because insulin is a protein and would otherwise be digested in the gut. At present, subcutaneous injection is used for routine treatment, with intravenous infusion for emergency situations. Recommended subcutaneous injection sites include upper arms, thigh, buttocks and abdomen. Absorption is faster from the abdomen than from the limbs, although strenuous exercise can increase absorption from the limbs.
The half-life of insulin in plasma is very short (about 8–16 min), and to avoid the need for frequent injections during maintenance treatment the absorption of insulin from injection sites must be prolonged. Insulin is formulated either in a soluble preparation or complexed with a substance to delay absorption from the injection site (Table 40.4).
Table 40.4
Characteristics of insulins following subcutaneous administration
aSometimes called NPH (neutral protamine Hagedorn) insulin.
Soluble insulins aggregate to form hexamers, which delays their absorption from the injection site. After subcutaneous injection, the maximum plasma concentration of soluble insulin (also called neutral insulin) is achieved about 2 h later, compared with minutes after intravenous injection. To limit the increase in plasma glucose concentration generated by a meal, subcutaneous soluble insulin must be given 15–30 min before eating. The action of intravenous soluble insulin lasts less than an hour and is mainly terminated by degradation in the kidney. Continued absorption from a subcutaneous injection site prolongs the duration of action after injection to about 5 h.
To generate intermediate- or long-acting formulations, insulin is complexed with the following.


Unwanted effects



Insulin analogues
Mechanism of action and effects
The insulin analogues are recombinant chemical modifications of naturally occurring insulin. These changes have no effect on the binding of the molecule to cellular insulin receptors.
Short-acting insulin analogues: Unlike soluble insulins, these do not readily form dimers and hexamers, and therefore they are rapidly absorbed from an injection site with a faster onset and a shorter duration of action.

Insulin aspart and lispro are available complexed with protamine to give an intermediate duration of action, and in this form are combined with the short-acting formulations as ready mixed biphasic insulins.
Long-acting insulin analogues:


Pharmacokinetics
Compared with standard soluble insulin, absorption of short-acting insulin analogues from a subcutaneous injection site occurs faster and leads to an early peak plasma concentration (Table 40.4). The duration of action is also shorter, at almost 3 h. They are usually given just before a meal, but can be used immediately after eating. They can be mixed with long-acting standard insulins, and are also available as biphasic formulations (insulin aspart with insulin aspart protamine in a 30 : 70 ratio; insulin lispro with insulin lispro protamine in a 25 : 75 or 50 : 50 ratio). Insulin analogues can be given by subcutaneous injection or infusion or by intravenous injection or infusion.
Long-acting insulin analogues are slowly and uniformly absorbed after subcutaneous injection, which avoids plasma insulin peaks.
Therapeutic regimens for insulin
The choice of regimen for insulin administration depends on the age, lifestyle, circumstances and preference of the individual. The general principle is to maintain a background (basal) level of insulin and then to give insulin boluses prior to meals to deal with the glucose load (basal-bolus regimens). Options include the following.



There are also situations in which a basal-bolus regimen is not appropriate.



Other parenteral hypoglycaemic drugs
Mechanism of action
Exenatide and liraglutide are peptides that share part of their amino acid sequences with the naturally occurring incretin, glucagon-like peptide-1 (GLP-1). They bind to and activate the GLP-1 receptor, leading to an increase in glucose-dependent synthesis of insulin and its secretion from β-cells. They restore the first-phase insulin response to an oral glucose load and, unlike insulin, promote weight loss. Unlike GLP-1 they are resistant to the enzymatic action of DPP-4.
Pharmacokinetics
Exenatide and liraglutide are given by subcutaneous injection. Exenatide is eliminated by the kidney and has a short half-life of about 2 h. Liraglutide is eliminated by proteolysis and has a half-life of 11–15 h.
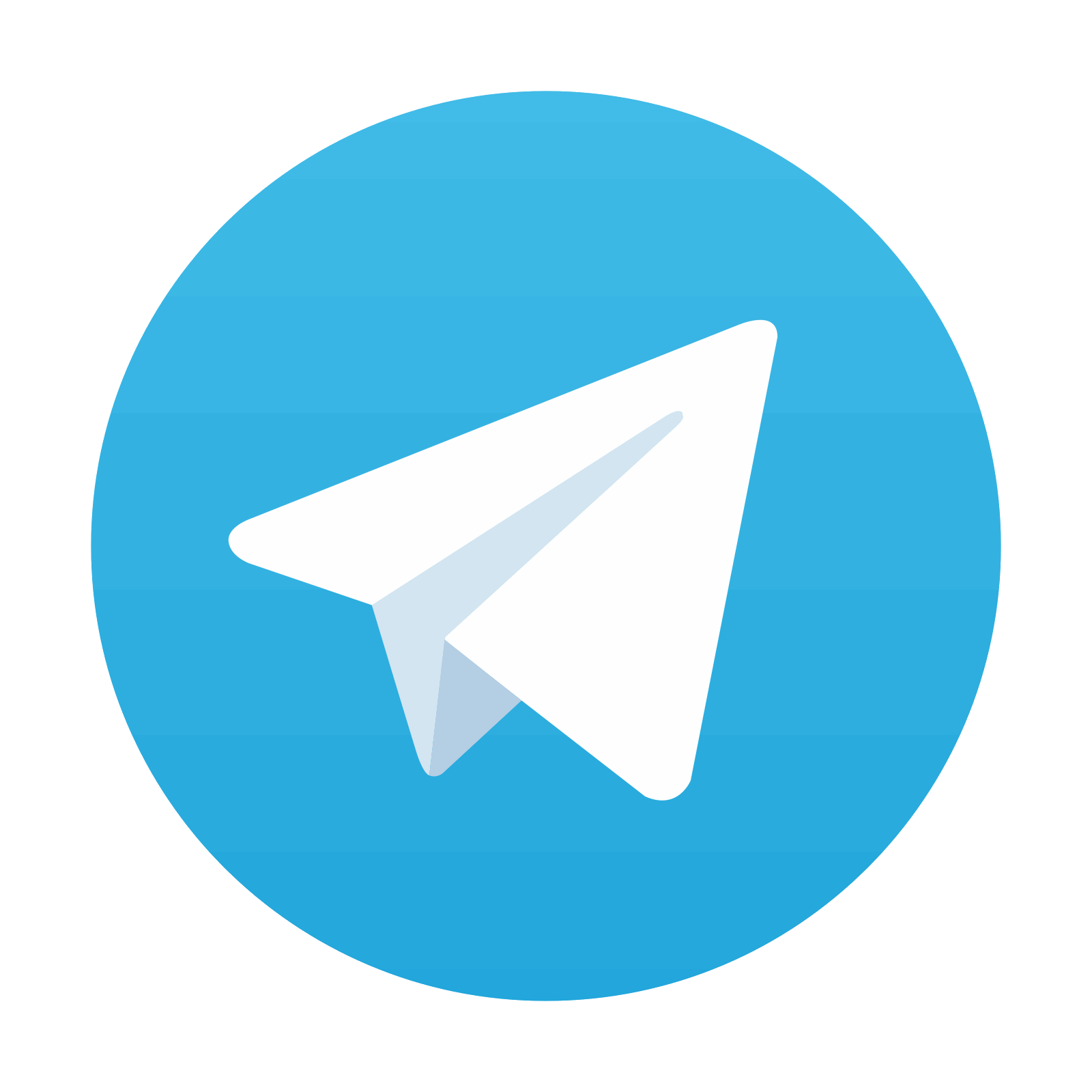
Stay updated, free articles. Join our Telegram channel

Full access? Get Clinical Tree
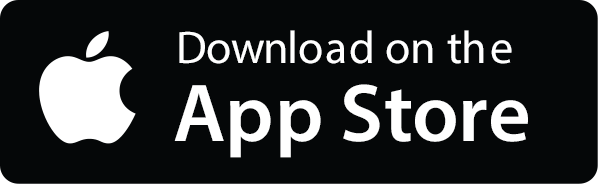
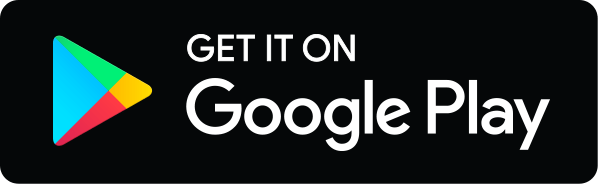