2.11.15 C(sp3)—H Functionalization by Allylic C—H Activation of Zirconocene Complexes
A. Vasseur and J. Bruffaerts
General Introduction
Among the different transition-metal-catalyzed C—H activation options involving an inner-sphere or outer-sphere mechanism, the former is particularly attractive to synthetic chemists.[1] Such a process affords, after C—H cleavage, a transition-metal species which can be functionalized by reaction with either an external reagent or a reactive group already present at the metal center. These transformations generally proceed with good stereoselectivity, notably with the C—H bonds of less sterically hindered molecules. Moreover, they can be controlled by tuning the properties of the organometallic species (for instance, the choice of metal or the use of ligands). Through this activation mode, a very large number of reactions functionalizing the C—H bonds of alkynes,[2–8] aldehydes,[9–21] alkenes, and arenes[22–37] have been reported in good yields and good stereoselectivities.
In contrast, examples of C(sp3)—H functionalization are less numerous. The presence of a π-system allows the substrate to complex to the metal, which contributes to C(sp2)—H or C(sp)—H activation. The absence of this type of system from saturated derivatives makes activation of nonacidic C(sp3)—H bonds extremely challenging. Different approaches have been developed to this end, using precoordination of the metallic complex to the substrate by a variety of means:[38,39] oxidative addition of the metal into a C—halogen bond,[40–43] or coordination of the metal to the nitrogen atom of a C—N bond.[44,45] In addition to heteroatoms, unsaturation can also be used to direct C—H activation. In particular, allylic C—H activation enables the formation of π-allylic complexes on which a variety of carbon, oxygen, or nitrogen reagents can react. These reactions are typically conducted with palladium,[46–51] rhodium,[52–54] ruthenium,[55,56] or zirconocene complexes.[57]Zirconocene-assisted allylic C—H activation has the advantage of proceeding rapidly, under mild conditions, and being stereoselective, regioselective, and insensitive to the length of the carbon chain.[58] Moreover, the number of different functionalities that can be introduced by cleavage of the σ-C—Zr bond makes it a valuable tool for organic synthesis.[59–62] Furthermore, possible subsequent elimination,[63–65] ring expansion,[66] selective carbon–carbon bond activation,[67–69] or transmetalation reactions[70] can dramatically increase the synthetic potential. At the same time, hydrozirconation–isomerization of internal alkenes makes unactivated C(sp3)—H bonds accessible to substitution.[71] In any case, these transformations imply a migration of the double bond but the mechanism is very different since the former brings into play a low-valent zirconocene complex and the latter involves a high-valent zirconocene complex. Consequently, the resulting products will be different too, depending on whether a zirconium(IV) or (II) species is used, as depicted in the simplified ▶ Scheme 1.
High-valent zirconocene complexes, such as the Schwartz reagent (1),[72–75] initially prepared by Kautzner, Wailes, and Weigold,[76] undergo a 1,2-insertion of the alkene into the Zr—H bond followed by a β-hydrogen elimination. This reaction mechanism thus leads to an isomerization of the alkene double bond and regeneration of the zirconium hydride.[77–81] A second 1,2-insertion of the resulting isomerized alkene into the Zr—H bond then affords a terminal alkyl(chloro)bis(η5-cyclopentadienyl)zirconium(IV) complex (e.g., 2), which provides the corresponding functionalized alkane 3 by reacting with an electrophile. This is an efficient way to selectively functionalize C(sp3)—H bonds of unsubstituted internal alkenes through a multipositional isomerization of the double bond,[82–84] even with very long carbon chains.[85,86] Nevertheless, when heteroatom-substituted internal alkenes[87–92] or aromatic internal alkenes[93–97] are involved in such a reaction pathway, many important issues arise in terms of regioselectivity, drastically limiting the synthetic interest.
In contrast, low-valent complexes such as the Negishi-type reagent 4 can lead to regioselective reactions. The reaction proceeds through the formation of a zirconacyclopropane intermediate 5 from the involved alkene as a result of a ligand exchange. After a direct allylic C—H activation, the formed (allyl)bis(η5-cyclopentadienyl)hydridozirconium species 6 yields a terminal alkene 7 functionalized in the allylic position by reacting with an electrophile. This chapter especially focuses on reactions enabling functionalization of unactivated C(sp3)—H bonds by allylic C—H activations.
Scheme 1 Simplified Mechanism of C(sp3)—H Functionalization of Internal Alkenes Using High- and Low-Valent Zirconocenes[114]

The low-valent zirconocene derivative (η2-but-1-ene)bis(η5-cyclopentadienyl)zirconium (4), also called the Negishi reagent, is usually prepared by treatment of dichlorobis(η5-cyclopentadienyl)zirconium(IV) with 2 equivalents of butyllithium in tetrahydrofuran or diethyl ether at −78 °C.[98] Initially, this reaction furnishes a dibutylbis(η5-cyclopentadienyl)zirconium species 8, which is unstable at room temperature and therefore readily suffers from a thermal decomposition leading to the elimination of butane following abstraction of hydrogen at the β-position relative to the metal center (▶ Scheme 2). Proceeding through a nondissociative pathway,[99] which distinguishes it from a β-hydrogen elimination, this β-hydrogen abstraction formally provides a zirconacyclopropane 9 that could also be considered as the 16-electron species 4.[100,101]
Induced by an agostic interaction between the vacant d-orbital of the metal and the C—H bond at the β-position, β-hydrogen abstraction is mainly governed by steric effects.[102]Such a chemical event is indeed easier for a β-methyl group than a β-methylene group, and easier for a β-methylene group than a β-methine group. However, electronic factors can also play a significant role. For instance, the phenylethyl group is a better β-hydrogen donor than ethyl (a β-methyl group), presumably due to benzylic stabilization in the styrene–zirconocene complex. Electronic effects are also responsible for the inhibition of β-hydrogen abstraction notably when the empty orbital of the metal is unavailable as a result of stabilization of the dialkylzirconocene species by chelation [for example, during the formation of bis(η5-cyclopentadienyl)bis(3-ethoxypropyl)zirconium or lithium tributylzirconate].[102]
A detailed NMR study has revealed that the complex, versatile behavior of dibutylbis(η5-cyclopentadienyl)zirconium(IV) (10) during its thermal decomposition is because it can follow different paths where the oxidation state of the metal is maintained or changed (▶ Scheme 3).[103] Indeed, during the thermal decomposition, a plethora of species, in equilibrium with each other, are generated, and if only one compound is consumed in a given reaction (even if its concentration is not high), the whole system can evolve toward it. Very briefly, dibutylbis(η5-cyclopentadienyl)zirconium(IV) (10) would first lead to a zirconacyclobutane 11 by a γ-hydrogen abstraction from the neighboring butyl group via transition state 16. Further transformation would lead to crotylzirconocene hydride 12 (probably existing as a +3 oxidation state species)[104] proceeding by β-hydrogen abstraction from the methylene group via transition state 17. Crotylzirconocene hydride 12 would then undergo rearrangement to produce zirconacyclopropane 9, via insertion of the double bond into the Zr—H bond,[105–107] and the dimeric butenylzirconocene hydride 13 by a 1,3-hydrogen shift process via transition state 18.[105] Further thermolysis would finally lead to the formation of zirconacyclopentane 14 from zirconacyclopropane 9 through a reversible, unclear mechanism,[108,109] and the symmetrical dimer 15 (presumably from zirconacyclobutane 11 and/or zirconacyclopentane 14), a symmetrical dimer where two zirconocenes would be bonded to the (α,γ)- and β-positions of the carbon chain.
In view of the possible β-carbon-hydrogen agostic interactions mentioned above, the allylic C—H activation by zirconium in alkene–zirconocene species is not surprising and such a process easily leads to regioisomerization. Earlier work on the bicyclization of nonconjugated dienes containing at least a 1,1-di- or 1,1,2-trisubstituted alkene led to the formation of conjugated cis-dienylzirconocene species 20 via multipositional regioisomerization.[110] In other words, the nature and degree of substitution of the nonconjugated dienes strongly influence the course of the reaction. Further study[111] strongly supports the associative mechanism involving the formation of monocyclic zirconacyclopentane 21 by reaction of the diene 19 with the zirconacyclopropane 9, whereupon a ring contraction of the five-membered zirconacycle[100,112,113] affords the zirconacyclopropane 22 (▶ Scheme 4). An allylic C—H bond activation then furnishes a π-allyl(hydrido)zirconocene intermediate 23 (also seen as a σ-alkyl-π-allylzirconocene species) from which a rotation and reinsertion of the alkene into the Zr—H bond gives the new zirconacyclopropane 24.[114] This mechanistic pathway indicates that alkene–zirconocene complexes must be in dynamic equilibrium with 23. Repetition of this allylic C—H activation/rotation/alkene insertion sequence finally delivers the ziconocene complex 20.
Scheme 4 Mechanism of Multipositional Regioisomerization via an Allylic C—H Activation Sequence[114]

This type of chemical transformation can only take place in the absence of any other more facile processes and/or in the presence of some functionalities in the alkene–zirconocene species promoting regioisomerization.[115] Nevertheless, later studies suggest that it is in fact possible to combine this multipositional regioisomerization with numerous processes, leading to molecules that were hitherto difficult to access.
2.11.15.1 Method 1: Synthesis of Conjugated Dienes from Nonconjugated Dienes
2.11.15.1.1 Variation 1: From Nonheteroatom-Substituted Alkenes
The reaction of the Negishi reagent 4 with nonconjugated dienes 25 possessing substituted vinyl groups can either yield the bicyclization adducts or the dienylzirconocene complexes 26 via multipositional regioisomerization, and the free conjugated dienes 27 if the regioisomerization is followed by a decomplexation reaction with iodine (▶ Scheme 5).[111] The course of the reaction is heavily dependent on the nature and degree of substitution of the nonconjugated dienes. Indeed, the alkene group at the migration terminus must be either 1,1-di- or 1,1,2-trisubstituted to favor the formation of a single predominant dienylzirconocene complex and slow down or block an eventual zirconacyclization. Moreover, the rate of formation of the final product is greater in the case of a migration terminus corresponding to a 1,1,2-trisubstituted alkene than that of 1,1-disubstituted alkene, the latter leading to a more stable intermediate through chelating π-complexation. In each case, the conjugated dienylzirconocene product is s–cis, the diene moiety is >95% regio- and stereochemically pure, and the stereochemistry of 1,1,2-trisubstituted alkene moiety is E. This transformation is compatible with dienes with a three-, six-, and ninecarbon chain even though yields tend to decrease as the chain length increases. A free conjugated diene is obtained without the need of a decomplexation reaction with iodine when the migration terminus is a tetrasubstituted alkene.
R1 | R2 | R3 | n | Time (h) | Yield (%) | Ref | |
26a | 27b | ||||||
Me | H | H | 2 | 70 | 59 | – | [111] |
Me | Me | H | 2 | 18 | 78 | 79 | [111] |
(CH2)3 | H | 2 | 18 | 73 | 65 | [111] | |
(CH2)3 | H | 5 | 18 | 66 | n.d.c | [111] | |
(CH2)3 | H | 8 | 18 | 51 | – | [111] | |
Et | Et | Me | 2 | 18 | – | 73d | [111] |
a Determined by 1H NMR spectroscopy. | |||||||
b Isolated yield. | |||||||
c n.d. = not determined. | |||||||
d Yield of free conjugated diene without need of iodine treatment. |
[s–cis-(E)-l-(Pent-1-enyl)cyclopentene]zirconocene [26, R1, R2 = (CH2)3; R3 = H; n = 2]; Typical Procedure:[111]
To 1-(pent-4-enyl)cyclopentene [25, R1, R2 = (CH2)3; R3 = H; n = 2; 0.163 g, 1.28 mmol) and Zr(Cp)2Cl2 (0.402 g, 1.37 mmol) in THF (2 mL) was added a 2.62 M soln of BuLi in hexanes (1.05 mL, 2.75 mmol) at −78 °C. After 1h at −78 °C and 18 h at 20 °C, the yield was determined by1H NMR spectroscopy of the mixture using p-xylene as an internal standard. The lH NMR spectrum of the mixture showed two Cp signals at δ 5.00 and 5.51 ppm, whose quantitative analysis indicated the formation of the title compound in 73%.
(E)-l-(Pent-1-enyl)cyclopentene [27, R1, R2 = (CH2)3; R3 = H; n = 2]; Typical Procedure:[111]
The in situ generated conjugated dienylzirconocene complex 26 [R1, R2 = (CH2)3; R3 = H; n = 2] was treated with I2 (389.9 mg, 1.54 mmol) in THF at 0 °C. The mixture was diluted with Et2O, washed with aq Na2S2O3 and H2O, dried (MgSO4), and concentrated. The residue was purified by column chromatography; yield: 65%.
2.11.15.1.2 Variation 2: From Nonconjugated Dienes Bearing an Alkoxy Substituent
Nonconjugated dienes 28 bearing an alkoxy substituent undergo a tandem isomerization–elimination reaction to provide the corresponding metalated dienylzirconocenes, which can react with various electrophiles to afford conjugated dienes 29 (▶ Scheme 6). Reactions of such dienes are conducted in tetrahydrofuran at 50 °C for 15 minutes[116] or in diethyl ether at 35 °C for 30 minutes[117] when the Negishi reagent 4 is prepared by treatment of dichlorobis(η5-cyclopentadienyl)zirconium(IV) with butyllithium in the appropriate solvent at −78 °C. The elimination step takes place after the formation of a zirconacyclopentene produced by a rearrangement of a zirconacyclopropane.
Scheme 6 Preparation of Isomerically Pure Dienes from Nonconjugated Dienes Bearing an Alkoxy Substituent[116,117]

R1 | n | Config of 28 | Step 1 Conditions | Step 2 Conditions | E | Yielda (%) | Ref |
H | 1 | Z | THF, 50 °C, 15 min | 1 M HCl, rt | H | 80 | [116] |
H | 1 | Z | Et2O, 35 °C, 30 min | 1 M HCl, rt | H | 80 | [117] |
H | 1 | Z | THF, 50 °C, 15 min | I2 (4.4 equiv), −40 °C | I | 75b | [116] |
H | 1 | Z | Et2O, 35 °C, 30 min | NBS (4.4 equiv), −40 °C | Br | 60b | [117] |
H | 1 | Z | THF, 50 °C, 15 min | NCS (4.4 equiv), −40 °C | Cl | 60 | [117] |
H | 6 | E/Z | THF, 50 °C, 15 min | 1 M HCl, rt | H | 70 | [116] |
H | 5 | E/Z | THF, 50 °C, 15 min | 1 M HCl, rt | H | 70 | [117] |
(CH2)5Me | 5 | E/Z | THF, 50 °C, 15 min | 1 M HCl, rt | H | 61 | [116] |
a Isolated yield. | |||||||
b The product is unstable at room temperature and isomerizes with time. |
The reaction is stereoselective and always yields the E,Z-dienylzirconocene derivatives, e.g. 30, irrespective of the stereochemistry of the starting enol ether, via multiple allylic C—H activations, rearrangement, and elimination (▶ Scheme 7).[116,117] The method is also compatible with dienes with a six-carbon chain between the two double bonds and with the substrates whose migrating double bond is 1,2-disubsituted.
When a nonconjugated diene such as 31 is used, where the migrating group is geminal to the leaving group, the isomerization similarly occurs to furnish a zirconacyclopropane 32 but is followed by a β-elimination reaction to afford a β-metalated allenyl intermediate 33 which isomerizes into its more stable dienyl form 34 (where the alkyl and the organometallic groups are anti to each other) (▶ Scheme 8). Thus, the reaction of (E)-4-methoxydeca-1,4-diene (31) with the Negishi-type reagent 4 in tetrahydrofuran at 50 °C for 15 minutes gives the unique E,Z-conjugated diene 35 in 75% yield (▶ Scheme 8).
Conjugated Dienes 29; General Procedure:[116,117]
A 1.33 M soln of BuLi in hexane (1.8 mL, 2.4 mmol) was added dropwise to a stirred soln of Zr(Cp)2Cl2 (350.6 mg, 1.2 mmol) in anhyd THF (5 mL) at −78 °C under inert atmosphere. The temperature was slowly allowed to reach −50 °C and a soln of a nonconjugated dienol ether 28 (1.0 mmol) in anhyd THF (5 mL) was added dropwise, keeping the temperature below −50 °C. The cooling bath was removed, and the mixture was warmed as fast as possible to rt (a delay in warming the mixture may cause the formation of side products) and then heated at 50 °C for 15 min (when the experiment was performed in anhyd Et2O, the mixture was heated at 35 °C for 30 min) to provide the metalated diene ready for further use: I2 (1.116 g, 4.4 mmol), NBS (783.1 mg, 4.4 mmol), or NCS (587.5 mg, 4.4 mmol) could be added at −40 °C or, alternatively, 1 M HCl at rt. The layers were separated and the aqueous phase was extracted with Et2O (2 ×). The combined organic extracts were washed successively with sat. aq NaHCO3 and brine, and dried (MgSO4). The obtained residue was purified by column chromatography (silica gel).
(2E,4Z)-Deca-2,4-diene (35); Typical Procedure:[116,117]
A 1.37 M soln of BuLi in hexane (1.02 mL, 1.4 mmol) was added dropwise to a stirred soln of Zr(Cp)2Cl2 (208.6 mg, 0.714 mmol) in anhyd THF (15 mL) at −78 °C under inert atmosphere. The temperature was slowly allowed to reach −50 °C and a soln of (E)-4-methoxydeca-1,4-diene (31; 100 mg, 0.59 mmol) in anhyd THF (5 mL) was added dropwise, keeping the temperature below −50 °C. The cooling bath was removed, and the mixture was warmed as fast as possible to rt and heated at 50 °C for 15 min. The mixture was cooled to rt and 1 M aq HCl was added. The layers were separated and the aqueous phase was extracted with Et2O (2 ×). The combined organic extracts were washed successively with sat. aq NaHCO3 and brine, and dried (MgSO4). The obtained residue was purified by column chromatography (silica gel); yield: 75%.
2.11.15.2 Method 2: Synthesis of Trienes
The isomerization–elimination tandem sequence proceeds very efficiently with methoxysubstituted dienes 36 (n = 1) in tetrahydrofuran at 50 °C for 15 minutes to afford the resulting dienylzirconocene. After transmetalation with a copper salt, an allylation reaction gives the trienes 37 as unique E,Z-isomers in good overall yields (▶ Scheme 9).[117] Whatever the stereochemistry of the starting enol ether is, a unique isomer is obtained at the end of the process. Dienes 36 (n = 2, 3) with two- and three-carbon tethers also undergo this tandem transformation as fast as 4-(methoxymethylene)non-1-ene (36, n=1; R1 = H) to produce the corresponding E,Z-trienes 37 (n = 2, 3) in good yields. Lastly, when the migrating double bond is 1,2-disubstituted, the isomerization–elimination–transmetalation–allylation tandem sequence still proceeds very efficiently.
R1 | n | Config of 36 | Yielda (%) | Ref |
H | 1 | Z | 70 | [117] |
H | 1 | E | 72 | [117] |
H | 2 | E/Z | 70 | [117] |
H | 3 | E/Z | 71 | [117] |
Me | 1 | E/Z | 80 | [117] |
a Isolated yield. |
Trienes 37; General Procedure:[117]
A 2.05 M soln of BuLi in hexane (1.17 mL, 2.4 mmol) was added dropwise to a stirred soln of Zr(Cp)2Cl2 (350.6 mg, 1.2 mmol) in anhyd THF (17 mL) at −78 °C under inert atmosphere. The temperature was slowly allowed to reach −50 °C and a soln of a nonconjugated dienyl ether 36 (1 mmol) in anhyd THF (5 mL) was added dropwise, keeping the temperature below −50 °C. The cooling bath was removed, and the mixture was warmed as fast as possible to rt (a delay in warming the mixture may cause the formation of side products) and heated at 50 °C for 15 min to provide the metalated diene ready for further use. Freshly distilled allyl chloride (0.12 mL, 1.5 mmol), CuCl (9.9 mg, 0.1 mmol), and LiCl (85 mg, 2 mmol) were added at 0 °C. The soln was heated at 60 °C and stirred at this temperature for 1 h. An aqueous mixture of NH4Cl/NH4OH (2:1) was added at rt and the layers were separated. The aqueous phase was extracted with Et2O (2 ×). The combined organic extracts were washed successively with aq NaHCO3 and brine, dried (MgSO4), filtered, and concentrated. The crude residue was purified by column chromatography (silica gel, hexane).
2.11.15.3 Method 3: Synthesis of Homoallylic Alcohols
2.11.15.3.1 Variation 1: From Acid Chlorides without Ligand Exchange
The treatment of benzoyl chloride (38, Ar1 = Ph) with the Negishi-type reagent 4 in benzene at 0 °C for 3 hours furnishes 2-methyl-1-phenylbut-3-en-1-ol (39, Ar1 = Ph; R1 = Me) in 82% yield with a 31:69 syn/anti ratio (▶ Scheme 10).[118] Reactions with other aromatic acid chlorides 38 provide the corresponding homoallylic alcohols 39 in good yields except when electron-rich acid chlorides are used. The reactions works well with other Negishi-type reagents prepared from pentyl-, octyl,- and 3-phenylpropylmagnesium bromide instead of butylmagnesium bromide.
Ar1 | R1 | Yielda (%) | Ratio (syn/anti) | Ref |
Ph | Me | 82 | 31:69 | [118] |
Ph | Et | 87 | 25:75 | [118] |
Ph | (CH2)4Me | 92 | 25:75 | [118] |
Ph | Ph | 91 | 23:77 | [118] |
2-Tol | Me | 63 | 29:71 | [118] |
4-ClC6H4 | Me | 67 | 33:67 | [118] |
2-furyl | Me | 60 | 42:58 | [118] |
4-MeOC6H4 | Me | 22 | 36:64 | [118] |
4-F3CC6H4 | Me | 65 | 38:62 | [118] |
a Isolated yield. |
It is suggested that the crotylzirconocene hydride 41, which is in equilibrium with the Negishi-type reagent 40 through a reversible allylic C—H bond cleavage, undergoes an allylation reaction after a hydride attack on the aroyl chloride, most likely via a six-membered transition state (▶ Scheme 10).
Homoallylic Alcohols 39; General Procedure:[118]
Benzene (20 mL) (CAUTION: carcinogen) was added to Zr(Cp)2Cl2 (585 mg, 2.0 mmol) in a 50-mL reaction flask under argon. The mixture was cooled to 0 °C in an ice–water bath and a 1.0 M soln of an alkylmagnesium bromide in THF (4.0 mL, 4.0 mmol) was added. The soln immediately turned into a viscous black suspension, and the resulting mixture was stirred for 30 min at 0 °C. The acid chloride 38 (1.0 mmol) was then added at 0 °C, and the mixture was stirred for another 3 h at 0 °C. The mixture was poured into 3 M aq HCl (50 mL) and extracted with hexane/EtOAc (5:1; 3 × 25 mL). The combined organic layer was dried (Na2SO4) and concentrated under reduced pressure. The crude oil was purified by chromatography (silica gel, hexane/EtOAc).
2.11.15.3.2 Variation 2: From Acid Chlorides with Ligand Exchange
The transformation of benzoyl chloride into the homoallylic alcohol 2-ethyl-1-phenylbut-3-en-1-ol (43, R1 = Et) proceeds smoothly by ligand exchange when dichlorobis(η5-cyclopentadienyl)zirconium(IV) is treated with cyclopentylmagnesium bromide in benzene at 0 °C for 30 minutes in the presence of pent-1-ene (42, R1 = Et). Numerous terminal alkenes 42 can take part in the ligand exchange to produce the corresponding homoallylic alcohols 43 (▶ Scheme 11).[118] In addition, reduction of dichlorobis(η5-cyclopentadienyl)zirconium(IV) with magnesium metal in the presence of an alkene 42 in tetrahydrofuran at 0 °C for 15 minutes[119–121] and subsequent addition of benzoyl chloride and benzene as a cosolvent at 0 °C gives similar results, but offers the advantage of avoiding the preparation of Grignard reagents and tolerates ester and amide moieties (▶ Scheme 11).[118]
R1 | Conditions (Step 1) | Time (h; Step 2) | Yielda (%) | Ratio (syn/anti) | Ref |
Et | Zr(Cp)2Cl2 (2 equiv), cyclopentylmagnesium bromide (4 equiv), benzene, 0 °C, 30 min; then 25 °C, 1 h | 3 | 76 | 25:75 | [118] |
Et | Zr(Cp)2Cl2 (3 equiv), Mg (4.5 equiv), 1,2-dibromoethane (0.45 equiv), THF,0 °C, 3 h | 0.5 | 77 | 23:77 | [118] |
(CH2)4Me | Zr(Cp)2Cl2 (3 equiv), Mg (4.5 equiv), 1,2-dibromoethane (0.45 equiv), THF,0 °C, 3 h | 0.5 | 62 | 26:74 | [118] |
Pr | Zr(Cp)2Cl2 (2 equiv), cyclopentylmagnesium bromide (4 equiv), benzene, 0 °C, 30 min; then 25 °C, 1 h | 3 | 74 | 20:80 | [118] |
Pr | Zr(Cp)2Cl2 (3 equiv), Mg (4.5 equiv), 1,2-dibromoethane (0.45 equiv), THF,0 °C, 3 h | 0.5 | 75 | 23:77 | [118] |
SiPh3 | Zr(Cp)2Cl2 (2 equiv), cyclopentylmagnesium bromide (4 equiv), benzene, 0 °C, 30 min; then 25 °C, 1 h | 3 | 31 | 0:100 | [118] |
(CH2)OBn | Zr(Cp)2Cl2 (2 equiv), cyclopentylmagnesium bromide (4 equiv), benzene, 0 °C, 30 min; then 25 °C, 1 h | 3 | 32 | 23:77 | [118] |
(CH2)2OBn | Zr(Cp)2Cl2 (3 equiv), Mg (4.5 equiv), 1,2-dibromoethane (0.45 equiv), THF,0 °C, 3 h | 0.5 | 62 | 23:77 | [118] |
![]() | Zr(Cp)2Cl2 (3 equiv), Mg (4.5 equiv), 1,2-dibromoethane (0.45 equiv), THF, 0 °C, 3 h | 0.5 | 67 | 22:78 | [118] |
![]() | Zr(Cp)2Cl2 (3 equiv), Mg (4.5 equiv), 1,2-dibromoethane (0.45 equiv), THF, 0 °C, 3 h | 0.5 | 61 | 26:74 | [118] |
a Isolated yield. |
Homoallylic Alcohols 43; General Procedure Using Cyclopentylmagnesium Bromide:[118]
A 1.0 M soln of cyclopentylmagnesium bromide in THF (4.0 mL, 4.0 mmol) was added to a soln of Zr(Cp)2Cl2 (585 mg, 2.0 mmol) and a terminal alkene 42 (6.0 mmol) in benzene (20 mL) (CAUTION: carcinogen) at 0 °C. The mixture was stirred at 0 °C for 30 min and then at 25 °C for an additional 1 h. The resulting mixture was cooled to 0 °C, and BzCl (141 mg, 1.0 mmol) was added. The mixture was stirred for 3 h at 0 °C, poured into 3 M aq HCl (50 mL), and extracted with hexane/EtOAc (5:1; 3 × 25 mL). The product was purified by column chromatography (silica gel).
Homoallylic Alcohols 43; General Procedure Using Magnesium Metal:[118]
THF (1 mL) was added to Zr(Cp)2Cl2 (877 mg, 3.0 mmol) and Mg metal (109 mg, 4.5 mmol) in a 50-mL flask filled with argon. 1,2-Dibromoethane (85 mg, 0.45 mmol) was added to activate the Mg metal, and the mixture was stirred for 15 min. The soln was cooled to 0 °C and a soln of a terminal alkene 42 (6.0 mmol) in THF (4 mL) was added. The mixture was stirred for 3 h at 0 °C to form the alkenylzirconocene complex. Benzene (25 mL) (CAUTION: carcinogen) was then added as cosolvent, and BzCl (141 mg, 1.0 mmol) was added dropwise. The resulting mixture was stirred for 30 min at 0 °C. The reaction was quenched with 3 M aq HCl and the separated aqueous phase was extracted with hexane/EtOAc (5:1; 3 × 25 mL). The solvent was removed and the residue was purified by column chromatography (silica gel).
2.11.15.3.3 Variation 3: From Aldehydes without Ligand Exchange
(Allyl)bis(η5-cyclopentadienyl)hydridozirconium complexes 46 generated by reaction of dichlorobis(η5-cyclopentadienyl)zirconium(IV) with Grignard reagents (e.g., 44) in toluene at 0 °C react with diisopropyl ketone to afford (alkoxy)(allyl)zirconium reagents 47. These intermediates 47 react smoothly with aldehydes to lead to the corresponding homoallylic alcohols 45 via a stereoselective allylation reaction, giving as major product the anti-isomer at −78 °C and the syn-isomer at 25 °C (▶ Scheme 12).[122] This protocol is unique because the reaction of aldehydes with alkoxyzirconium reagent 47, where the hydride is not transferred to a bulky ketone, gives a mixture of products. The formation of the anti-isomer can be rationalized by kinetic control via a six-membered transition state 48. Warming up the mixture before aqueous workup reverses the diastereoselectivity because the syn-isomer is then formed by isomerization of the anti-adduct through a retroallylation–allylation sequence (▶ Scheme 12).
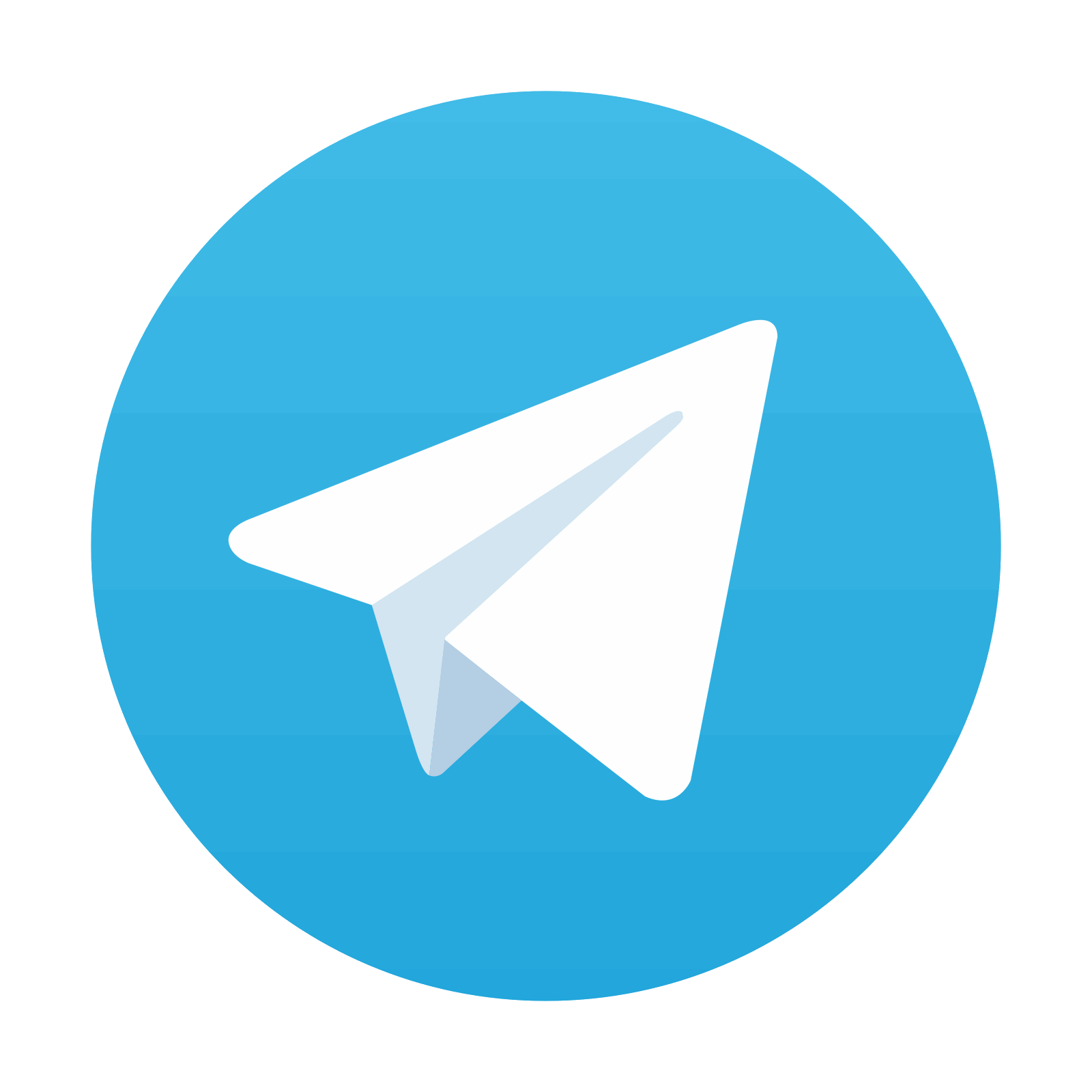
Stay updated, free articles. Join our Telegram channel

Full access? Get Clinical Tree
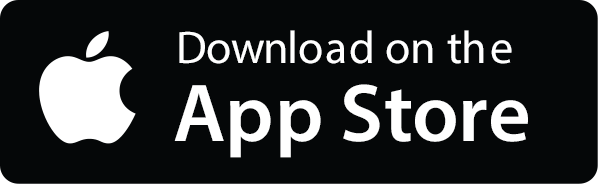
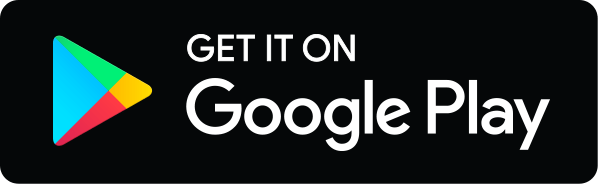