29 Tope Adeniyi Cryopreservation can be defined as the process by which living cells, tissues, and organs are preserved in a stable or viable state with the use of appropriate cryoprotecting agents (CPA) at extremely low temperatures. The application of cryopreservation procedures was first reported as early as the sixteenth century (Sherman 1954; Sherman 1963) and by Spallanzani, a renowned Italian physiologist, who attempted to cryopreserve semen using snow in 1776. In 1949, Polge et al. reported an accidental, albeit, significant breakthrough in the process of cryopreservation when they discovered the potent cryoprotective potential of glycerol at extremely low temperatures in an attempt to successfully cryopreserve mammalian sperm (Polge et al. 1949). A growing interest in the field of cryobiology led several subsequent researchers to confirm that sperm cells can be frozen and thawed successfully; these findings were consistent and reproducible (Lovelock 1953; Bunge et al. 1954; Lovelock and Polge 1954; Sherman 1954; Mackenzie and Luyet 1967). Nevertheless, cryopreservation of both male and female gametes in assisted reproductive treatment (ART) remains an area of ongoing research to further improve current outcomes and to also ensure that the procedure is safer for patients and the children born through ART. The process of gamete cryopreservation has evolved progressively over the last two centuries and its application has been embraced within animal breeding, conservation programmes, and human medicine, including ART. The cryopreservation procedures of ART involve exposure to CPA, cooling of gametes and embryos, storage at extremely low temperatures (‐196 °C), and thawing or warming. Water is the universal solvent and a major component of all living cells. Water also presents the potential for formation of dangerous ice crystals as the surrounding environment is super‐cooled, at temperatures below freezing point. Earlier investigations by Mazur described the association between the formation of ice crystals at low temperatures in a super‐cooled solution and its effects on biological cells (Mazur 1963). Following these investigations, the initial stages of freezing essentially involve the gradual removal of water molecules from within the intracellular environment to avoid cryoinjury or damage due to the formation of ice crystals inside the cell. The formation of ice crystals inside a cell can be avoided with the use of appropriate CPA, which safely dehydrates the cell as a result of the osmotic gradient created between the internal cellular environment and the external environment (freezing solution). This facilitates the movement of water molecules from inside the cell by osmosis outward across the cell membrane into the external cellular environment until equilibrium is reached. Subsequently, as the temperature of the surrounding environment continues to decrease, formation of ice begins to spread in the external environment. This concentrates the solute and the freezing solution becomes viscous, inevitably initiating the migration or removal of possible residual water molecules from inside the cell into the external environment and inducing changes in cell volume as the cell begins to shrink during the process of cryopreservation (Figure 29.1). However, it is important to note that the complete or partial removal of excessive water molecules beyond what cells can tolerate for viability and membrane integrity can also be detrimental to cell survival. Figure 29.1 Cryopreservation. (1) Cells prepared for cryopreservation are placed in a cryomedium containing cryoprotectant. (2) As the temperature decreases, ice crystals form in the extracellular media, (3) which subsequently increase solute concentration causing osmotic dehydration. (4) Cells continue to shrink while losing intracellular water. (5) Successful cryopreservation is achieved with little or no ice crystal formation. (6) Vitrification of cells is carried out in medium with very high concentrations of cryoprotectant, thus minimizing any cryodamage. (7) In the absence of any cryoprotectant, the formation of intracellular ice crystals will damage cellular structures, ultimately killing the cells. Leibo et al. demonstrated that the rate of ice crystal formation during freezing is dictated by the cooling rate and the permeability of the given cell membrane to water molecules (Leibo et al. 1978; Leibo 1984). These findings have been shown to be very critical to the survival of any biological cell. Therefore, it is essential for the optimum cooling rate to be adequate and slow enough to allow the progressive withdrawal of water molecules from inside the cell so that excessive residual water molecules, which can form harmful intracellular ice crystals as the temperature decreases, are not trapped inside the cell. Similarly, if the cooling rate is too fast this may not allow appropriate migration of water molecules from inside the cell into the external environment, resulting in the formation of ice crystals within the intracellular environment causing cryodamage to cellular organelles. However, the avoidance of harmful ice crystals at this initial stage does not completely circumvent this problem, as recrystallization of ice has been shown to occur during thawing (Mazur 1990; Mazur and Kleinhans 2008; Mazur 2010). Therefore, the use of an appropriate combination of either permeating or nonpermeating cryoprotectants at a safe concentration is critical to avoiding rapid rehydration of the cell, which eventually causes the cell membrane to rupture. Despite these challenges, human gametes and embryos have been safely and successfully cryopreserved for more than 35 years with arguably optimized procedures (Bagchi et al. 2008; Aflatoonian et al. 2010; Argyle et al. 2016). However, for effective cryopreservation it is important to avoid or minimize deleterious conditions associated with cryopreservation, which can be achieved through the following approaches: (i) use CPA to maintain cellular membrane fluidity and stability during osmosis; (ii) eliminate or reduce the formation of harmful intracellular and extracellular ice crystals; (iii) substitute intracellular water molecules with CPA and; (iv) reduce cellular toxicity of CPA at high or low temperatures. Adhering to these practices can significantly reduce the impact of osmotic shock and cryoinjury and increased freeze–thaw survival rates for gametes and embryos in ART (Edgar et al. 2009; Wennerholm et al. 2009; Edgar and Gook 2012; Kopeika et al. 2015). Routinely, cryopreservation media used in ART involves the use of cryoprotectants and can be classified into two different groups based on the mechanism of action during cryopreservation: (i) permeating and (ii) nonpermeating cryoprotectants. The effectiveness of permeating cryoprotectants such as glycerol, dimethyl sulfoxide (DMSO), 1,2‐propandiol (PROH), and ethylene glycol in preventing or minimizing cryoinjury can be attributed to the high membrane permeability of these cryoprotectants and their ability to displace water molecules from the intracellular environment, in addition to stabilizing the concentration of harmful electrolytes within intracellular spaces. On the contrary, nonpermeating cryoprotectants such as sucrose, trehalose, polyvinylpyrrolidone (PVP), and lactose are unable to permeate cell membranes due their large molecular weight and size. Hence, they facilitate significantly the withdrawal of water molecules from the intracellular environment via an osmotic gradient, in addition to reducing the risks of formation of harmful intracellular ice crystals. However, both groups of cryoprotectants may indirectly inflict cryoinjuries or osmotic shock on human reproductive gametes and embryos (Szurek and Eroglu 2011; Vanderzwalmen et al. 2013). Identification of the appropriate concentration, the type of cryoprotectants, and the cooling/warming rates have been identified as some of the initial challenges of cryopreservation especially vitrification procedures (Lovelock 1953; Edgar et al. 2009; Edgar and Gook 2012). The pioneering works of Polge et al. (1949) confirmed the ability of specific compounds to protect sperm cells from osmotic shock or cryoinjury at extremely low temperatures. Moreover, the discovery of the cryoprotective properties of glycerol and the use of similar CPA has now become routine practice in cryobiology. The unique properties of CPA to facilitate a chain of both physical and chemical events that allow for successful cryopreservation and storage of reproductive cells at extremely low temperatures and viability upon thawing include the following: high solubility in water, membrane permeability, and low toxicity at low temperatures (Lovelock 1953; Lovelock and Polge 1954; Kopeika et al. 2015). In an attempt to minimize the potential damage associated with cryopreservation, freezing media used in ART for cryopreservation of gametes routinely contains permeating and nonpermeating CPA, physiological buffer, human serum albumin and inhibitors of apoptosis such as vitamin E, melatonin, and ascorbic acid. The primary objective of cryopreservation media used in ART is to reduce the risks of cellular damage whilst ensuring cell survival and viability postcryopreservation. Therefore, the composition of cryopreservation media is arguably critical to gamete survival as cryopreservation procedures are associated with nonphysiological chemical and physical changes which may compromise cell survival and normal cellular and gamete development after thawing or warming (Aye et al. 2010; de Menorval et al. 2012). Unfortunately, detailed compositions of most commercially available human embryo cryopreservation media have not been properly documented by some manufacturers (Harper et al. 2012; Sunde and Balaban 2013). This lack of proper documentation and transparency has raised significant concerns among leading researchers in ART and some professional bodies such as the European Society of Human Reproduction and Embryology (ESHRE) and Alpha Scientists in Reproductive Medicine (Magli et al. 2008; Balaban et al. 2012). Nonetheless, available knowledge on the basic principles of cryobiology and the role/function of each essential component suggests the following as the basic composition of cryopreservation media. Cryoprotecting agents (permeating and nonpermeating) primarily protect gametes from cryopreservation‐related osmotic shock, which may result in cellular damage or injuries. Briefly, permeating cryoprotectant such as ethylene glycol penetrates the cell and displaces water molecules, and also forms a stable hydrogen bond with intracellular residual water molecules. This event significantly lowers the freezing point of water molecules and reduces the formation of deleterious ice crystals. Similarly, nonpermeating CPA such as sucrose and trehalose withdraw water molecules from the intracellular environment via an osmotic gradient. This prevents the risks of formation of harmful ice crystals during cryopreservation. Gametes cryopreservation media used in ART routinely contain a combination of appropriate concentrations of permeating and nonpermeating cryoprotectants. This combination reduces the risks of cytotoxicity of cryoprotectants and ice crystal formation, or recrystallization within the intracellular environment of the gametes during freezing, thawing, and warming (Vanderzwalmen et al. 2013; Kopeika et al. 2015). Cryopreservation procedures are often associated with oxidation stress arising from Reactive Oxygen Species (ROS). These are free radicals with a potentially harmful impact that may compromise sperm function, survival, and viability as a result of impaired structural and DNA damage following cryopreservation (Said et al. 2010). Impaired membrane integrity or mechanical damages as result of ROS, osmotic shock, or cryoprotectant toxicities have been linked with reduced sperm motility, and poor oocyte and embryo survival and development (Martinez‐Burgos et al. 2011). Hence supplementation of cryopreservation media with the appropriate concentration of antioxidants has been shown to facilitate successful freezing and thawing of human reproductive gametes and embryos (Lane et al. 2002; Taylor et al. 2009). Antioxidants (enzymes and nonenzymes) are essential inhibitors of apoptosis and potential DNA damage. In fact, the addition of antioxidants such as vitamin E (α‐tocopherol), ascorbic acid, selenium, and ubiquinones to cryopreservation and culture media used in ART have been advocated in order to reduce the impact of the in vitro culture environment and micromanipulation‐related damage (Taylor et al. 2009; Agarwal et al. 2014; Amidi et al. 2016). According to Agarwal et al. (2014) evidence suggesting the efficacies of antioxidants in oocyte and embryo cryopreservation procedures may be apparent. However, the establishment of this evidence following a large and properly designed randomized controlled clinical trial remains essential in ART. The appropriate concentration of a suitable salt remains an essential component of cryopreservation media and various micromanipulation media used in ART. However, sodium (Na+) is thought to cause significant damage to the cell membrane and its integrity, as the removal of the high cellular concentration of sodium salts is energy dependent via the sodium pump. This critical energy dependent procedure may be significantly disrupted during cryopreservation, which may compromise cell survival. An experimental study by Toner et al. showed that mouse oocytes failed to survive the thawing procedure in a hypertonic sodium chloride (NaCl) solution supplemented with phosphate buffered saline solution without a cryoprotecting agent (Toner et al. 1993). Although, this observation may be different in the presence of a cryoprotecting agent (Arcarons et al. 2016), nonetheless this experimental study by Toner et al. suggested that an isotonic solutions of NaCl may not be adequate in preventing cell lysis. Unpublished data involving the use of choline chloride as a substitute for sodium chloride showed a contrary observation as the majority of oocytes remained intact at room temperature following thawing in the absence of a cryoprotectant (Stachecki et al. 1998; Gardner et al. 2007; Lin et al. 2009). A related case report (Quintans et al. 2002) involving the successful live birth of two babies, used a media in which NaCl was substituted by choline chloride (ChCl). This clinical evidence suggested that cryopreservation media with low Na+ concentration might be a more beneficial component in the media. Unlike Na+, choline salts are nonmembrane penetrating cations which efficiently remain in the external environment preventing the influx of cations into the intracellular environment during freezing and thawing (Stachecki et al. 1998). pH buffers such as 3‐(N‐morpholino) propanesulfonic acid (MOPS) and 4‐(2‐hydroxyethyl)‐1‐piperazine‐ethane sulfonic acid (HEPES) are very critical chemical substances with unique abilities to stabilize changes in pH which may be associated with in vitro culture or gamete handling outside the incubator environment such as the cryopreservation procedures of ART. Alteration in pH levels arising from changes in temperature and osmolarity in the immediate environment of the gamete is often associated with cryopreservation. Biological pH buffers are essential in providing a relatively stable physiological pH environment (pH 6–8) for most gamete micromanipulation procedures (Will et al. 2011). Furthermore, this role is critical as nonphysiological pH may compromise gamete membrane integrity, normal embryo development, and post‐thaw sperm motility. The inclusion of protein molecules in the form of Human Serum Albumin (HSA) enhances the viscosity of the cryopreservation media. Important macromolecules such as antifreeze proteins provide an additional source of cryoprotection by reducing the growth and formation of ice crystals during the cooling stages of cryopreservation procedures. Also, they assist in limiting the impact of osmotic shock experienced by cells and gametes during cryopreservation (Elliott et al. 2017). Furthermore, macromolecules such as glycoproteins are effective in protecting cell surfaces during cryopreservation in addition to facilitating appropriate gamete and embryo handling which prevents gametes and embryos from sticking to plastic wares and carrier devices during macromanipulation. Human sperm freezing media used in ART are essentially formulated for the maintenance and preservation of sperm function and viability, and DNA integrity for continuity of progeny. These are critical objectives which facilitate optimum sperm survival after cryopreservation with significant impact on sperm functional viability, motility, and treatment outcomes (Amidi et al. 2016). In severe conditions, these cryopreservation related changes may culminate in cell death by apoptosis or total loss of sperm motility (Said et al. 2010). Also, potential deviations from sperm cryopreservation procedures may compromise the ability of the human sperm to successfully activate the metaphase II (MII) oocyte for normal fertilization to occur (Ducibella et al. 2002; Ozil et al. 2005; Gardner et al. 2007). A similar observation has been reported in several experimental studies involving cryopreservation (vitrification) of mouse and human MII oocytes, and subsequent normal embryo development (Ducibella et al. 2002; Gardner et al. 2007; Larman et al. 2007a). These studies highlight the importance of oocyte activation and fertilization processes as a prerequisite to normal gametes and embryo development and how susceptible these critical processes may become to the elements of cryopreservation. Nonetheless, current cryopreservation media used in ART remain efficient, yielding acceptable survival and clinical pregnancy rates with successful live births (Boldt 2011; Cobo et al. 2014; Nagy et al. 2016). The presence of Ca2+ in cryopreservation media has been implicated in premature cortical granule exocytosis leading to hardening of the zona pellucida (ZP) (Larman et al. 2006). However, this challenge may be circumvented by the intracytoplasmic sperm injection (ICSI) procedure. Nonetheless, the use of Ca2+‐free cryopreservation media in ART has been advocated due to concern over premature ZP hardening and potential parthenogenetic activation of the oocyte (Larman et al. 2004; Ozil et al. 2005). Several studies have also reported cryopreservation‐related changes to the oocyte’s spindle structure and integrity, thickening of the ZP, repositioning of the polar body, and chromosome misalignment (Larman et al. 2007b; Cobo et al. 2008c; Bromfield et al. 2009). Some of these changes are often irreversible and may be partly responsible for suboptimal fertilization outcomes or complete fertilization failure occasionally observed with frozen–thawed oocytes (Larman et al. 2007a). Regardless of these observations it remains plausible if these observations are the result of the physical and chemical changes associated with cryopreservation procedures or a product of a specific component of the cryopreservation media (Katz‐Jaffe et al. 2008; Larman et al. 2011). However, available clinical data obtained from ART cryopreservation procedures remains reassuring despite the association of these potentially detrimental impacts with the cryopreservation procedure (Cobo and Diaz 2011; Garcia‐Velasco et al. 2013; Wennerholm et al. 2013). In 1986 Chen reported the first successful cryopreservation of the human MII oocyte using the traditional/slow method of cryopreservation (Chen 1986). This report led to a successful clinical pregnancy and a twin live birth. Prior to this report, cryopreservation of the human oocyte was relatively unsuccessful and inefficient compared with cryopreservation of the human cleavage and blastocyst embryo (Cobo et al. 2008a; Brambillasca et al. 2013). This observation was similar to the different stages of the human oocyte. The initial slow freezing method of oocyte cryopreservation was associated with suboptimal post‐thaw survival rates and clinical outcomes. Following this consistently low outcome over a decade it became apparent that applying the same protocol used in cleavage stage embryo freezing was inadequate for successful oocyte freezing, due to its unique physiology and structure (Almodin et al. 2015). Hence, the slow freezing procedure for oocyte cryopreservation was subsequently modified (Gook et al. 2016). Marginal improvement in post‐thaw survival rates and clinical outcomes were observed following these modifications. A large multicentre observational study which compared clinical outcomes with slow frozen–warmed oocytes indicated that oocyte slow freezing may be associated with reduced efficacy as a result of lower clinical pregnancy per thaw oocyte (4.3%) and an implantation rate of 3.3%, (Borini et al. 2010). Regardless of these modifications, the fertilization, clinical pregnancy, implantation, and live birth rates were still considered very low relative to those obtained in treatment cycles involving fresh oocytes (Fabbri et al. 2001; De Santis et al. 2007). The application of this slow freezing method of oocyte cryopreservation was routine practice in Italy due to the legal restriction on cryopreservation of human oocytes between 2004 and 2009 (Borini et al. 2010; Levi Setti et al. 2014). The high water content of the oocyte, low surface area to volume ratio, the suspension of temperature sensitive cellular organelles (e.g. meiotic spindle) within the cytoplasm, and the delicate plasma membrane of the human oocyte are some of the unique features of the oocyte which presented initial challenges to successful cryopreservation of oocytes (Konc et al. 2014). This initial challenge seems to have been significantly overcome with the advent of open and closed systems of vitrification (Bagchi et al. 2008; Arav and Natan 2013). Kuleshova et al. (1999) reported the first successful birth following the application of vitrification methods of cryopreservation in ART. Oocyte cryopreservation offers a very unique and significant benefit compared with those associated with embryo cryopreservation. It allows cryopreservation of oocytes which otherwise would have been discarded due to lack of a semen sample from the male patient following successful controlled ovarian stimulation and egg retrieval from the female patient. Similarly, cryopreserved oocytes can be thawed–warmed and used in cases of an absentee husband and in some extreme cases it facilitates the posthumous use of cryopreserved oocytes. Importantly, cryopreservation of oocytes offers female reproductive autonomy, in addition to avoiding possible ethical or religious challenges associated with embryo cryopreservation or disposal after the consented period of storage. The benefits and applications of oocyte cryopreservation remain critical in modern reproductive science and management of female infertility, such as fertility preservation and delayed childbearing. Hence, increasing numbers of babies are being born from the application of the procedure. To date, over 1500 babies are reported to have been born worldwide following the application of vitrification methods of cryopreservation in ART. Some investigators argue that this figure is an underestimate, as significantly higher numbers of babies are reported to have been born from the application of both slow and vitrification methods of cryopreservation in Italy alone (Herrero et al. 2011). A related retrospective study which includes data from the Italian Birth Registry by Levi Setti et al. (2014) reported the live births of 1338 babies with 778 and 556 arising from the application of slow freezing and vitrification procedures, respectively, between 2007 and 2011. This live birth data was associated with 14 328 frozen–thawed–warm oocyte treatment cycles in which a total of 11 599 patients had at least one embryo transferred. Similar live birth data following the application of oocyte cryopreservation has been reported in other studies (Cobo and Diaz 2011). In the UK, the Human Fertilisation and Embryology Authority (HFEA) (2013 and 2015) report indicated that an estimated 60 babies have been born from the application of both slow frozen–thawed and vitrified–warmed oocytes. Whilst this data may indicate that application of oocyte cryopreservation may not be routine in the UK, some treatment‐licenced centres have reported a surge in the application of the procedure for medical or nonmedical reasons (HFEA Trends and Figures, 2015 report). A prospective randomized sibling study involving fresh and vitrified–warmed MII oocytes by Rienzi et al. (2017) did not report any statistical differences in fertilization rate, pronuclei morphology, embryo development, and quality between the two different study groups. Also, similar ongoing clinical pregnancy per transfer and implantation rate per cycle of 30.8 and 20.8% versus 30.0 and 17.0% were reported in the vitrified–warmed and fresh treatment groups, respectively. This data suggest that treatment outcomes following oocyte vitrification are not inferior to those obtained in fresh treatment cycles. These findings were largely in agreement with a systematic review and meta‐analysis of randomized controlled trials involving the clinical application of oocyte vitrification in ART by Cobo and Diaz (2011). However, the later study seems to suggest that vitrification methods are a more efficient means of oocyte cryopreservation. Different protocols have been reported with successful oocyte and embryo cryopreservation procedures in ART (Edgar and Gook 2012). These protocols are unique in terms of the concentration and type of cryoprotecting agent, the duration of exposure, and the type of carrier (straw) device used. Regardless of these variations or similarities in the procedure, cryopreservation procedures in ART equilibrium (slow freezing) and nonequilibrium (vitrification) are both established on the same basic principles of cryopreservation. These procedures involve initial cellular dehydration using a combination of CPA at different concentrations followed by exposures to appropriate cooling temperatures and subsequent storage in liquid nitrogen (LN) (Rienzi et al. 2017
Cryopreservation of Gametes and Embryos
Introduction: A Brief History of Gamete Cryopreservation
Principles of Cryopreservation
Permeating and Nonpermeating Cryoprotectants in ART
Freezing Media and Cryopreservation in ART
Cryoprotecting Agents
Inhibitor of Apoptosis
Salts
Buffers
Macromolecules
Cryopreservation Media and Related Challenges
Gamete and Embryo Cryopreservation
Oocyte Cryopreservation
Stay updated, free articles. Join our Telegram channel

Full access? Get Clinical Tree
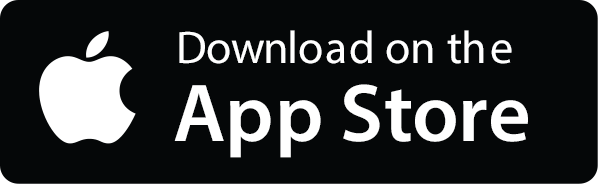
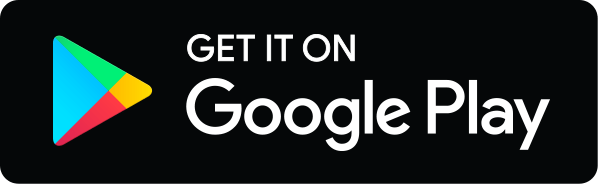