Fig. 13.1
Application of microarray technologies for PGS. Following biopsy, DNA is amplified using WGA, and then each biopsy sample is labeled with green fluorescent tags. Green biopsy sample is mixed with normal male DNA labeled with red fluorescent tags in equal proportions. The mixture is hybridized onto the BAC array and, following stringent washing, the slide is analyzed for the proportion of red and green fluorescence on each spot. Computer algorithms are used to assess each spot and call any gains or losses of chromosome in the test sample as compared to the normal DNA
In the case of array CGH, the DNA spotted onto each slide can be from bacterial artificial chromosome (BAC), DNA clones (typically longer sequences) from defined chromosomal regions, or specific oligonucleotides (shorter DNA sequences). This chapter will focus mainly on the BAC clone approach as this system is the most well validated of the methods and has been used for well over 400,000 clinical preimplantation genetic samples to date. The most widely used array, 24SureTM (Illumina Inc., San Diego, CA) contains nearly 3,000 DNA spots spaced approximately 1 Mb apart. Each clone was chosen based on minimizing copy number polymorphisms, and its location has been confirmed via reverse painting and FISH mapping.
Embryo Biopsy and Sample Handling
Following biopsy of the egg (polar body 1 and/or 2), embryo (cleavage stage), or blastocyst, the sample is washed through a number of droplets, most often phosphate buffered saline (PBS) with an additive such as polyvinyl alcohol (PVA) to reduce cell stickiness. The sample is then picked up in a small volume (<2 μL) and placed into a sterile 0.2 mL Eppendorf tube for transport to the laboratory for testing. Most embryologists perform a quick step to ensure that the cellular material and all of the fluid are collected together at the bottom of the sample tube. This can be accomplished by centrifugation or a quick flick of the tube to collect the sample in the bottom. Depending on the length of time the sample will have to travel, ambient temperature or wet ice (blue ice packs) can be used for shorter trips, while dry ice may be used for longer distance trips, especially in locations with warm weather at certain times of the year. While mineral oil may be used as an overlay in molecular biology experiments that require polymerase chain reaction (PCR), it should be noted that mineral oil should never be used prior to whole genome amplification (WGA) and aCGH as it inhibits the amplification process and will yield samples with no results. Of course, each laboratory will have its own standard operating procedure for sample handling ahead of transport to the testing laboratory.
Whole Genome Amplification and Labeling
A number of different WGA methods have been used historically for array CGH experiments, with the current, most often utilized method being SurePlexTM (Rubicon Genomics Inc., Ann Arbor, MI, USA and BlueGnome). This WGA kit is fragment amplification based, where self-inert degenerative primers are annealed at multiple sites along the genome. This system was chosen because it produces optimal fragment sizes, which have been found to be reproducible between samples and are optimized for array CGH. Many of the other WGA techniques have been adapted for use in array CGH but were originally used for other purposes (e.g., single locus PCR and mutation detection). SurePlexTM is suitable because of its simple, short protocol and highly representative amplification.
Following sample receipt and accessioning in the lab, each tube is opened in a dedicated DNA amplification clean room, under a laminar flow or PCR-dedicated hood. Amplification is performed according to the manufacturer’s instructions as the SurePlexTM kits have been validated for use in single cells. When using the SurePlex kit, the first step is lysis/extraction step (15 min), followed by pre-amplification steps (90 min), and finally amplification (30 min). To reduce possible contamination issues and eliminate the risk of accidental sample switches, all steps in SurePlex are performed in a single tube. The procedure is performed in a PCR machine as each step is temperature and time dependent.
After SurePlex, agarose gel electrophoresis is performed to confirm successful amplification. As the arrays can be quite expensive, it is best to ensure amplification prior to taking the sample further through the process. A smear of DNA near the top of the gel is indicative of good amplification; low molecular weight DNA or no DNA would be indicative of poor/no amplification. Following agarose gel verification of good amplification, the WGA product is labeled through nick translation with either Cy3 (green) or Cy5 (red) fluorescent tags.
Hybridization
In traditional aCGH, embryo biopsy samples labeled in one fluorescent color (e.g., green) and control reference DNA (typically a karyotypically normal male) labeled in an alternative color (e.g., red) are denatured at 74 °C to make the DNA single stranded. The single-stranded DNAs from the sample and control are then mixed together in equal proportions in hybridization buffer containing formamide and cot-1 human DNA before being adding to each 24SureTM microarray. Microarrays are hybridized at 47 °C for at least 4 h or overnight in a humidified chamber. The length of hybridization time varies depending on the timing of biopsy, the number of samples in the lab on any given day, staffing levels, and shift patterns. During validation of the array in the lab, hybridization times as short as 3 h and as long as 16 h (overnight) were tested with no differences in diagnostic accuracy noted [28]. On the basis of these results, hybridization for at least 4 h and no longer than 16 h is deemed to be interchangeable. It should be noted, however, that shortening both labeling and hybridization may lead to suboptimal results; therefore, any protocol used clinically should be robustly validated prior to use on actual human samples.
More recent advances have led to so-called single channel aCGH. With this method, control DNA is not hybridized on each array, rather the control DNA is hybridized in each fluorescent color (Cy 3 and Cy 5), for both normal male and normal female, on separate arrays run during each experiment. Therefore, each experimental array in single channel aCGH contains two embryo biopsy samples, one labeled in Cy3 and another labeled in Cy5. When the analysis is performed, each experimental sample is compared in silico to the male and female reference separately. Single channel aCGH allows for more samples to be run per experiment and reduces the amount of control DNA necessary in each experiment. This has also allowed the price per sample to be lower than in conventional aCGH.
Post-Hybridization Washing
Following hybridization, each microarray is washed as follows: 10 min in 2×SSC/0.05 % Tween 20 at room temperature, 10 min in 1×SSC at room temperature, 5 min in 0.1×SSC at 60 °C, and 2 min in 0.1×SSC at room temperature to remove unbound DNA.
Scanning
Each microarray slide is scanned using a dual channel fluorescent laser scanner in order to create TIFF images (e.g., ClearScanTM, Illumina) showing green fluorescence at 632 nm and red fluorescence at 587 nm associated with hybridization of embryo and reference DNA samples, respectively. Raw images are loaded automatically into analytical software such as BlueFuseTM for evaluation of fluorescent signals (ratio analysis).
Scoring
Sample scoring is typically performed by a trained technologist who assesses traces for all 24 chromosomes, noting all gains and losses, as well as determining the sex of each sample. A second technologist then scores the sample blindly, with no knowledge of the initial scoring. The final result for each sample is then assigned by comparing the two scores. If discrepancies are noted between the two assessors, they are typically adjudicated by a third technologist and/or the laboratory supervisor or director. It should be noted that the current version of the BlueFuseTM software allows for automated calling of whole chromosome gains and losses; however, most laboratories do not rely on this for clinical diagnosis.
Reporting
Once results for all samples from each patient are finalized, a diagnostic report is prepared, signed off by an appropriately qualified person (on site or remotely), and shared with the referring laboratory and physician prior to embryo transfer.
Discussion
Validation
In extensive validation using single cells from known cell lines against the gold standard of karyotyping, 24SureTM demonstrated 98 % accuracy [29]. The use of cell lines does, however, have drawbacks. During this validation, mosaicism was seen in most cell lines meaning that any one cell in the culture may or may not always have the same molecular genotype. Validation for embryo aneuploidy is perhaps even more difficult as truth data (i.e., definitive proof that the sample used as an unknown is actually the genotype that you expect it to be); this is difficult to obtain for human embryos grown in culture (due to mosaicism, for example). Human oocytes offer an interesting method for validation. One can biopsy the first and second polar body and use array CGH to analyze the chromosome complement in each sample individually. This method allows a laboratory to look at trios of data, comparing the first and second polar body to the oocyte. The expectation is to see reciprocal chromosome gains and losses from aneuploid polar body(ies) and oocyte [30].
Limitations of Array CGH
While array CGH has been shown to be highly accurate and reproducible in multiple validation studies and has been used on hundreds of thousands of embryo samples, it still has drawbacks that must be understood prior to clinical use. For example, aCGH cannot discriminate between maternal and paternal errors; it can simply elucidate chromosome gain and loss. It remains to be determined whether knowledge of the parental source of error has clinical value. Array CGH cannot distinguish between meiotic and mitotic errors of chromosome segregation; however, again the data on whether this is an important factor remains unclear. Perhaps the most clinically relevant limitation of aCGH is the fact that it cannot distinguish a euploid embryo from certain forms of triploidy (i.e., 69,XXX chromosomes) or tetraploidy (i.e., 92 chromosomes). Purely triploid and tetraploid embryos often implant, leading to pregnancy loss prior to delivery.
Competing Technologies
While array CGH has become the gold standard and most widely used method for counting chromosomes clinically, there are a number of competing platforms that could challenge this position. As with all competing technologies, there are advantages and disadvantages to each [31, 32]. Comprehensive chromosomal screening using multiplex quantitative PCR (qPCR) [33] has been proposed as a faster and less expensive means of detecting aneuploidy. However, the qPCR systems optimized for embryo analysis have not been fully commercialized, restricting availability. Furthermore, existing qPCR systems are only applicable to trophectoderm samples and cannot be used for the analysis of polar bodies or single blastomeres. Chromosome counting can also be performed through the use of single nucleotide polymorphism (SNP) arrays. Using a combination of loss of heterozygosity, quantitative SNP calling, and analysis of patterns of SNP inheritance from parents to embryos, it is possible to detect chromosomal gains and losses [34–37]. SNP-based arrays do offer the ability to detect the parent of origin in aneuploidy cases and have been validated to reliably detect inheritance of specific genotypes allowing for nearly universal detection of many single gene defects [34]. However, SNP arrays also have a much longer protocol (24 h+ in most cases), are typically more expensive than alternative methods, and typically require parental DNA ahead of testing adding to the cost and time needed for this type of array.
Noninvasive Indirect Methods of Determining Aneuploidy
It is appealing to consider noninvasive approaches to embryo selection. Weak correlations exist between the presence of embryonic aneuploidy and morphological aspects of embryo development following retrospective analysis [38, 39]. These findings have stimulated the field of morphokinetic analysis during IVF, with an attempt to identify aneuploidy in a real-time clinical setting. Analysis of time-lapse imaging during embryo growth demonstrates that certain morphologic features and/or developmental timings of the embryo may have some relationship to aneuploidy [40, 41]. This data, if confirmed in larger data sets with appropriate subgroup analysis stratified by maternal age and in multiple clinics, may provide some useful information to place embryos in the order of priority for transfer. However, it does not appear that morphokinetics will have the capacity to provide the same level of specificity and accuracy yielded by aneuploidy testing using array CGH. Another promising morphokinetic approach is to assess dynamic fragmentation patterns within early embryos but again, this currently does not identify specific aneuploidies and only provides a relative risk of abnormality for each embryo [42]. Currently, no morphokinetic parameter or set of parameters has been shown to be able to discriminate between euploid and simple aneuploid (e.g., trisomy 21) embryos. In addition to morphokinetics, measurement of specific metabolites or combinations of biologically relevant molecules in culture medium has been suggested as a method to predict the viability of an embryo. However, none of these methods have been proven to be able to differentiate between general chromosomal aneuploidy and specific aneuploidy in prospective controlled studies.
Next-Generation Sequencing for Chromosome Counting
Next-generation sequencing (NGS) may supercede all other methodologies as it promises several advantages over all other techniques [43]. The term next-generation sequencing (NGS) describes several distinct methods that share in common an ability to provide huge quantities of DNA sequence data from the samples analyzed, rapidly, and at relatively low cost. There are two ways in which NGS can be employed for the detection of aneuploidy screening. The first involves biopsy of cells from embryos followed by whole genome amplification, after which the DNA is broken into small fragments and then subjected to NGS. The sequence of each fragment is compared to the sequence of the human genome, allowing its chromosome of origin to be determined. The relative proportion of fragments attributable to each chromosome is indicative of its copy number—e.g., an increase in the proportion of DNA fragments derived from an individual chromosome (relative to a chromosomally normal sample) is evidence of a trisomy. The second way that NGS can be used for aneuploidy detection involves the use of multiplex PCR (rather than WGA) to simultaneously amplify multiple specific loci on each chromosome. After amplification, the mixture of DNA fragments is analyzed using NGS, and the number of sequences attributable to each chromosome is calculated. A deviation from the expected number of DNA fragments for a particular chromosome is indicative of aneuploidy.
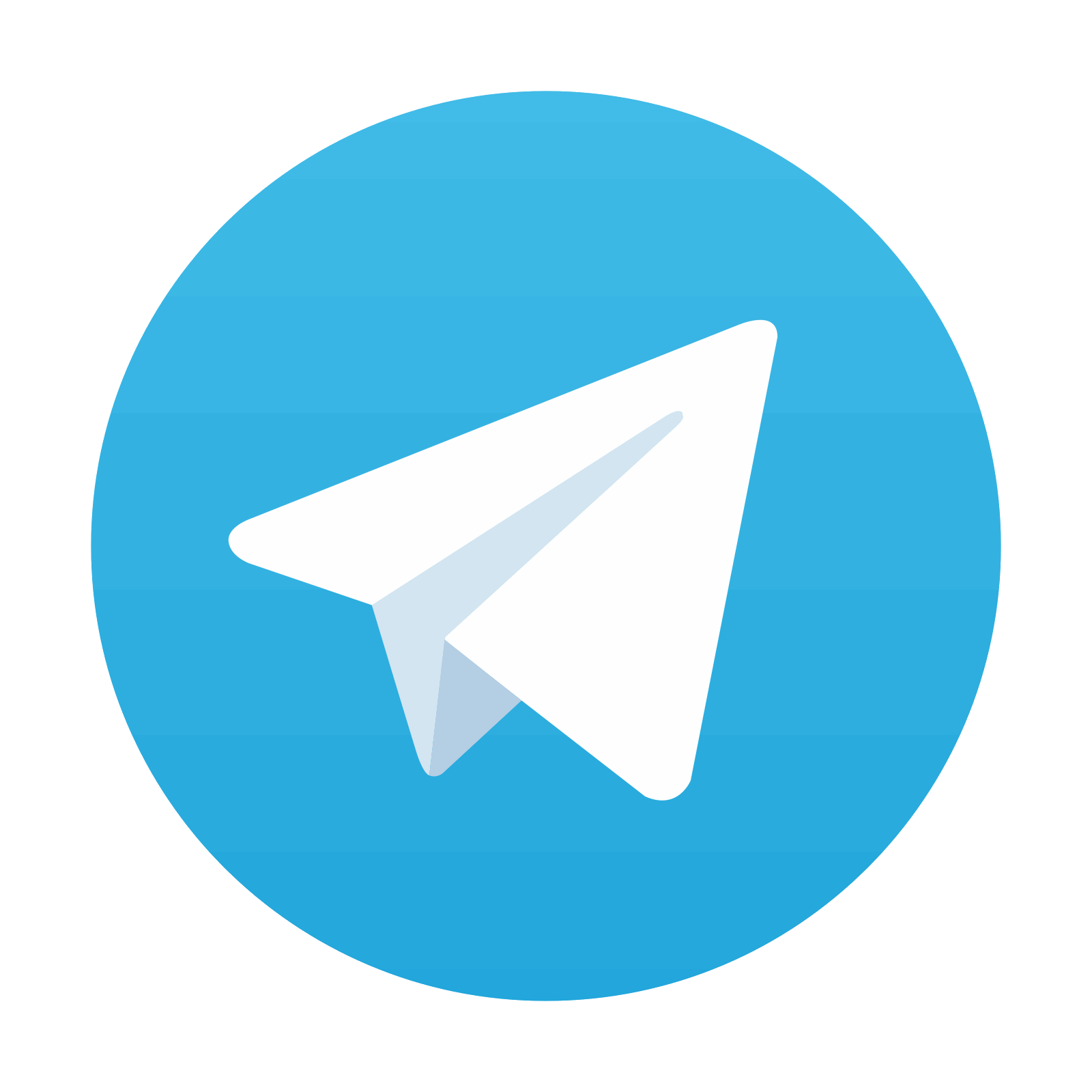
Stay updated, free articles. Join our Telegram channel

Full access? Get Clinical Tree
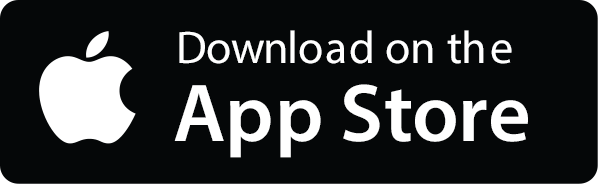
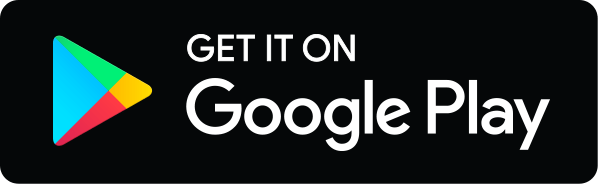