Cyanide
Rebeca Gracia Pharm. D.
THEORETICAL AND SCIENTIFIC BACKGROUND
HISTORY
Cyanide was used as a poison for centuries before the chemical was isolated and identified. Ancient records from Egypt make reference to poisoning from a natural food source of cyanide in reports detailing “the penalty of the peach” (1). The use of cyanide also dates back to the first century where, in Rome, the Emperor Nero supposedly used the cyanogenic plant cherry laurel to poison opponents. The Swedish scientist Scheele first chemically isolated cyanide in 1782, and it has been reported that he was the first person to become a victim of the purified chemical four years later in a laboratory accident (2). Historically, cyanide has been a relatively uncommon agent of warfare, although it is highly lethal. Napoleon III suggested its use as a weapon by coating bayonets in the 1870 Franco-Prussian war. The French military made an unsuccessful attempt at utilizing hydrocyanic acid early during World War I but, through continued experimentation, finally did create the more effective cyanogen chloride. The rate of development of chemical weapons increased greatly during the following years with subsequent design and use of additional cyanogens. Most notoriously, Zyklon B was the form of cyanide reportedly used in Nazi death camps during World War II. Zyklon B was hydrocyanic acid adsorbed onto a pharmaceutical base that was initially developed as a pesticide and rodenticide (3a). Although cyanide has not frequently been used for military purposes, several attempts have been made to utilize it as a poison and contaminant (Fig. 3-1). As a chemical weapon, it is not easy to disseminate upon a large number of people. However, cyanide is both widely available and easily accessible throughout the world, making it very attractive as a potential terrorist agent (4). It also possesses the ability to cause significant social disruption and public panic and demands special attention to public health preparedness (i.e., antidote stocking). Although cases of
cyanide toxicity from industrial sources and poisonings are currently the most commonly encountered exposures, the potential impact of an attack with cyanide warrants preparation. Health care providers should be equipped with the knowledge and resources necessary to manage a mass casualty incident involving this agent.
cyanide toxicity from industrial sources and poisonings are currently the most commonly encountered exposures, the potential impact of an attack with cyanide warrants preparation. Health care providers should be equipped with the knowledge and resources necessary to manage a mass casualty incident involving this agent.
SOURCES
Cyanide is most likely to be used in the volatile, water-soluble, and liquid forms of hydrogen cyanide and cyanogen chloride (NATO designation AC and CK, respectively). Hydrogen cyanide (HCN) may also be referred to as hydrocyanic acid, blauseare (German for Berlin blue acid), and prussic acid (due to its origination from Prussian blue). The highly reactive salt forms are exploited for numerous industrial applications including chemical synthesis, electroplating, tanning, metallurgy (especially silver and gold), printing, agriculture, photography, and manufacture of paper and plastics, as well as fumigants and insecticides. Water-soluble salt forms such as calcium cyanide (CaCN), sodium cyanide (NaCN), and potassium cyanide (KCN) are most often utilized. These salts produce HCN gas when mixed with strong acid and thus pose a significant risk in industrial accidents as well as intentional exposures (5,6). The insoluble salt forms, mercury cyanide (HgCN), copper cyanide (CuCN), gold cyanide (AuCN), and silver cyanide (AgCN), also can be found in the industrial setting (7). Waste products from mining processes produce vast amounts of cyanide complexes including ferrocyanide. These chemicals are significantly less toxic than their salt counterparts; however, they do pose a considerable risk to the environment due to accumulation (8). The use of these cyanide waste products as terrorist agents may be appealing due to their abundance and relative ease of acquisition. In fact, ferrocyanide was implicated in the aborted attempt to poison a water supply in Rome in 2002.
A noteworthy form of cyanide is the chemical group referred to as nitriles (R-CN); acetonitrile and propionitrile are the most frequently encountered forms (9,10,11,12,13,14,15). Nitriles are easily accessible over the counter as acrylic nail and glue removers. These chemicals are also commonly encountered in industry as solvents. As parent compounds, nitriles are not significantly toxic, but slowly release cyanide into the body as they are metabolized. This occurs via cytochrome p450 (cyp 450) enzyme 2E1 where the resulting cyanohydrin metabolite then is converted into the actively toxic cyanide molecule (16,17,18). Exposure to these organic cyanides results in a delay in the onset of signs and symptoms as a latent period of several hours passes during transformation and liberation of the toxin. This delay has been implicated in several case reports of missed diagnosis and fatal outcomes and poses a significant risk to responding personnel who may not accurately perceive the risk in the situation.
Structural fires, as a source of cyanide, pose a looming threat in any disaster situation. HCN gas may be released in the combustion of many synthetic polymers, such as nylon and plastics, as well as natural materials including wool and silk. Any material that contains carbon and nitrogen may release cyanide during pyrolysis (19). Victims of fire inhalation especially from domestic fires are at an additional risk for cyanide toxicity as well as carbon monoxide effects (20). Because cyanide has a considerably limited residential time in the blood, levels may be decreased significantly by the time the victim arrives to a health care facility. Both carbon monoxide and cyanide will cause hypoxic damage and the effects will be additive and possibly synergistic (21). Cyanide toxicity may be more contributory to death in some fire victims than the carbon monoxide itself (22). Early initiation of empiric treatment and management of probable cyanide exposure may be warranted in many cases of fire exposures (23,24,25,26,27).
Medically important sources of iatrogenic cyanide toxicity include nitroprusside (Nipride) and Laetrile. Each molecule of nitroprusside can release up to four or five CN groups, and patients may develop toxicity as cyanide accumulates. It is recommended to avoid prolonged administrations of high dose nitroprusside (28,29). Laetrile was used as a chemotherapeutic medication and is the purified form of the natural cyanide compound, amygdalin. When these products are ingested, the enzyme beta-glucosidase in the gastrointestinal tract metabolizes the amygdalin into hydrogen cyanide, resulting in toxicity. Many case reports have been published on the devastating use of Laetrile (30,31,32,33).
Additional sources of cyanide include tobacco smoke and food sources, although these rarely pose risk as acute toxicity (34). Smokers can average about 0.17 μg/ml of cyanide in their blood as compared to 0.06 μg/ml of cyanide in nonsmokers. Food sources of the cyanogenic glycoside, amygdalin, include apple seeds and fruit pits from the Prunus sp. Of these, the bitter almonds and apricot pits contain the highest concentrations. Food sources rarely cause toxicity as they must be consumed in large amounts or in purified forms to accumulate enough cyanide. Dietary staples such as cassava and lima beans have been implicated in chronic cyanide poisonings (37). Not only can long-term exposure to large amounts of these cyanide-containing food sources result in toxicity, but acute cyanide toxicity can occur from exposure to the wastewater from these food-processing factories (38,39).
CHEMICAL CHARACTERISTICS
Key features determining the effectiveness of a chemical as a warfare agent include not only the toxicity of the specific agent but also chemical characteristics such as volatility, persistence, and latency (40,41,42). Volatility is the tendency of a liquid to evaporate and form a vapor or gaseous form. Cyanide is one of the most volatile chemical warfare agents and as a liquid can readily transform into a more potent gas form. HCN is extremely volatile to such an extent as to limit its use as an effective weapon because it is, in fact, lighter than air. The HCN gas too readily dissipates and is difficult to deliver in high concentrations unless in an enclosed space. Cyanogen chloride was specifically formulated to be slightly heavier than air and provide a more persistent weapon. Persistence is inversely related to volatility; a balance is needed between these chemical characteristics in order to result in the most effective distribution. Agents with increased persistence are also more likely to remain on site available for contamination, penetrate the skin, and pose the greatest risk for rescue and medical personnel. Latency is the time delay between
absorption of the agent and onset of symptoms. Cyanide can cause symptoms within seconds to minutes, or the presentation may be delayed up to hours depending on the form. Agents with prolonged latency times not only pose a risk to exposed victims who may go undiagnosed and untreated but also pose an increased risk to responding teams who may not be aware of the need for decontamination.
absorption of the agent and onset of symptoms. Cyanide can cause symptoms within seconds to minutes, or the presentation may be delayed up to hours depending on the form. Agents with prolonged latency times not only pose a risk to exposed victims who may go undiagnosed and untreated but also pose an increased risk to responding teams who may not be aware of the need for decontamination.
TOXIC DOSE
Cyanide is notorious for its high degree of lethality. This potency is a product of its rapid diffusion into tissues and irreversible binding to target sites. It is the rapidity of action rather than the minuteness of a lethal dose that makes cyanide effective as a chemical weapon. The toxic dose of cyanide is relatively high when compared to other agents used in chemical warfare. Because the most likely exposure to cyanide is via inhalation rather than the intravenous route, the lethal dose is expressed in a product of the concentration (C) of the agent in the air and exposure time (t). The amount determined to be lethal to 50% of a population is signified as the LCt50. The LCt50 for HCN is 2,500 to 500 mg × min/m3 and the LCt50 for CK is 1.1g × min/m3 (43). Cyanide does not produce a constant effect of lethality; exposure to a high dose of cyanide gas even for a very short period of time will be fatal in comparison to smaller doses over a longer time. Inhalation of a concentration of cyanide at 300 mg/m3 (270 ppm) will result in immediate death. In contrast, it would take several hours of exposure to a concentration of 20 mg/m3 (18 ppm) before even mild symptoms would be seen (44). The form of cyanide significantly influences the toxic dose. The LD50 for IV CK is 1 mg/kg, and the estimated LD50 for dermal CK is 100 mg/kg. The lethal dose of an ingested cyanide salt is between 50 and 200 mg, depending on the specific salt form (43).
The severity and time to onset of clinical symptoms depend on the amount and mode of exposure as well as the type of cyanide. Intravenous and inhalational exposures to cyanide produce the most rapid onset of symptoms. Death can occur within seconds to minutes. Ingestion of cyanide salts may result in a delayed presentation of toxicity as the cyanide must first be absorbed. Presentation may be further delayed after dermal exposures to intact skin. Exposure to the gaseous form of cyanide results in immediate toxicity. Signs of poisoning from exposure to the salt forms of cyanide will be delayed to varying degrees depending on the specific salt. Exposure from the cyanide salts elicits a less dramatic progression of signs and symptoms. The soluble salts display a reduced onset to action time as compared to insoluble salts. One important exception to this would be the case of mercurial salts of cyanide. The mercury content in these chemicals is highly caustic and immediately elicits noxious injury to tissue. Significant cyanide poisoning may follow, but the clinical presentation is confounded by the concurrent mercury poisoning. The delay in clinical presentation of toxicity from cyanogens and cyanide compounds such as the nitriles will be even more pronounced. Cyanide can be transferred into breast milk and therefore can pose a significant risk to children through secondary transmission (45).
MECHANISM OF TOXICITY
Sulfane and cyanocobalamin reactions primarily within the liver metabolize the low levels of cyanide encountered normally. In the acute poisoning situation, endogenous pathways of cyanide metabolism are rapidly overwhelmed, and conjugate substrates are depleted allowing for accumulation and progression of toxic effects. Although cyanide inhibits many additional metabolic processes, the commonly cited mechanism of toxicity involves binding of cytochrome oxide within the mitochondria (46) (Fig. 3-2). The cyanide avidly binds to the ferric ion (Fe3+) on the a3 complex. Subsequently, oxygen no longer is able to re-oxidize the reduced cytochrome a3, effectively bringing electron transport to a halt. This uncoupling of oxidative phosphorylation in essence terminates the synthesis pathway of adenosine triphosphate (ATP). The mitochondria exhibit impaired oxygen extraction and utilization despite adequate exposure to the oxygen supply (47). In response to this disruption of the primary metabolic aerobic pathway, the rate of glycolysis via anaerobic pathways increases.
The binding to the mitochondrial oxidase system can be delayed by a few minutes, but early signs of cyanide poisoning are seen within seconds. This observation leads to the theory of additional mechanisms of toxicity. Because cyanide exists predominantly in the un-ionized form within the body, it readily diffuses across membranes. Rapid effects seen after inhalation may be due to the near instantaneous diffusion across the blood brain barrier. Cyanide appears to alter neuronal transmission following absorption into the central nervous system (CNS) possibly through a glutamate pathway. It also appears to increase vascular resistance early in poisoning and to increase cerebral blood flow further potentiating penetration. These additional mechanisms represent just a few of the potential toxicities and open the door to experimentation with new therapies (8).
SIGNS AND SYMPTOMS
ACUTE CLINICAL MANIFESTATIONS
Exposure to high concentrations of cyanide can result in death within seconds to minutes leaving inadequate opportunity to recognize toxic symptoms and initiate management (34,48). Cyanide is often classified as a blood agent although it manifests primarily as CNS and cardiac toxicity. In cases of more prolonged onset of toxicity, signs and symptoms reflect a progressive intracellular hypoxia. Clinical hypoxia with cyanosis in the case of a worsening acidosis is a hallmark of cyanide poisoning. Initial symptoms are nonspecific and transient. The early symptoms of dizziness, headache, weakness, diaphoresis, dyspnea, and hypernea may be misinterpreted as anxiety. Without a reasonable index of suspicion, the diagnosis may be missed (49). The effects on respiration are thought to be mediated via direct stimulation of the carotid body and peripheral chemoreceptor bodies (8). This immediate stimulation of chemoreceptor
bodies also mediates a transient rise in blood pressure. HCN and cyanogen chloride gas may cause mucous membrane irritation as well. Cyanide is purported to have an odor of bitter almonds, but only around 40% to 60% of the population have the gene necessary to detect this odor. The presence of a bitter almond odor is not a reliable sign of cyanide exposure (50,51). Although cyanide historically is associated with a cherry red flush, the dramatic color alteration is not always present. Visualization of equally red retinal arteries and veins can be used as an easy and rapid indicator of cyanide toxicity. The venous blood supply will have a similar oxygen concentration as the cells are no longer able to extract oxygen efficiently (52). As cellular hypoxia worsens, victims will experience loss of consciousness progressing to coma with fixed dilated pupils, hemodynamic compromise, arrhythmias, seizures, apnea, secondary cardiac arrest, and finally death. Those organs such as the heart and brain with high oxygen demands are the most sensitive to cyanide poisoning.
bodies also mediates a transient rise in blood pressure. HCN and cyanogen chloride gas may cause mucous membrane irritation as well. Cyanide is purported to have an odor of bitter almonds, but only around 40% to 60% of the population have the gene necessary to detect this odor. The presence of a bitter almond odor is not a reliable sign of cyanide exposure (50,51). Although cyanide historically is associated with a cherry red flush, the dramatic color alteration is not always present. Visualization of equally red retinal arteries and veins can be used as an easy and rapid indicator of cyanide toxicity. The venous blood supply will have a similar oxygen concentration as the cells are no longer able to extract oxygen efficiently (52). As cellular hypoxia worsens, victims will experience loss of consciousness progressing to coma with fixed dilated pupils, hemodynamic compromise, arrhythmias, seizures, apnea, secondary cardiac arrest, and finally death. Those organs such as the heart and brain with high oxygen demands are the most sensitive to cyanide poisoning.
![]() Figure 3-2. The binding of the cytochrome oxidase within the mitochondria resulting in abrupt cessation of cellular respiration (1). Cyanide binds to the iron in the cytochrome a3 complex (2). Oxidative phosphorylation is blocked and oxygen no longer is utilized (3). Electron transport chain is uncoupled resulting in loss of aerobic metabolism/generation of ATP (4). Nitrites form methemoglobin, which draws cyanide out of mitochrondria (5). Thiosulfate combines with cyanomethemoglobin and cyanide and is converted to the less toxic thiocyanate, which is excreted in the kidney. |
LABORATORY ABNORMALITIES
The most prominent laboratory finding in cyanide toxicity is a metabolic acidosis with dramatically elevated lactate levels. The shift from aerobic to anaerobic metabolism ultimately results in marked production of lactate as a profound high anion gap acidosis ensues. Several reports indicate that lactate levels may be utilized as markers of severity of cyanide toxicity (24,53). This correlation may be confounded by numerous processes in a critically ill patient, therefore its utility is limited. Later in the course, the metabolic acidosis may be compounded by the addition of a respiratory acidosis from worsening apnea.
In the case of an unknown exposure, check venous blood gases as well as arterial blood gases to determine if there is a supranormal venous oxygen content or “arteriolization of venous blood,” which would strongly indicate cyanide toxicity (54,55,56). The pulse oximetry will remain high as the blood has good oxygen content; consequently, the problem will lie in oxygen utilization and extraction. Note that once therapy has been initiated to induce methhemoglobinemia, the pulse oximetry will in fact depict a higher than actual oxygen concentration as both entities deflect light at similar wavelengths (57,58).
Although cyanide does target cardiac tissue, no prominent electrocardiogram (EKG) changes are noted. The patient may demonstrate a variety of conduction abnormalities including junctional rhythms, heart block, atrial fibrilliation, and premature beats. Case reports have described nonspecific ST-T segment elevation and depression, but this is not characteristic of cyanide poisoning. As the clinical picture worsens, the poisoned patient may eventually develop shock with profound hypotension, bradyarrhythmias, and possibly noncardiogenic pulmonary edema (49).
Cyanide tests are not readily available and often require a few days as turnaround time. Cyanide levels should be used only to verify toxicity; management of the victim should be based upon index of suspicion and clinical presentation. Levels may not necessarily correlate with clinical manifestations due to frequent, but unavoidable interferences. Red blood cells have the highest concentration of cyanide, and whole blood specimens should be collected for analysis.
Cyanide levels tend to decrease over time, and even the most carefully handled specimens may underestimate true peak concentrations (59). Note that moderately elevated cyanide levels probably indicate a severe toxicity due to the extreme extent the cyanide molecules adhere to the cytochrome oxidase (47). Reported toxic concentrations of cyanide range from levels as small as 0.5 μg/ml. A conversion factor of 0.026 should be used when interpreting traditional units and International System units (μg/mL = μmol/L × 0.026). Cyanide levels may be misleading and patient care should be based on clinical presentation.
Cyanide levels tend to decrease over time, and even the most carefully handled specimens may underestimate true peak concentrations (59). Note that moderately elevated cyanide levels probably indicate a severe toxicity due to the extreme extent the cyanide molecules adhere to the cytochrome oxidase (47). Reported toxic concentrations of cyanide range from levels as small as 0.5 μg/ml. A conversion factor of 0.026 should be used when interpreting traditional units and International System units (μg/mL = μmol/L × 0.026). Cyanide levels may be misleading and patient care should be based on clinical presentation.
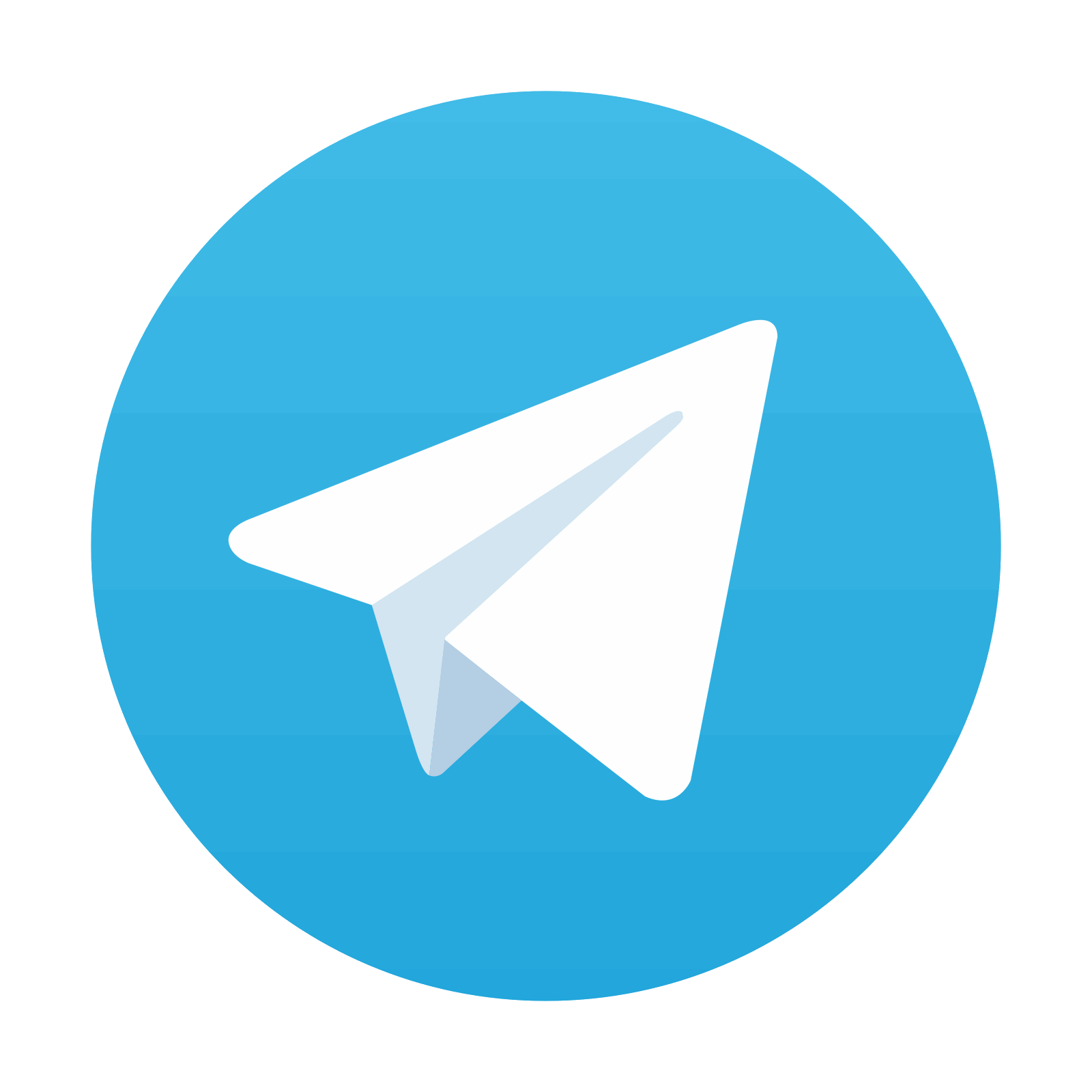
Stay updated, free articles. Join our Telegram channel

Full access? Get Clinical Tree
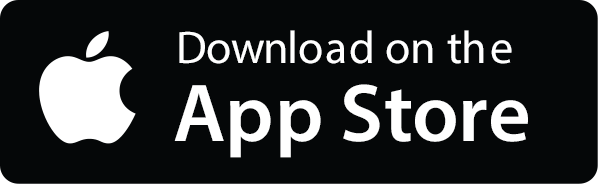
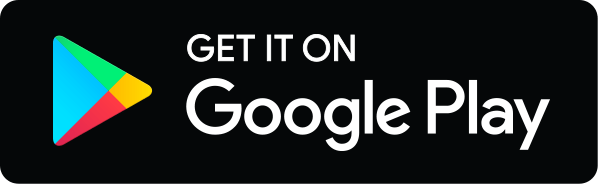