The CFTR chloride channel has five domains, shown in Figure 12-15: two membrane-spanning domains, each with six transmembrane sequences; two nucleotide (ATP)-binding domains; and a regulatory domain with multiple phosphorylation sites. The importance of each domain is demonstrated by the identification of CF-causing missense mutations in each of them (see Fig. 12-15). The pore of the chloride channel is formed by the 12 transmembrane segments. ATP is bound and hydrolyzed by the nucleotide-binding domains, and the energy released is used to open and close the channel. Regulation of the channel is mediated, at least in part, by phosphorylation of the regulatory domain.
The Pathophysiology of Cystic Fibrosis.
CF is due to abnormal fluid and electrolyte transport across epithelial apical membranes. This abnormality leads to disease in the lung, pancreas, intestine, hepatobiliary tree, and male genital tract. The physiological abnormalities have been most clearly elucidated for the sweat gland. The loss of CFTR function means that chloride in the duct of the sweat gland cannot be reabsorbed, leading to a reduction in the electrochemical gradient that normally drives sodium entry across the apical membrane. This defect leads, in turn, to the increased chloride and sodium concentrations in sweat. The effects on electrolyte transport due to the abnormalities in the CFTR protein have also been carefully studied in airway and pancreatic epithelia. In the lung, the hyperabsorption of sodium and reduced chloride secretion result in a depletion of airway surface liquid. Consequently, the mucous layer of the lung may become adherent to cell surfaces, disrupting the cough and cilia-dependent clearance of mucus and providing a niche favorable to Pseudomonas aeruginosa, the major cause of chronic pulmonary infection in CF.
The Genetics of Cystic Fibrosis
Mutations in the Cystic Fibrosis Transmembrane Regulator Polypeptide.
The most common CF mutation is a deletion of a phenylalanine residue at position 508 (ΔF508) in the first ATP-binding fold (NBD1; see Fig. 12-15), accounting for approximately 70% of all CF alleles in white populations. In these populations, only seven other mutations are more frequent than 0.5%, and the remainder are each quite rare. Mutations of all types have been identified, but the largest single group (nearly half) are missense substitutions. The remainder are point mutations of other types, and less than 1% are genomic rearrangements. Although nearly 2000 CFTR gene sequence variants have been associated with disease, the actual number of missense mutations that are disease-causing is uncertain because few have been subjected to functional analysis. However, a new project called the Clinical and Functional Translation of CFTR (CFTR2 project; cftr2.org) has succeeded in assigning pathogenicity to more than 125 CFTR mutations, which together account for at least 96% of all CFTR alleles worldwide.
Although the specific biochemical abnormalities associated with most CF mutations are not known, six general classes of dysfunction of the CFTR protein have been identified to date. Alleles representative of each class are shown in Figure 12-15.
• Class 1 mutations are null alleles—no CFTR polypeptide is produced. This class includes alleles with premature stop codons or that generate highly unstable RNAs. Because CFTR is a glycosylated membrane-spanning protein, it must be processed in the endoplasmic reticulum and Golgi apparatus to be glycosylated and secreted.
• Class 2 mutations impair the folding of the CFTR protein, thereby arresting its maturation. The ΔF508 mutant typifies this class; this misfolded protein cannot exit from the endoplasmic reticulum. However, the biochemical phenotype of the ΔF508 protein is complex, because it also exhibits defects in stability and activation in addition to impaired folding.
• Class 3 mutations allow normal delivery of the CFTR protein to the cell surface, but disrupt its function (see Fig. 12-15). The prime example is the Gly551Asp mutation that impedes the opening and closing of the CFTR ion channel at the cell surface. This mutation is particularly notable because, although it constitutes only approximately 2% of CFTR alleles, the drug ivacaftor has been shown to be remarkably effective in correcting the function of the mutant Gly551Asp protein at the cell surface, resulting in both physiological and clinical improvements (see Chapter 13).
• Class 4 mutations are located in the membrane-spanning domains and, consistent with this localization, have defective chloride ion conduction.
• Class 5 mutations reduce the number of CFTR transcripts.
• Class 6 mutant proteins are synthesized normally but are unstable at the cell surface.
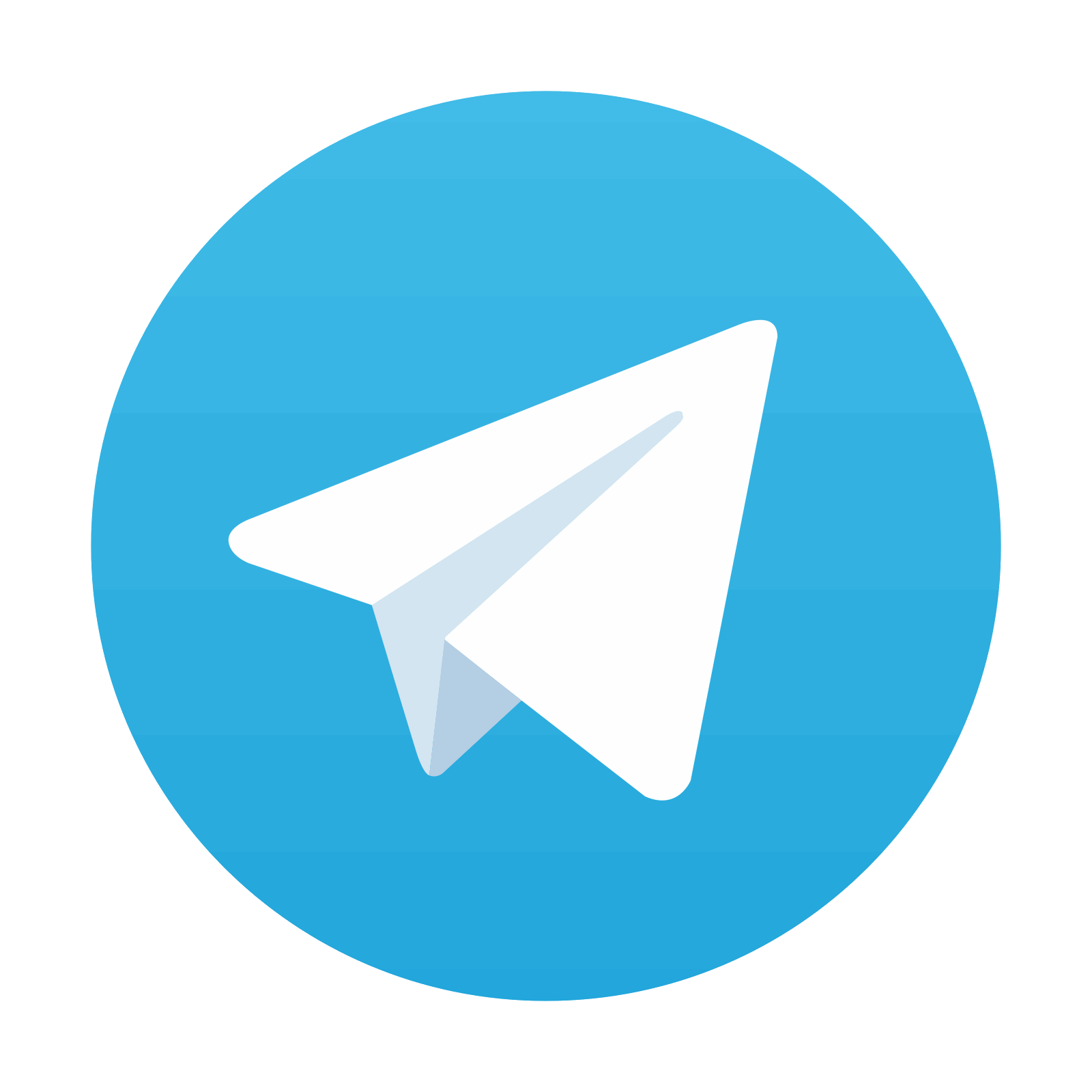
Stay updated, free articles. Join our Telegram channel

Full access? Get Clinical Tree
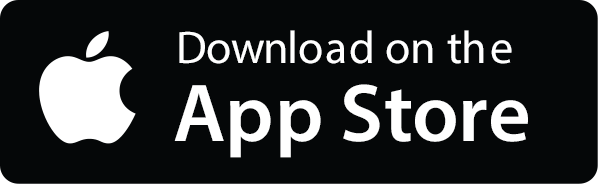
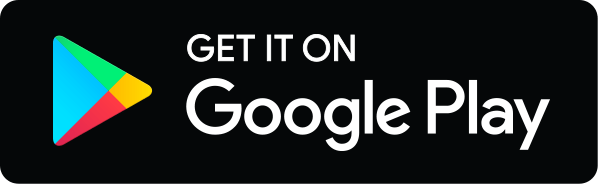