Hank Rahe and Brian G. Ward
CONTENTS
Defining Drugs in Terms of Contamination Risk
Risk Assessment by NIOSH and the European Medicines Agency
Comparison of Exposure Risk to Isolation Technology Solutions
Open-Front Unidirectional Airflow Devices
INTRODUCTION
Containment technology reaches across the entire health care spectrum, ranging from discovery to patient delivery. New drugs and delivery systems for drugs require the two critical elements that containment (or isolation) technology provides, namely, protection of the product and protection of personnel who produce the drugs. Since drugs are becoming more potent, exposure to small amounts can result in deleterious effects. Innovative delivery technologies create an even greater challenge, with final dosage forms that focus on specific targets in the body, creating a higher risk of exposure for health care personnel involved in the delivery of these drug forms to the intended patient.
The ability to measure small quantities of the active pharmaceutical ingredient (API) has resulted in a closer examination of the potential for cross-contamination. Drugs that can cause an adverse reaction or, even more critical, a nonreversible effect at low doses are becoming more common. The potency of these new drugs has added new dimensions for cleaning validation. Detection in the picogram (one trillionth of a gram) range of the drugs has also created new concerns with sample collection, measurement, and evaluation.
Additional factors that have created a need for isolation technology are waste minimization, which has become a priority, increased cost of raw materials, and the need for more extensive processing, all of which add significantly to the cost of the final product. The combination of the need for product protection and personnel protection, cross-contamination risk, waste reduction, and cost of the drug results in the need for isolation systems. The capability of the isolation system must meet the need for containment of airborne active drug substances to less than 1 ng/m3.
Terms such high containment have little value when determining an effective approach to product or personnel protection. Defining the risk potential by using risk assessment tools leads to better solutions. This chapter addresses the technological solutions for minimizing drug exposure from material release. It also explores the means of evaluating the capabilities of isolation technology solutions, measurement techniques to determine how much drug is present, and the risks created by cross-contamination of the drug.
DEFINING DRUGS IN TERMS OF CONTAMINATION RISK
“The dose makes the poison” was first expressed by Paracelsus (Philippus Aureolus Theophrastus Bombastus von Hohenheim, 1493–1541), who intended the comment to communicate a basic principle of toxicology, which is that a substance can produce harmful effects associated with its toxic properties only if it reaches a susceptible biological system within the body in a high enough concentration. The basic principle of pharmaceuticals is to create a positive, not negative, effect on the patient. To accomplish the positive effect, the correct dosage for the intended patient is carefully designed and tested by the pharmaceutical manufacturing company. Extensive testing is conducted also to determine what quantity of the drug will create a negative effect. The negative effect can come in several different ways: a person can consume a higher than intended dose, or personnel working with and around the drug can be exposed to a level that will create a negative effect. The entire life cycle of the drug, from discovery to administration to the patient, is associated with exposure risks.
Understanding of the difference between the dose that makes the poison and that which benefits patients is far from simple. At the early stages of drug development, researchers are looking for a compound that creates a focused positive effect on a given category of disease. This process results in searches of thousands of compounds, looking for one that has the desired effect on the target disease. Along with the positive effect also comes a negative effect based on the quantity of the compound to which one is exposed. Once a compound is selected for further evaluation, testing begins and follows an extensive routine to determine both effectiveness and side effects. Initial containment assessments include only information available from limited toxicology tests and compounds that are chemically similar to determine the hazard potential of a compound.
Initial dose–response studies help to determine the point at which a positive effect is observed. As the dose is increased, there is an increase in the incidence and severity of adverse effects. Containment protection of personnel working with the compound at this stage is most important because the exposure risk is not well understood. In these early stages of drug development, a risk factor that fits the class of compound should be assigned based on worst-case assumptions, and the proper engineering controls that limit exposures should be implemented.
COMMUNICATION OF RISK
Communication of risk, using banding, was created in the 1990s in an attempt to place compounds in groups or bands that could then be associated with specific engineering technologies. A typical band defines a range of occupational exposure limits (OELs) that the given engineering control is capable of achieving. Individual companies created definitions of bands, ranging from three to six categories of exposure limits. Depending on category or band assigned to a specific drug, there was a significant economic impact on facilities that handled the drug.
The early primary containment focus was on powders, as it was believed that airborne particulates represented the greatest risk of exposure to workers. Studies were conducted to determine why different compounds produced greater exposure risks when processed in identical equipment. The results of the studies concluded that particle characteristics play a critical role with airborne emissions in terms of particle size, shape, and density that directly impact the ability of the particle to become and stay airborne [1]. The “dustiness index” describes the results of testing dust, using a Heubach dust meter. The Heubach method most appropriately simulates the particle behavior characteristic of those generated during drug manufacturing processes.
Liquids were considered a lower risk of airborne exposure until studies showed that when a liquid was placed under pressure, such as during the filling operation, liquid that detached from the filling needles could produce airborne aerosols. This risk also exists in the compounding transfer from drug vials to the final delivery package. Concerns about the risk of compounds transforming into vapors have been raised with the antineoplastic drug class by the National Institute for Occupational Safety and Health (NIOSH). Vapors can only be created during a phase change from solids and liquids to a gas as a result of pressure and temperature changes. Therefore, it is very unlikely that this phase change is significant at room temperature during normal manipulations that occur in transferring the drug from a vial to the final patient delivery package. An article written by a NIOSH team member stated that vapors could be produced during manipulation of some antineoplastic drugs, but this process required elevated temperatures [2].
Containment equipment manufacturers, engineering firms, and engineering societies wanted a definitive means of describing achievement levels of the engineering control and uniform test methods to validate the level of control capability of a specific engineering control configuration. In 2001, a book entitled Containment in the Pharmaceutical Industry was published, outlining containment control strategies based on hazard bands that addressed the potential solids, liquids, and vapors have to create hazardous emissions [3].
RISK ASSESSMENT BY NIOSH AND THE EUROPEAN MEDICINES AGENCY
In August 2009, NIOSH published “Qualitative Risk Characterization and Management of Occupational Hazards: Control Banding (CB): A Literature Review and Critical Analysis” [4]. The scope of the publication includes CB strategies, presented within the context of qualitative occupational risk management concepts. The risk management strategy associated with CB is characterized by selection and implementation of appropriate control solutions.
In November 2014, the European Medicines Agency (EMA) finalized a guideline on setting exposure limits for use in risk identification in the manufacture of different medicinal products in shared facilities [5]. The guideline addresses management of cross-contamination based on risk assessment to ensure the safety of workers exposed to residual substances.
MEASUREMENT TECHNIQUES
Empirical methods are used to check the integrity of an enclosure before putting it in service. Acceptance by the quality organization is the main criterion for method selection. Test procedures include pressurized leak testing; ammonia leak testing, using ammonia-sensitive strips; aerosol fog leakage, using a particle counter; and pressurized sound sensing. Methods not involving chemicals contacting enclosure inner walls are more suitable for small enclosures with simple designs.
Quantitative methods have been established for measuring potential personnel exposures and engineering performance. The International Society for Pharmaceutical Engineering (ISPE) Good Practice Guide entitled Assessing the Particulate Containment Performance of Pharmaceutical Equipment [6] details the measurement of worker exposure within the test environment. A statistically robust engineering approach is described in Containment in the Pharmaceutical Industry [3]. The procedure can be used to create reference performance data against which further testing throughout the life of a containment device can be established (e.g., after a maintenance event).
TABLE 14.1
Hierarchy of Containment Technologies
Technology | Contamination Control Capabilitiesa |
---|---|
a Capabilities are expressed in levels in the breathing zone of a person over an 8 or 12 h exposure time frame. | |
b The use of PPE as the primary engineering control is unacceptable to either the Occupational Safety and Health Administration (OSHA) or the Canadian Centre for Occupational Health and Safety (CCOHS). | |
c Below the quantification limit of the methodology. | |
Isolators | Less than 0.1 ng/m3b (both personnel exposure and engineering capability data) |
Glove bags | Less than 0.1 ng/m3c (both personnel exposure and engineering capability data) |
Open-front, unidirectional airflow devices (includes downflow booths and biological safety cabinets) | Approximately 10 ng/m3 |
Local/point exhaust | 30–100 μg/m3 |
COMPARISON OF EXPOSURE RISK TO ISOLATION TECHNOLOGY SOLUTIONS
Exposure risks are based on a number of factors, including engineering controls, personal protective equipment (PPE), quantity of compound present, and sampling and measurement sensitivity. The basic premise of the engineering control strategy should be to contain the compound as close to the source as possible. If the process equipment is able to provide adequate containment, the challenge becomes transfers and sampling of the compound during processing and equipment servicing. These are the points where additional isolation technology is required.
Engineering controls used for containment of the exposure risk can be categorized based on the effectiveness of the control device. Banding of compounds into groups with a given level of exposure allows engineering controls to be matched up with the bands. An example of this approach is expressed in a hierarchy of the containment capabilities of given engineering control technologies (Table 14.1). It should be noted that the level of protection afforded by the engineering technology is only a guide and needs to be verified by validation of the engineering control.
TECHNOLOGY DESCRIPTIONS
Within a given containment technology, there are a number of key elements that impact performance. This section explores not only the different technologies but also the configuration of the technology that impacts the containment ability of the engineering control device.
ISOLATORS
All isolators are constructed with four basic components: the physical structure, the internal environment, interaction components, and monitoring systems. The choices selected for alternative components in each of these categories determine the overall capability of the isolator. A mock-up of the isolator is an effective means of determining functionality of the isolator and should consist of easily modified materials, such as polystyrene panels defining the boundaries of the isolator and clear polycarbonate for the window. The mock-up is likely a throwaway item, not creating a look-alike piece to the isolator, but focused on testing functionality. The bottom should be capable of supporting the intended objects that will be placed into the isolator for manipulation. The mock-up offers several other uses, including ergonomic evaluations and as a training tool.
Physical Structure
The physical structure is made up of a variety of materials broken into two basic classes: hard shell or soft shell. The selection depends on the following three issues: durability, cleanability of internal surfaces, and line-of-sight visibility of the inside of the isolator. Typical hard-shell materials are various grades of stainless steel, plastic, and polycarbonates. Durability requirements, such as the environment in which the isolator is placed, equipment to be used in the isolator, internal environment in terms of heat and chemical compatibility, and cleaning requirements, impact the type of material used to construct the physical structure of the isolator.
Internal Environment
The internal environment requirements are a function of the processes to be performed inside the isolator. Requirements include air quality in terms of particulates; incorporation of special gases, such as nitrogen or argon, for product protection; the risk of flammability or explosion; and humidity control required by the process. Passing the air entering the isolator through high-efficiency particulate air (HEPA) or ultra-low penetration air (ULPA) filters removes particulates, controlling particulate air quality requirements. Air handling systems recirculate the air through either redundant filters or a once-through system. The recirculation system is typically used if special gases are required inside the isolator or if the location of the isolator makes exhausting to the outside difficult. The once-through systems are typically used for removing heat from the isolator or reducing cycle times if a Vapor Hydrogen Peroxide (VHP) biodecontamination system is used to decontaminate aseptic operations.
Transfer Technologies
Transfer technologies are the means of introducing materials into or taking materials out of isolators and range from opening a door to access the interior of the isolator to double-door transfer systems. A single-door access offers no level of separation between the outside environment and the interior of the isolator; however, if the process does not result in any hazardous gases or particulates, it would be acceptable when all materials are placed in the isolator and the door is closed. The double-door transfer system creates a transition space, or airlock, that contains an environment that differs from the outside environment. Typical applications for this are an aseptic transfer, an inert environment, or manipulation of hazardous compounds (e.g., weighing, mixing, or product additions). ISO 14644-7, “Cleanrooms and Associated Controlled Environments (Part 7): Separative Devices (Clean Air Hoods, Gloveboxes, Isolators and Mini-Environments),” contains descriptions of a number of transfer devices [7] (Figure 14.1).
Interaction Components
Interaction technologies are the means by which personnel exterior to the isolator perform operations inside the boundary of the isolator. Table 14.2 describes types of interaction technologies and their applications to different types of isolator structures.
Robotics
The use of robotics has made advances in a number of applications. Robotics offers a consistent means of performing a repetitive task that can reduce ergonomic stress as well as personnel error. Applications include sampling and personnel assist with heavy or hard-to-reach areas in isolators (Figure 14.2).
Flexible Membranes
Flexible membranes allow enough pliability to extend manipulation through the membrane wall. This technology has very limited applications.
FIGURE 14.1 Containment weigh and mix isolator.
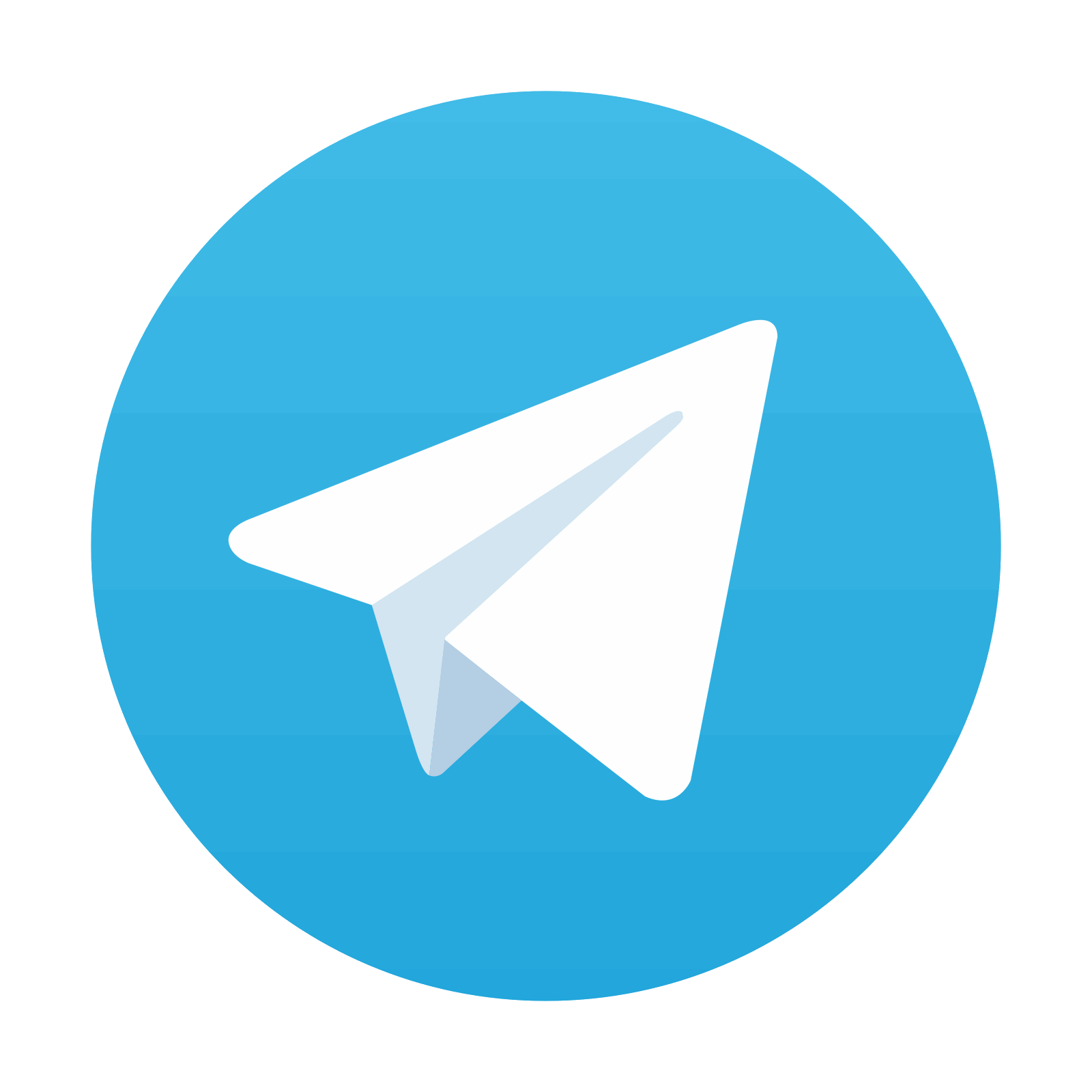
Stay updated, free articles. Join our Telegram channel

Full access? Get Clinical Tree
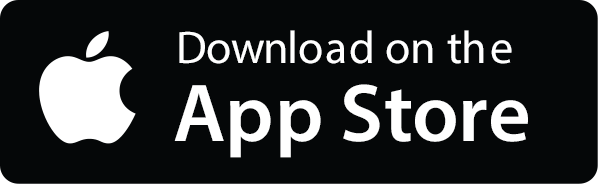
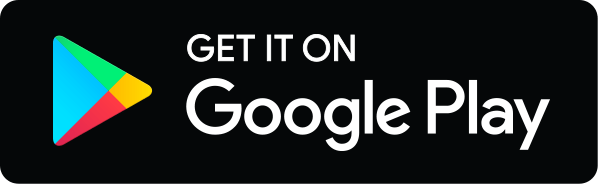