of all implanted prostheses will be replaced after 10 years as a consequence of the aseptic loosening of one implant component [3]. Especially the migration or loosening of the artificial hip cup due to bone remodelling is still a current problem [4, 5]. With regard to the ageing population this means an increase in revision surgeries marked with a higher risk of complications and thus increasing cost for the health care system.
The migration or loosening may be a result of the changed mechanical conditions after the implantation of the THA. This change can lead to a bone remodelling caused by stress shielding [6]. Furthermore, for a stable anchoring of the hip cup a high bone resection is necessary which promotes bone resorption and hampers revision surgery. A patient-specific solution can be used to counteract these disadvantages of conventional hip cups. However, individual hip cups are only implanted for the treatment of great deformations or tumours. This is due to the time- consuming and cost-intensive production of each components [7].
The overall aim of this project is the development of an innovative and economic concept for the design and production of patient-individual hip cups for primary surgery by means of sheet metal forming. Specific limitations (e.g. rigid tools) of conventional forming processes normally prevent a time-saving and economically reasonable patient-individual production. Nevertheless, the use of metal forming for the manufacturing of customized products is necessary because of excellent mechanical component properties, extensive shaping formabilities as well as optimal material utilization. Currently potential approaches to an individual production are unitized tools, CNC-controlled incremental forming methods or multi-point forming [8–10].
The manufacturing concept developed in this project consists of an innovative two-stage sheet metal forming process. The development is accompanied by a FE simulation-based planning as well as a and a new design method adjusted to the process. The following sections of this paper give the results of a numerical investigation of the bone remodelling process with a conventional prosthesis via finite element method (FEM) coupled with multi-body simulation (MBS) as well as the idea of the concept for the manufacturing of the individual hip-cup. Based on already executed comparative simulations, the high pressure sheet metal forming (HPF) is introduced for the manufacturing of the standardized components. Afterwards the first part of the design method is demonstrated, which contains the deduction of a universal acetabular geometry, necessary for the production of the standardized component.
2 Bone Remodelling
As already stated, the migration of hip-cup prostheses due to bone remodelling is still a problem. A numerical method for the prediction of the bone remodelling after implantation of a artificial hip-cup is utilized below to emphasize the need of customized prostheses.
By using a THA the physiological load distribution in the femur and the acetabulum is modified. This modification is due to the fact that the bone stiffness is considerably lower than the stiffness of the implant material. Thus, a large proportion of the load is not directed over the bone but preferably over the prosthesis. In accordance with Wollfs law, which says that changes in form and function of a bone lead to changes in internal structure and external form [11], the so-called strain-adaptive bone remodelling occurs, which can lead to a migration or loosening of the prosthesis [12, 13].
Due to the mentioned problems a qualitative and quantitative prediction of the bone remodelling is important for implant design. At the Institute of Forming Technology and Machines a simulation method was developed to calculate the strain-adaptive bone remodelling in the periprosthetic femur via FEM [14, 15]. Furthermore, the bone remodelling in the pelvis after total hip replacement was numerically simulated on the basis of the established simulation method [16, 17]. In Fig. 1 the FE-model of this simulation is illustrated, consisting of the bony structure provided with a cemented cup. In addition to the FE-model the components of the calculated resultant hip joint forces used as boundary conditions are pictured in Fig. 1. For the determination of hip joint forces an MBS model of a human test person with a normal walking speed (
m/s) was used.
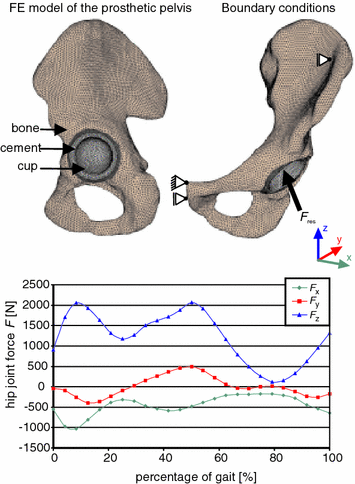

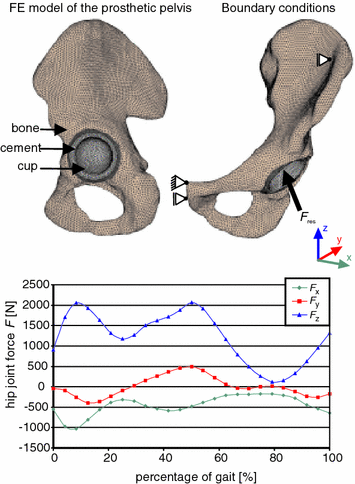
Fig. 1
FE-model of the periprosthetic pelvis and components of the calculated resultant hip joint forces [17]
According to the simulation with load conditions derived from the MBS a total bone loss of
can be assumed in the periprosthetic pelvis. The density distributions in the periprosthetic pelvis for the initial and final state are illustrated in Fig. 2. A high bone resorption can be observed in the acetabulum. On the basis of the final density distribution in the acetabulum a migration of the conventional cup can be suggested in proximal direction.

This migration can be avoided by means of patient-individual hip cups. In the following section a new concept for the design and production of patient-individual hip-cups prostheses out of titanium sheets will be introduced and the results of the concept development will be presented.
3 Manufacturing Concept
It is planned to produce the patient-individual hip-cup prosthesis by a two-stage manufacturing process consisting of the following steps: The first step is the production of standardized titanium sheet metal components with undersize. These standardized components can be produced by means of punch-die forming (“conventional” deep drawing with punch, blank holder and die) or HPF in large-scale production. In the second step the true-to-size enlargement of the produced standardized components is executed. This enlargement is realized by double acting rubber-die forming with adjustable elastomer lower die considering the present patient-individual geometries of the acetabula.
In the following the production of the standardized titanium sheet metal components is considered in detail. Thereby comparative simulations are presented for the identification of the appropriate method for the first production step. Taking into account these simulations, the HPF-process will be introduced to the production of the standardized components.
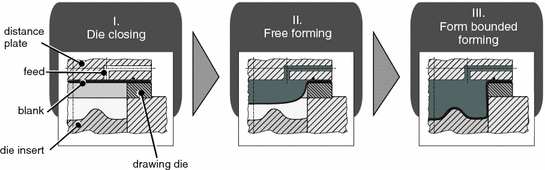
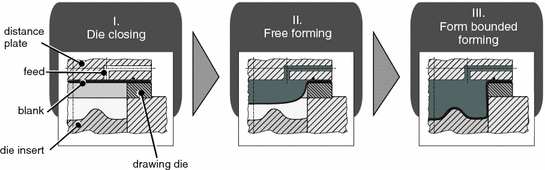
Fig. 3
Process sequence of high-pressure sheet metal forming with die insert
3.1 Comparative Simulations
Comparative simulations were executed to identify the most convenient method to produce the standardized prosthesis [18]. This preliminary investigation consists of the simulation of both forming concepts at room temperature and at 200
C using commercially pure titanium grade 2 characterized by means of standard tentile testing experiments at both temperatures. Except for the HPF at room temperature, the manufacturing of the standardized hip-cup prosthesis by means of the other forming methods and conditions lead to a critical reduction of the sheet thickness (
). Thus the HPF-process at room temperature was choosen for the real process described in detail in the next section.

3.2 High Pressure Sheet Metal Forming
The HPF process offers, in addition, the following advantages over the punch-die forming procedure [19]:
In Fig. 3 the process sequence is illustrated. First the die is closed, so that blank and distance plate are in contact. This leads to a sealed interior space. Then the fluid is pumped into the interior space of the die through the feed in the distance plate. During the first forming phase a free forming take place. In the second phase, the form-bounded forming takes place, so that the blank is pressed against the contour of the die insert.
use of different blank thicknesses and qualities without changing the die,
production with less stages,
improvement of buckling strength as well as dimensional and geometrical accuracy.
4 Derivation of the Universal Prosthesis Geometry
To realize the manufacturing concept the development of the two-stage manufacturing process is accompanied by a design method adjusted to the two-stage manufacturing process presented before. For the manufacturing of the standardized components, a universal acetabulum geometry should be derived from the CT-data of real hip joints. By means of reverse engineering an associative parametric model is generated based on this universal geometry. The parametric composition of the produced universal geometry finally allows the adjustment of the parametric model to the individual geometry of the patient. For this geometrical superposition and a parameter adaptation is planned. The resulting individualized geometry is used for the enlargement step with the double acting rubber-die forming.
In this section the approach to the generation of the universal prosthesis geometry will be introduced. Fist the general design chain of the generation of the universal geometry is presented as well as a preliminary investigation. Subsequently the agglomerative clustering is introduced and utilized for the reproducible deduction of the universal geometry.
4.1 General Design Chain
The method for the derivation of the universal prosthesis geometry is firstly implemented by means of canine pelvis geometries. In Fig. 4 the design chain for the deduction of the universal geometry is illustrated.
