5 Bryan A. Fioret and Antonis K. Hatzopoulos Department of Medicine, Vanderbilt University, USA Adult stem cells (ASCs) are unique clusters of undifferentiated cells which reside in specialized tissue-specific niches within an adult organ. ASCs have the ability to self-renew and to differentiate into some or all of the mature cells of the specific organ in which they reside [1]. They are also known as somatic stem cells, or stem cells of the body, to distinguish them from germ cells involved in reproduction. ASCs primarily differ from their embryonic stem cell (ESC) counterparts in both their origins and their lineage differentiation capabilities. ESCs, derived from the inner cell mass (ICM) of the blastocyst, are pluripotent and capable of differentiating into the three germ layers of the embryo (endoderm, mesoderm, and ectoderm) and generating all embryonic and somatic cell types [2]. Unlike ESCs, whose origins are clearly defined, the origins of various ASC populations are still unclear. The differentiation potential of ASCs is also more limited (multipotent instead of pluripotent) and they are generally lineage-restricted to the cell types of their host organ [3]. ASCs have been found in adolescent and adult animals, in tissues such as the hair follicles of the skin, intestine, bone marrow, specific regions of the brain, skeletal muscle, liver, teeth, ovaries, and testes [4]. However, ASC populations are relatively rare. Within each of these tissues, ASCs exist in specified niches, which are local microenvironments responsible for regulating their proliferation and differentiation [1,3]. ASCs often remain within their specific niche in a quiescent state until required to proliferate. ASC populations are maintained through symmetric cell divisions, in which two identical daughter stem cells are generated. They are also capable of generating multipotent progenitors with limited self-renewal capacity through asymmetric division [3]. One of the primary characteristics of ASCs is their ability to self-renew indefinitely [3]. Consequently, they play a vital role in the maintenance of organ homeostasis by replacing dying cells lost to wear-and-tear, and also serve as a regenerative cell source after tissue damage or disease [3]. Because of their ability to self-renew and differentiate into mature cell types, ASCs are a potential resource for a wide variety of therapeutic applications. For example, various types of organ-specific ASC have been administered to animals and patients in an attempt to treat hematopoietic cancers [5], vascular disease [6], spinal cord injury [7], and cirrhosis of the liver [8]. The versatility and therapeutic potential of ASCs, as well as their need for maintenance and repair of adult tissue, warrant further study to optimize their use for treatment of tissue-specific disease or injury. The number of organs in which ASCs have been identified and characterized is continuously growing. However, numerous questions remain regarding their origins and the factors regulating their proliferation and differentiation. Consequently, significant effort has been applied toward understanding their regulatory signals and the regions in which they reside: the stem cell niche. The concept of a niche for cells was first proposed in 1978 based on studies involving hematopoietic stem cells (HSCs) [9]. Contradictory data indicated apparent limitless renewal of stem cells but a finite lifespan for spleen colony-forming cells, which were believed to be HSCs themselves [9]. To reconcile this discrepancy, the idea of a stem cell niche was proposed to explain how stem cells were regulated to continuously self-renew but also to generate differentiated progeny that do not persist indefinitely and have various rates of turnover. The stem cell niche is more fully defined as a unique, tissue-specific, regulatory microenvironment responsible for enabling and controlling stem cell self-renewal while balancing internal and external molecular signals for the maintenance or repair of host tissue [1]. An increasing number of studies have identified a wide variety of tissue-specific stem cells, each with its own unique niche [3]. Studying the relationship between these stem cells and their niche environments will provide a better understanding of maintenance of organ homeostasis, or repair of damaged tissue in the adult. The primary focus of this chapter is the study and comparison of four specific, well-characterized niches in mammalian adult organs. These niches are found within (i) the hair follicles of the skin, (ii) the crypts of the small intestine, (iii) marrow within the bone, and (iv) distinct neural regions of the brain. The role of the niche in providing structure and regulating resident stem-cell proliferation, maintenance, and differentiation through coordination of support cells and molecular signaling is also reviewed. The skin is composed of three layers: the epidermis, the dermis, and a subcutaneous fatty layer, the hypodermis. The hair follicle, the structure which produces hair, extends through each of these layers. It is part of the pilosebaceous unit, which also includes the hair shaft, sebaceous gland, and arrector pili muscle. Each hair follicle contains a bulge region in the outer root sheath, which houses quiescent follicular stem cells [10]. The hair germ (HG) lies directly below the bulge and contains another cluster of proliferating follicular stem cells. Signals from the dermal papilla (DP), which lies at the base of each hair follicle, stimulate activation of HG stem cells. Hair follicle stem cells (HFSCs) reside in the bulge region and migrate to the HG to regenerate hair and sebaceous glands [11]. HFSCs exist in a quiescent state in the bulge region and in an active state in the HG and are capable of generating all lineages of hair [10,12]. Studies of HFSCs have progressed rapidly due to their location in the anatomically distinct bulge region and their ability to be isolated and studied in transplant models [13]. Their primary role is to serve as a source of cells for hair-follicle growth during the hair cycle. The hair cycle is a three-phase process of anagen (growth), catagen (apoptosis-mediated retraction), and telogen (resting). Each hair follicle contains a permanent segment (including the bulge and sebaceous gland) and a cycling segment, which moves through the three hair-cycle phases [10,14]. Anagen begins when the DP is proximal to the bulge and HG, and stimulates HFSC differentiation from the HG through Wnt/β-catenin signaling, fibroblast growth factor 7 (FGF7), and antagonism of bone morphogenetic protein (BMP) signaling [15]. Activated HG cells enter the hair matrix that surrounds the DP, which causes activation and migration of quiescent HFSCs in the bulge to replenish the lost HG cells. TACs derived from activated HG stem cells contribute to hair growth, which pushes the DP further from the bulge HFSCs. The physical separation of these two components minimizes the continued activation of HFSCs, allowing for the transition to catagen. During this phase, apoptosis of active progenitor cells then causes the hair follicle to shrink until the DP again rests close to the bulge and HG, allowing the cycle to start anew, after the resting period of telogen. The hair follicle bulge serves as a niche for HFSCs. These cells were first hypothesized to be stem cells based on studies which identified slow-cycling cells within this anatomical compartment [16]. Labeling of HFSCs using either [3H]-thymidine or 5-bromo-2′-deoxyuridine (BrdU) demonstrated their existence as label-retaining cells (LRCs), but live cells could not be isolated. Subsequently, transgenic mice were generated with a keratin 5 (K5) promoter driving expression of a doxycycline-controlled, histone H2B-GFP fusion protein in the cells of the skin epithelium. Due to their slow cycling, bulge HFSCs retained the H2B-GFP after a 4-month chase and could be isolated [12]. The self-renewal and multipotency characteristics of HFSCs were further defined using keratin 14 (K14) promoter-driven GFP transgenic mice [17]. HFSCs isolated using GFP and their stem-cell marker CD34 generated the entire hair follicle after transplantation on to the back skins of nude mice. mRNA analysis of HFSCs (isolated LRCs) compared to their differentiated progeny revealed a number of differences, which provide insight into their niche [12]. HFSCs had greater expression (≥2×) of transcripts encoding cell-cycle regulatory proteins, including keratinocyte growth inhibitors, and downregulated levels of proliferation-related proteins (Ki67 and Cdc25C) [12]. Expression of both secretory and integral membrane proteins (β1,4,6 and α6 integrins, among others) were elevated in the HFSCs [12], including ephrin receptors and their ligands, which are involved in cell–cell communication and the formation of tissue boundaries. In addition, β6 integrin is expressed only during the early anagen growth phase and may stimulate stem cell proliferation and migration through interaction with the extracellular matrix (ECM) glycoprotein tenascin C. These findings imply a level of organization within the bulge that allows for regulation of HFSC proliferation, adhesion, and interaction with neighboring cells. The bulge HFSC niche is organized into upper and lower regions, each with a distinct subset of cells [17,10]. The stem-cell marker Leucine-rich repeat-containing GPCR5 (Lgr5) is expressed in the bulge and HG and can be used to subclassify these populations of cells. The upper bulge contains a population of CD34+, Lgr5− cells, while the lower bulge contains a CD34+, Lgr5+ cell pool. Within the HG, closer to the DP, is a population of CD34−, Lgr5+ cells. These are activated first by the DP during anagen to generate TACs and generate the hair shaft. The quiescent population of upper-bulge CD34+, Lgr5− cells is then activated to replace the HG cells. Thus, the bulge contains unique sites for maintaining quiescent and active populations of HFSCs, restricting their growth and differentiation until replenishment of cells is required. The canonical Wnt and BMP signaling pathways are crucial regulators of the bulge HFSC niche. Transgenic mice with transiently stabilized β-catenin exhibited de novo hair-follicle morphogenesis, forming newly functioning follicles with DP, sebaceous gland, outer and inner root sheath, and hair shaft [18]. The interaction of β-catenin and the Wnt-dependent transcription factor Lef-1 was strongly implicated in mediating this new growth. Furthermore, transgenic mice with enhanced β-catenin showed decreased time spent in telogen in favor of a more rapid switch to the anagen growth phase of the hair cycle. Signaling through BMP receptor 1A (BMPR-1A) is necessary for the differentiation of HG progenitor cells within the inner root sheath and hair shaft [19]. BMPR-1A null animals exhibit defects in their hair follicles. Additionally, mice unable to express the BMP inhibitor Noggin lack expression of Lef-1, indicating an intrinsic link between canonical Wnt and BMP signaling. Crosstalk between these two pathways begins with Noggin-mediated inhibition of BMP signaling, allowing for activation of Wnt/β-catenin to stimulate proliferation of HFSCs. Thus, activation of Wnt/β-catenin signaling leads to proliferation of HFSCs, while BMP signaling represses their activation through inhibition of Wnt [20]. Consequently, maintenance of quiescence in the HFSC niche of the bulge involves a “Wnt-off/BMP-on” balance of mediators, which is reversed at the DP to stimulate cell differentiation and follicle growth. Interestingly, in studies of individuals with androgenetic alopecia (AGA), or common baldness, cytokeratin15 (KRT15+) stem cells were still present in samples of bald scalp [21]. These quiescent KRT15+ cells were small in size and localized within the bulge. However, a population of larger, proliferative CD200+ CD34+ progenitors located next to the bulge was noticeably diminished. Within these bald-scalp samples, the size of the hair follicle was also significantly smaller, perhaps due to a defect in the conversion of HFSCs to their progenitors. The role of the HFSC niche as a factor in this situation cannot be understated, especially when the problem with generation of follicular progenitors may be a result of deficient signal transduction at the HFSC level. A better understanding of the molecular cues related to this process, which may be misregulated by the bulge HFSC niche, could directly benefit individuals with AGA. Digestion of food and absorption of nutrients occurs in the gastrointestinal (GI) tract, particularly the stomach and the small and large intestines. The mix of digestive enzymes from the stomach and pancreas and bile from the gallbladder creates an inhospitable environment for GI epithelial mucosa, which causes these cells to have some of the most rapid turnover rates of any organ in the body. Mature cells of the intestinal epithelium have an average lifespan of less than 1 week, and thus require a robust pool of intestinal stem cells (ISCs) for maintenance of intestinal homeostasis [22]. The ISC niche is located at the bottom of the intestinal Crypts of Lieberkühn (crypts), which are small recesses within the epithelial lining of the small intestine and colon. Both of these regions contain crypts, but only the small intestine contains villi [20,22]: epithelial protrusions from the intestinal wall composed of mature absorptive cells known as enterocytes and numerous secretory cells, including enteroendocrine, goblet, and tuft cells. Paneth cells are also secretory but remain in the crypt of the intestine to support maintenance of ISCs. The ISC niche generates all of the cells of the small intestine. It does so as they migrate upwards into the villi, maturing into the cell types needed to replace lost cells. The colon also contains an ISC niche within the crypts, but progenitor cells migrate apically into a smooth epithelium (instead of villi), which is optimized for absorbing water [22]. Two populations of ISCs exist within the crypt niche of the small intestine, both of which are multipotent and capable of self-renewal. The first, at the +4 position from the crypt base, is a quiescent population of slow-cycling LRCs [10]. This population constitutes 2–4 of 16 cells in the circumferential ring of the crypt and is marked specifically by Bmi-1, mTert, and Hopx [23,24]. The second ISC population is found at the bottom of the crypt, interspersed between supportive Paneth cells, and marked by Lgr5, CD133 (or Prom1), and Sox9 [22,25]. This fast-cycling group is also known as crypt basal columnar cells (CBCs), and like the first group is able to generate the entire crypt and villi. While the full extent of the interactions between these two ISC populations (Bmi-1+ LRCs and Lgr5+ CBCs) is still being elucidated, it is known that they participate in a bidirectional relationship. In mice, complete ablation of Lgr5+-expressing cells did not affect intestinal epithelial homeostasis [25]. In response to the loss of these cells, Bmi-1+ cell proliferation increased, and lineage-tracing studies indicate that they compensated by generating Lgr5+ cells. Conversely, in vitro and in vivo studies have demonstrated that Lgr5+ cells can generate Hopx+ cells at the +4 position of the crypt [26]. These studies also indicate that the quiescent Bmi-1+ ISC population interconverts into rapidly cycling CBCs, which further differentiate to repopulate to the villi. Conversely, the quiescent Bmi-1+ cells can be replenished from the CBCs. Thus, distinct clusters of ISCs in the crypt niche appear to interact and renew one another. Within the crypts, the ISC niche contains a number of support cells and structural components. Paneth cells are specialized daughter cells, derived from and interspersed between Lgr5+ CBCs. They secrete antimicrobial enzymes and peptides such as lysozyme and cryptins/defensins, respectively [27]. In addition to providing defense against foreign agents at the mucosal surface, CD24+ Paneth cells express epidermal growth factor (EGF), transforming growth factor alpha (TGFα), Wnt3, and Dll4, which promote proliferation and maintenance of ISCs through their respective signaling pathways [27]. Genetic removal of Paneth cells in vivo leads to the loss of Lgr5+ CBCs, indicating their important supportive role in the crypts of the small intestine. Maintaining intestinal homeostasis under a wide range of digestive conditions requires a number of signaling pathways to control cell fate and is mediated via niche support cells throughout the crypt and villus. The canonical Wnt/β-catenin pathway is crucial for maintenance and proliferation of undifferentiated ISCs [28,29]. Paneth cells release Wnt ligands, which act on the Wnt receptors Frizzled (Frz) and LRP5/6 in neighboring cells. They also produce the Wnt agonist R-spondin, which also activates Wnt through the Lgr5 and LRP5/6 receptors. In transgenic mice, inducible ablation of the β-catenin gene caused a loss of all intestinal epithelial cells within 6 days of induction, as well as a decrease in number and size of intestinal crypts [29]. Loss of β-catenin also inhibits ISC proliferation, partially through decreased expression of the Wnt/β-catenin target gene c-myc, which induces ISC proliferation, and partially through increased expression of the cell-cycle inhibitor p21 [29]. In addition, loss of Tcf7l2, the gene encoding the Wnt transcription factor Tcf-4, causes death in postnatal mice [30]. Analysis of the intestine in these mice revealed the absence of proliferative crypts between villi, the entire intestinal epithelium consisting of differentiated, nonproliferative villus cells. Conversely, young transgenic mice expressing a dominant stable mutation of β-catenin revealed excessive ISC proliferation and adenomatous polyps [31]. Furthermore, loss of adenomatous polyposis coli (APC), a critical member of the β-catenin destruction complex, induces a progenitor-like state throughout the crypts of the intestine and formation of colorectal adenomas [32,33]. These studies indicate the strong influence of canonical Wnt signaling on promoting ISC proliferation and maintenance of self-renewal. Much like canonical Wnt signaling, the Notch pathway enhances the pool of proliferative ISCs in the crypt [28]. The Notch target gene Hairy, an enhancer of split (Hes1), and the putative stem-cell marker Musashi-1 (Msi1) both mark CBCs and +4 cells within the crypt [34]. Through lineage-tracing studies of Notch signaling-positive cells, using the Notch1 Intramembrane Proteolysis (N1IP-Cre) mouse line, Notch signaling was found to be active in ISCs [35]. Cell-fate studies in mice have also demonstrated that Notch1 and -2 receptors are specifically expressed in crypt stem cells but do not mark supportive Paneth cells [36]. Transgenic mice lacking the Notch-dependent transcription factor CSL display an expanded number of secretory goblet cells and less proliferative ISCs [22]. The same effect is observed after antibody blockade of the Notch1 receptor [37]. Furthermore, mice expressing a constitutively active Notch1 receptor (via the Notch intracellular domain, NICD) contain villi populated with immature progenitor cells and lack a differentiated epithelium [36]. These studies strongly indicate the role of Notch signaling in maintaining ISC proliferation and inhibiting differentiation to secretory cells. This maintenance of stemness via the Notch pathway is partially achieved in Lgr5+ CBCs through interaction with the Notch ligand Dll4 from supportive Paneth cells [27]. In addition to active Wnt and Notch signaling, the BMP pathway is active in the epithelium and mesenchyme of the small intestine [28]. Mouse models overexpressing the BMP inhibitor Noggin display ectopic crypts and have intraepithelial neoplasia commonly associated with polyposis [38]. A similar phenotype is observed in transgenic mice lacking the BMPR-1A (Alk3), which exhibit hyperproliferation of ISCs [39]. Thus, BMP signaling represses proliferation of ISCs, which reduces the number of aberrant crypts and polyp formation in the intestine. Suppression of ISC self-renewal via intestinal BMP signaling alternatively promotes ISC differentiation into secretory enteroendocrine cells. BMP signaling also regulates the maturation of differentiated ISC progeny by counteracting the proliferative effects of the Wnt/β-catenin pathway. BMP signaling activates the phosphatase PTEN, which then blocks AKT to inhibit activation of β-catenin [20]. This BMP-mediated suppression of canonical Wnt balances ISC self-renewal with differentiation, since both processes are equally necessary for intestinal homeostasis. Isolated Lgr5+ CBCs have been successfully used to generate crypt–villus “minigut” structures in vitro [40]. Single Lgr5+ stem cells, isolated from the intestines of Lgr5-EGFP-ires-CreERT2 transgenic mice, were isolated based on expression of GFP and cultured in conditions promoting growth of intestinal epithelium. These conditions involved treatment with EGF, Noggin, and the Wnt agonist R-spondin to imitate the proliferative crypt environment. After 2 weeks of treatment, proliferative intestinal-like organoids formed from individual Lgr5+ GFP+
Comparative Analysis of Adult Stem Cell Niches
5.1 Adult Stem Cells
5.2 Adult Stem Cell Niches
5.3 The Hair Follicle Stem Cell (HFSC) Niche
5.4 The Intestinal Stem Cell (ISC) Niche
Stay updated, free articles. Join our Telegram channel

Full access? Get Clinical Tree
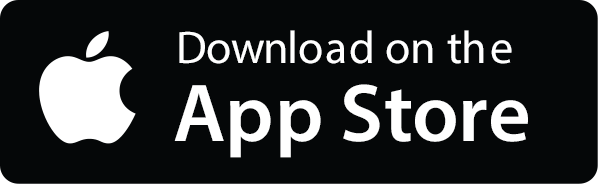
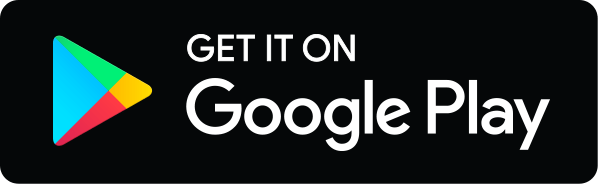