Vaccines
Vaccine type
Serum IgG
Mucosal IgG
Mucosal IgA
T cells
Hepatitis A
Killed
++
Japanese encephalitis
Killed
++
Polio Salk
Killed
++
+
Rabies
Killed
++
Influenza
Killed, subunit
++
(+)
Hepatitis B (HBsAg)
Protein
++
Papillomavirus (human)
VLPs
++
++
Rotavirus
VLPs
(+)
(+)
++
In retrospect, the historical approach to viral vaccine development succeeded against viruses that exhibit minimal antigenic variation (antigenic variation may be conceptualized as the variation in reactivity of the virus with human antibodies). By contrast, HIV may be the most antigenically variable pathogenic virus known. The first randomized placebo-controlled phase III clinical trial of the traditional vaccine strategy, consisting of immunization with repeated boosting injections of recombinant HIV surface envelope proteins, took place in the 1990s. The trial was known as the VaxGen trial, and the vaccine was trademarked as AIDSVAX®. This vaccine successfully elicited autologous-strain-specific, anti-HIV neutralizing antibodies but failed to protect human subjects from HIV acquisition.
One conclusion that became better appreciated as a result of the VaxGen trial was that the degree of antigenic variation exhibited by circulating HIV strains was too great to overcome with existing vaccine technology, namely, that immunization could protect only against the strains comprising the vaccine, but vaccinated individuals were very unlikely to encounter those strains among the thousands circulating in the real world. Accordingly, a paradigm shift took hold in the HIV vaccine field: some thought leaders advocated acknowledging the inability of antibodies elicited by vaccination to protect against HIV infection and instead supported developing methods to stimulate cell-mediated immunity against the virus in the hope of clearing the virus successfully upon encounter. Cell-mediated immunity stimulation is generally achieved through delivery of an intact, functional, but attenuated and engineered viral vector, such as adenovirus.
The known efficacy of licensed live, attenuated viral vaccines, e.g., against polio, seemed consistent with this approach. Promising preclinical data on an adenovirus construct advanced this alternative approach toward a second randomized placebo-controlled phase III HIV vaccine clinical trial. Unfortunately, this approach, too, failed and, worse, appeared to have increased infection risk for certain subsets of the vaccinated subjects (STEP study) (Kim et al. 2010). The failure of both the traditional and the major alternative approach to HIV vaccination left the field at a loss over the first decade of the twenty-first century. Fortunately, a third randomized placebo-controlled phase III clinical trial, the RV144 trial, had already started and finally resulted in the observation, in 2009, of statistically significant protection from HIV acquisition by a vaccine (Rerks-Ngarm et al. 2009). Interestingly, the RV144 trial was formulated as a hybrid of the two prior failed approaches, consisting of both a viral vector prime intended to stimulate cell-mediated immunity and the same AIDSVAX® protein immunogen boosts from the VaxGen trial. This recipe was highly controversial, if simply from a “two-wrongs-don’t-make-a-right” point-of-view, to the point that many major thought leaders in the field objected to the trial in a letter published in Science (Burton et al. 2004).
Ironically, this “flawed” recipe turned out to be the only one that has ever resulted in any evidence that human subjects can be protected from HIV acquisition by vaccination (Haynes et al. 2012). In this study, HIV infection was reduced by approximately 30 %. Although RV144 provided the first evidence of HIV vaccine efficacy, the effect was not strong enough to justify development of its ALVAC canarypox prime-AIDSVAX recombinant protein boost vaccine into a commercial product. Unfortunately, the protective effect was transient, disappearing after 6 months in the study population. Sub-analyses suggest that the antibody response did not endure (Haynes et al. 2012).
6.4 Human Immune Correlates of Protection in HIV
The RV144 case-control study was specifically designed to correlate protection from HIV infection with vaccine-induced immune factors (Haynes et al. 2012). More than 270 assays were performed for the RV144 immune correlates case-control study. Of these 270 measurements of host immune molecular function, only three were associated with an odds ratio (OR) of less than 0.6 of being infected with HIV. Thus, only these three detected a host molecular entity, high levels of which were significantly associated with low levels of infection. The common viral antigen used in all three assays was a short peptide fragment from position 166 to 179 of the V2 loop of HIV’s surface envelope glycoprotein (V2166–179; Fig. 6.1).
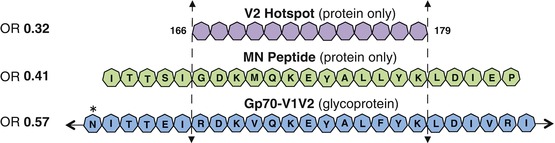
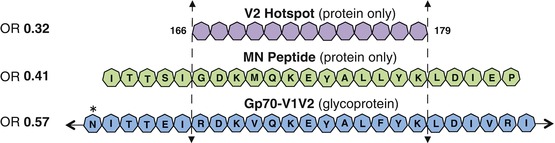
Fig. 6.1
Protection maps to V2166–179. Of the approximate 270 assays performed in the RV144 immune correlates analysis, only Abs binding three reagents showed an odds ratio (OR) of 0.57 or lower (Haynes et al. 2012) (OR shown at the left of the above figure). These reagents were the gp70-V1V2 fusion glycoprotein (sequence for a portion of the V1V2 domain of gp70 shown in blue heptagons with glycosylation sites indicated by an asterisk), the MN peptide (sequence shown as green heptagons from positions 161–183), and the V2 Hotspot (shown as purple heptagons spanning positions 166–179). All three of these reagents include an unglycosylated portion of the V2 domain spanning positions 166–179 (Adapted from Tassaneetrithep et al. PLoS One. 2013; Creative Commons Attribution)
The superset of the viral antigens of all three assays was the entire V1V2 glycoprotein domain of HIV’s surface envelope glycoprotein (two of the viral antigens were fragments of the largest one; see Fig. 6.1). These three viral antigens detected vaccine-elicited host antibodies that DIRECTLY led to protection (the low OR suggests that these antibodies directly blocked infecting viruses) (Haynes et al. 2012). These are the only three assays to detect such molecules, as the only other protection-associated OR was a significantly high OR for plasma IgA, which can only be explained by indirect mechanisms (Haynes et al. 2012). The protection observed in the RV144 trial did not correlate with broadly neutralizing antibody levels measured in vaccinated subjects. Thus, anti-V1V2 Abs, and perhaps only anti-V2166–179 human antibodies, are the only molecular entities with direct evidence of association with protection from HIV infection. Interestingly, protection against neutralization-resistant viruses was also recently correlated with anti-V2 Abs in a nonhuman primate (NHP) model (Barouch et al. 2012).
A sieve analysis of the RV144 data independently found only two amino acid positions in the HIV genome that were statistically associated with vaccine efficacy and both positions are within or near the same common region identified in Fig. 6.1 (position 169 and position 181) (Rolland et al. 2012). Furthermore, a plot of the OR of a series of V1V2 domain antigens tested in the RV144 immune correlates analysis containing the 169–179 region of HIV’s V2 loop shows that protection only appears when the antibodies in vaccinated subjects, which were likely elicited from a subtype AE HIV strain, cross-react with strains from subtype B (Fig. 6.2). These are the two most evolutionarily distant HIV subtypes among circulating strains, suggesting that the protective antibodies may be targeting accessible, functionally important epitopes in the virus that are conserved across most circulating strains. This observation extracted directly from the RV144 case-control analysis of human subjects has been partly confirmed by an independent, follow-up study (Zolla-Pazner et al. 2014). Thus, integration of all the detailed protection data from the RV144 trial points to Abs elicited by the ALVAC-AIDSVAX vaccine targeting epitope(s) composed of amino acids located approximately in V2165–179 and conserved across circulating HIV strains as being the most likely molecular entities associated with protection from HIV acquisition in human subjects. While these may not be the only epitopes responsible for the protection observed in the RV144 trial, these are the only ones for which such specific molecular detail can be inferred directly and exclusively from the primary RV144 trial human subject data. Most importantly, for the first time, an approximate, but very specific, location and composition of antibody-targeted epitopes on HIV-1’s viral envelope have been associated with protection from HIV acquisition in human subjects.
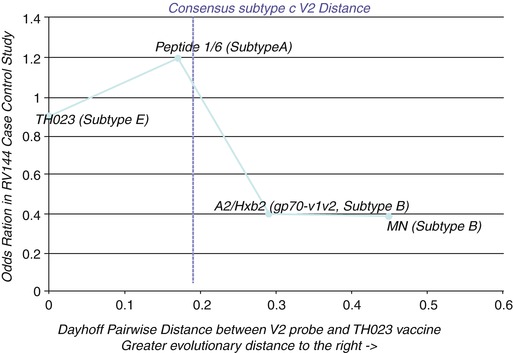
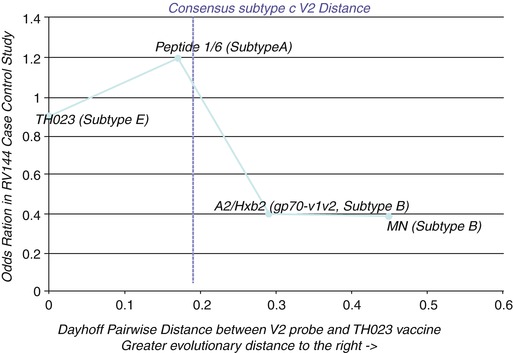
Fig. 6.2
Protective Abs need to be elicited by the subtype AE strain TH023 and yet cross-react with subtype B strains. Plotting the OR (Y-axis) of diverse V2 loop peptides tested in the RV144 case-control study against the evolutionary distance of each peptide from the TH023 immunogen (X-axis) shows that protection (low OR) only appears at the distance of subtype B. Thus, only those Abs elicited from subtype AE but cross-reacting with evolutionary distant subtype B strains were associated with protection. Abs that cross-reacted with less distant heterologous viruses (e.g., subtype A) were not associated with protection
6.5 Molecular Opportunity of a Combined Cocaine and HIV Immunogen
The above recent history of the parallel cocaine and HIV vaccine immunogen development efforts shows some striking parallels.
1.
Both efforts have resulted at the present time in the identification of defined immunogens that have demonstrated partial efficacy of around 30 % in human subjects. In both cases, these starting points are promising, but not sufficient for product development on their own merits.
2.
Both immunogens are highly “epitope focused,” with epitope in this sense referring to antibody-targeted epitopes (as opposed to T-cell-targeted epitopes). This means that protective antibodies need to target a small, highly specific molecular shape. For the cocaine immunogen, cocaine itself is the epitope, as are all haptens that are successfully immunogenic when conjugated to carrier proteins. For the HIV vaccine, although the immunogen used in the RV144 trial was not epitope focused by design, the immune correlates analysis clearly suggests that at least one very specific epitope was responsible for protection.
Accordingly, from this starting point, one can envision a combined cocaine and HIV vaccine, provided that its nature is to be “epitope focused” or preferentially exhibiting the specific protective epitopes in a highly immunogenic configuration. For the purposes of the following discussion, we will refer to the cocaine hapten-protein conjugate as “epitope focused.”
6.6 Concept of Epitope Focusing
The term epitope is used interchangeably in the scientific literature to refer to a single molecular entity at different spatial scales. An antibody-targeted epitope is defined as a three-dimensional chemical surface and shape bound by the antigen-combining site of an antibody. Historically, antibodies have been proven to be highly specific, so one can infer that the shape and chemistry of the epitope are also highly unique among the nearly infinite variety of such shapes and chemistries in biology. Nevertheless, many years of immunologic measurements were made with antibodies and peptide antigens before the availability of atomic detail structural information on these complexes.
The term “antibody-targeted epitope” in the literature, when it refers to peptide epitopes, has been equated to a short peptide segment within a protein (also termed a “linear epitope”) or an entire protein domain. Similarly, the antibodies in question often referred to a polyclonal collection of monoclonal antibodies that targeted these segments or domains. Now that crystallographic structures of monoclonal antibodies bound to their cognate antigens are available, it is clear that individual antibody-targeted epitopes are best defined by the amino acids seen to be contacting a monoclonal antibody in a crystallographic complex, be they continuous or discontinuous in the sequence of the protein antigen (Fig. 6.3).
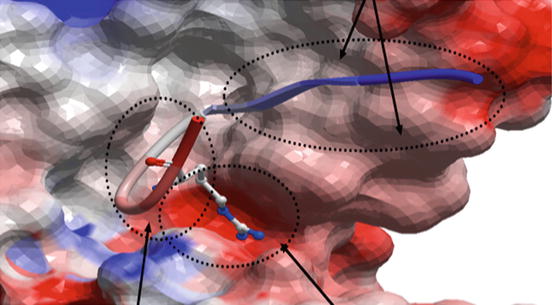
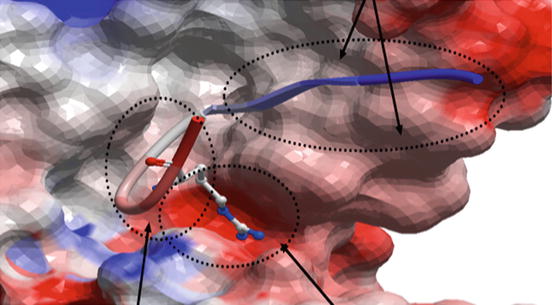
Fig. 6.3
The real definition of an antibody-targeted epitope: the molecular surface of the anti-HIV monoclonal 447-52D (PDB 1Q1J) is shown as a geometric object colored by electrostatics (red negative charge, blue positive). The backbone of the bound HIV antigen is shown in ribbon diagram with the single most strongly bound side chain shown in stick diagram. The amino acids in the circles all make contact with the antibody and thus, only their contacting atoms, in aggregate, form a chemical entity that is the epitope targeted by this antibody. This entity is poorly represented by any amino acid sequence because only a few atoms from any of the participating amino acids may actually make contact with the antibody while many noncontacting atoms enable or restrict the contacting atoms by van der Waals interactions, steric hindrance, or electrostatic interactions
For the purposes of this discussion, a single antibody-targeted epitope in HIV’s surface envelope glycoprotein is a single unique defined chemical entity decorating the surface of this protein, no different from cocaine decorating the surface of its carrier protein as a hapten conjugate. Protein chemistry is sufficiently robust that epitopes targeted by one monoclonal antibody can be directly adjacent to and actually overlapping that targeted by a different monoclonal antibody (from a different B cell and with a different amino acid sequence), yet be unrecognized by the other monoclonal antibody (Fig. 6.4). Accordingly, one can strictly theorize that a clonal antibody response can be elicited by immunization with an immunogen that presents only the unique chemical entity representing an individual epitope. In practice, eliciting by immunization a functional polyclonal response narrowly focused on individual viral epitopes has been achieved (Correia et al. 2014), but only in one report for an HIV epitope (Cardozo et al. 2014).
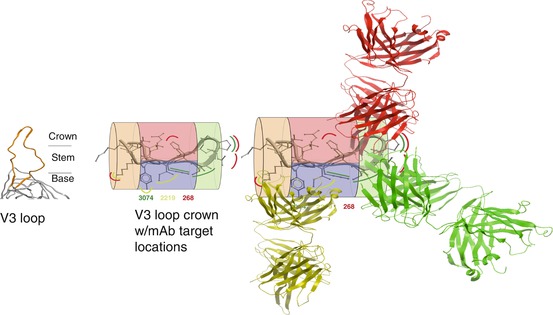
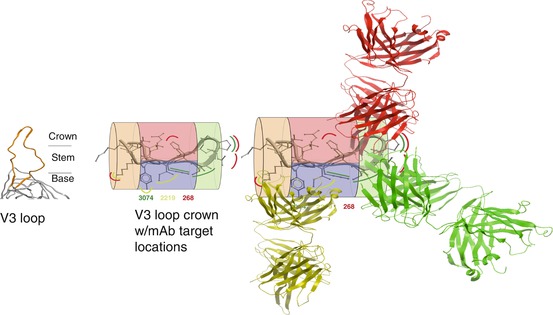
Fig. 6.4
Epitopes are often closely adjacent and overlapping discrete 3D shapes in a short peptide segment. Left: HIV’s V3 loop (orange) emerging from gp120 (gray). Middle: locations on V3 crown of monoclonal antibody (mAb)-targeted epitopes (curved lines: mAb 268 = red, mAb 3074 = green, mAb 2219 = yellow). The V3 crown is divided into four zones: stem, orange; turn, green; and two faces of ß-sheet, red and blue to assist depiction of the three-dimensional space. Right: mAb structures are depicted to illustrate distribution of their epitopes around the V3 crown (Adapted from Zolla-Pazner and Cardozo (2010))
Epitope-focusing technology may be a particularly acute need for HIV vaccine research. HIV protein immunogens designed to elicit broadly neutralizing antibodies (bNAbs) have failed to do so. These immunogens have often been variations of modifications of natural gp120 sequences. A big reason for the failure may be that HIV is highly antigenically variable and all naturally occurring gp120 sequences have evolved to display an abundance of immunodominant “decoy” epitopes that are type specific or elicit Abs that are otherwise advantageous to viral fitness (Tobin et al. 2008). Nevertheless, hidden among these decoy epitopes are epitopes that are true sites of vulnerability for the virus and that are conserved across strains, for example, those epitopes that are a structural component of host receptor binding sites or the epitopes identified in the RV144 trial.
Epitope focusing seeks to isolate these vulnerable epitopes and present them exclusively or preferentially on a non-HIV scaffold to form an epitope-focused immunogen (Fig. 6.5). The advantage of this approach is the ability to concentrate and potentially amplify the antibody response to known protective epitopes. Since, as previously discussed, specific peptide regions associated with protection in human subjects have been strongly implicated by RV144 clinical trial data, epitope focusing to these regions can be theorized as a straightforward method to optimize the existing RV144 vaccine.
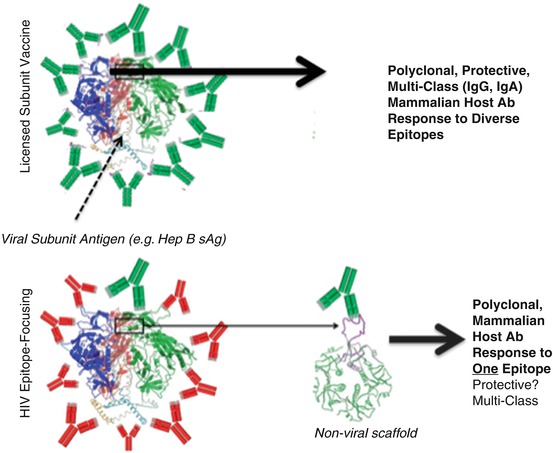
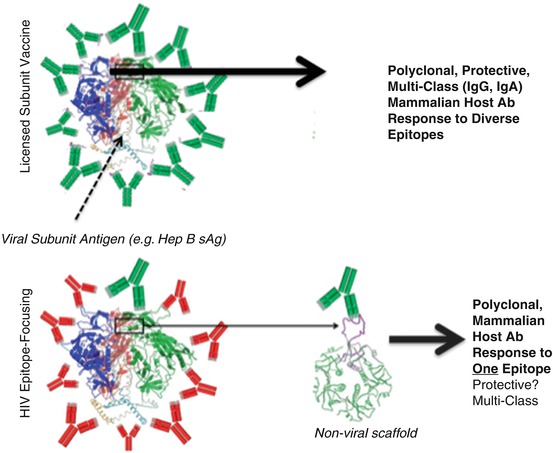
Fig. 6.5
How epitope vaccines differ from current viral vaccines. Upper panel: Currently licensed viral subunit vaccines are illustrated in the upper panel, wherein the major viral antigen is used to immunize subjects, resulting in protective IgG +/− IgA Abs (green Ab cartoons) elicited to many different sites on the antigen. Lower panel: Immunization with HIV’s gp120 antigen results almost entirely in the elicitation of Abs that do not protect against acquisition of circulating HIV viruses (red Ab cartoons). Nevertheless, the RV144 trial demonstrated that rare Abs to specific epitopes are hidden in this response that can protect against HIV acquisition. Epitope focusing seeks to “splice” out the epitopes targeted by these useful Abs and present them on non-HIV scaffolds
6.7 Cholera Toxin B: The Common Scaffold
Interestingly, the only cocaine immunogen tested in a randomized placebo-controlled clinical trial (Shen et al. 2012) and the only epitope-focused HIV immunogen to successfully elicit functional, broadly cross-strain anti-HIV antibodies (Cardozo et al. 2014) both used the identical protein scaffold to present their epitopes: cholera toxin B. Cholera toxin B (CTB) is the inert mucosa ganglioside binding subunit of the cholera toxin, so it is not toxic by itself but elicits a protective serum and mucosal anti-cholera toxin response upon immunization. It is highly immunogenic and elicits high antibody titers. Furthermore, each CTB gene product forms a pentamer, so its antigenicity is multivalent.
It is the central immunogen in a licensed cholera vaccine that has been widely and successfully used with an excellent safety record in humans. These properties of CTB make it an attractive carrier protein for vaccine investigations of cocaine and HIV at the discovery level. Notably, the cocaine vaccine showed no safety issues in humans, and the HIV construct differs from the licensed, safe-in-humans CTB protein by a short (<30 amino acid) peptide insert, so the safety concerns in humans of currently envisioned forms of CTB-based epitope-focused HIV and cocaine immunogens are very likely to be minimal.
6.8 The Concept of a Combined Cocaine and HIV Vaccine
Neither of the biologically active, specific anti-cocaine and anti-HIV immunogens is sufficiently protective in human subjects at the present time to be developed into a licensed product. Nevertheless, the existence of these two medicinal prototypes raises the theoretical possibility of a vaccine or immunotherapy available in the near future that can simultaneously raise anti-cocaine and anti-HIV Abs. Such a medicinal intervention has no precedent.
For the purposes of this text, we clarify the difference between the term “vaccine” and “immunotherapy.” An immunogen that raises Abs against antigens to which the individual has never been exposed is, in traditional terms, a vaccine. Since the desired activity of anti-cocaine Abs is more commonly envisioned to take place in individuals who have previously been exposed to cocaine, the cocaine immunogens are technically a form of immunotherapy while their use in naïve individuals constitutes vaccination. The above-envisioned HIV immunogen is a true vaccine. HIV immunotherapy is potentially feasible, including via the administration of broadly neutralizing anti-HIV monoclonal antibodies to HIV-positive individuals.
6.9 Combination Vaccination/Vaccine Coadministration
A combination vaccine consists of two or more different vaccines that have been combined into a single shot. Combination vaccines have been in use in the United States since the mid-1940s. Examples of combination vaccines in current use target DTaP (diphtheria-tetanus-pertussis) (Papaevangelou 1998), trivalent IPV (three strains of inactivated polio vaccine) (Faden et al. 1993), MMR (measles-mumps-rubella) (Bart et al. 1986), and DTaP-Hib (Fritzell and Plotkin 1992). The combination vaccine concept has been so successful clinically that greater combinations of immunogens (DTaP-Hep B-IPV-Hib) in a single vaccination have been introduced (Esposito et al. 2014). Simultaneous vaccination, or coadministration, is when more than one vaccine shot is administered during the same doctor’s visit, usually in separate limbs (e.g., one in each arm). An example of simultaneous vaccination might be administering DTaP in one arm or leg and IPV in another arm or leg during the same visit. Studies with the recently licensed HPV vaccine showed that coadministration did not reduce efficacy or safety of the individual vaccines (Noronha et al. 2014), although deleterious vaccine interactions have been theorized infrequently (Dagan et al. 2008).
Giving an at-risk individual several vaccinations during the same visit offers a practical advantage, and a combination vaccine promises even more benefit. Penetrance of treatment programs, treatment adherence, and use of public health resources for health maintenance and disease prevention is poor for adults in many of the communities afflicted by concurrent cocaine use and HIV infection. Coadministration of a cocaine-HIV vaccine could maximize compliance and afford protection more quickly than sequentially delivered vaccines. Given the history of safety and efficacy of coadministration of vaccines, this is a desirable aspect of the combined cocaine and HIV vaccine strategy. Furthermore, a combination vaccine affords the minor pain reduction advantage of a single injection instead of two. Nevertheless, a critical subject for future research is to confirm that a combined or coadministered cocaine and HIV vaccine are safe and effective compared to the coadministered version and with other commonly administered vaccines. This may be an especially interesting question if the first versions of cocaine and HIV vaccines are only partially effective.
6.10 HIV Prevention
HIV prevention methods are existing alternatives to vaccines. Although they present implementation difficulties compared to vaccines, these interventions are clinically proven, so they must be taken into account in theorizing about the impact of a combined cocaine-HIV vaccine (WHO Guidelines Approved by the Guidelines Review Committee 2014). Syringe exchange is highly effective at preventing HIV transmission among PWIDs (Abdul-Quader et al. 2013). Preexposure prophylaxis (PreP) is defined as the use of highly active antiretroviral therapy (HAART) prior to HIV exposure in HIV-negative subjects who are at high risk for exposure. The O52 study showed that PreP was over 95 % effective in preventing HIV acquisition in discordant couples. Other PrEP trials were much less effective, but theoretically, the effectiveness of such an approach could be very high for a small defineable group of individuals at risk in the population (reviewed in Wilton et al. (2015)). Circumcision also appears to be highly effective in preventing HIV transmission in non-US populations, where circumcision rates are low (reviewed in Tobian et al. (2014)).
6.11 Alternative Promising Approaches Lacking Primary Evidence in Humans
The only biologic medicinal interventions supported by activity observed in randomized placebo-controlled phase III clinical trials (TA-CD for cocaine and RV144 for HIV) are for a CTB-cocaine conjugate immunogen and a V2 loop-based epitope-focused HIV vaccine immunogen. While these are the only truly clinical-evidence-based biologics that can be inferred at the present time, technologies in both cocaine and HIV immunotherapies have continued to advance in the years since these trials were commenced.
The advances in the area of cocaine immunogens are reviewed in a previous chapter. Briefly, newer generations of cocaine hapten moieties with improved immunogenic and cross-reactive properties have been produced and studied. Similarly, alternative delivery approaches, including viral vector-based delivery of cocaine conjugates, cocaine-hydrolyzing enzymes, and anti-cocaine monoclonal antibodies, have been reported.
Similarly, in the HIV vaccine arena, a large number of broadly neutralizing monoclonal antibodies (bNAbs) have been isolated from HIV-positive individuals and characterized in detail in the past few years. Some of these antibodies neutralize more than 80 % of circulating viruses and antibodies targeting CD4 neutralize close to 100 % of viruses in vitro (Pace et al. 2013), so theoretically, if they can be elicited by vaccination, these monoclonal antibodies, or their polyclonal analogs, could be very promising. Unfortunately, only one functional (e.g., neutralizing) polyclonal antibody response in a mammal has ever been rationally elicited that specifically recapitulated the breadth and specific activity of an individual monoclonal anti-HIV neutralizing antibody (Cardozo et al. 2014), and this was an anti-V3 loop antibody targeting a very different epitope chemical entity than those targeted by the bNAbs.
Protection in RV144 did not correlate with antibody-mediated neutralization activity, so it is unlikely that any neutralizing antibody with significant breadth of specificity was responsible for the only known HIV protective effect observed in human subjects. Nevertheless, as an exploratory approach, much work has been reported on strategies to elicit such antibodies, including, as with the cocaine area, the use of viral vectors as immunogens, and to directly express the bNAbs in a sustained fashion rather than elicit them. Indeed, this latter approach has recently led to its logical conclusion that immunogenic viral epitopes need not be targeted at all: a CD4 mimic that neutralizes 100 % of viral isolates can instead be expressed in a sustained fashion using an adenovirus-associated virus vector (Gardner et al. 2015).
DNA vaccine approaches also offer a similar opportunity to viral vector approaches, as many aspects are conceptually parallel (Mossman et al. 1999; Haigwood et al. 1999). All viral vector/DNA approaches, although promising in their exploration beyond the constraints of natural antibody elicitation via immunization, in turn, however, suffer from the poor historical track record of such gene therapy-related approaches in human subjects, including the STEP study itself that previously failed unexpectedly (Kim et al. 2010).
6.12 Molecular Interactions in Common to HIV and Cocaine Use
Understanding common molecular targets affected by HIV infection and cocaine use may provide some further insight into the theoretical impact of a combination vaccine in specific US populations. Generally, HIV-1 cell entry is mediated by gp120 interaction with its receptor CD4 and its coreceptors CCR5 and CXCR4. Cocaine acts on cells via its effect on the dopamine transporter and σ-1receptor. In vivo evaluation of the interaction between HIV and cocaine was performed in huPBL-SCID mouse. This mouse model was initially developed by Mosier et al. to assess HIV pathogenesis in vivo. This is a hybrid human-mouse model in which human PBL are implanted into the peritoneal cavity of SCID mice (Mosier et al. 1991). Roth et al. have demonstrated that concurrent administration of cocaine and HIV results in a high rate of HIV-infected huPBL (Roth et al. 2002). This group also showed that in vitro exposure of PBL to cocaine increased expression of chemokine receptors CCR5 and CXCR4. Expression of CCR5 was also shown to increase in vivo preceding the boost in viral infection. Furthermore, a selective σ-1 antagonist, BD1047, blocked the effects of cocaine on HIV replication in the huPBL-SCID mouse, suggesting the role of σ-1 receptors in the modulation of the expression of HIV coreceptors (Roth et al. 2005).
In fact, σ-1R was shown to be one of cocaine’s main molecular targets (Matsumoto et al. 2002). σ-1R is a receptor chaperone at the mitochondrion-associated endoplasmic reticulum membrane that plays an important role in the ER-mitochondrial Ca2+ signaling and in cell survival (Hayashi and Su 2007). Cocaine upregulates the expression of σ-1R (Liu et al. 2005) and induces its translocation to the plasma membrane (Yao et al. 2010) and cocaine is able to bind σ-1R (Sharkey et al. 1988). In microglia, binding of cocaine to the translocated σ-1R leads to the activation of signaling cascade resulting in the induction of MCP-1 expression. MCP-1 in turn results in enhanced transmigration of monocytes leading to neuroinflammation (Yao et al. 2010). This could be one of the molecular mechanisms that explain how cocaine may increase susceptibility of HIV-infected individuals to HIV-1-associated neurocognitive disorder (HAND).
Additionally, this effect of cocaine can be mediated through its direct action on brain microvasculature. This is achieved via cocaine induction of the expression of cytokines (Yao et al. 2010), adhesion molecules (Yao et al. 2011a), and PDGF (Yao et al. 2011b). Thus, cocaine increases molecular permeability of the blood-brain barrier and HIV viral invasion by the induction of cytokines IL-8, interferon-inducible protein-10, MIP-1alpha, MCP-1, and TNF-alpha (Zhang et al. 1998). Cocaine was also shown to promote the induction of the adhesion molecules ALCAM, VCAM, ICAM, and E-selectin in both in vitro and in vivo experiments, and at least in case of ALCAM, it was mediated by the activation of σ-1R (Yao et al. 2011a; Gan et al. 1999). Cocaine induces PDGF-BB in human brain microvascular endothelial cells through the binding to σ receptor and activation of downstream signaling pathways (Yao et al. 2011b). The effect of cocaine on permeability of the blood-brain barrier is abrogated in vivo by PDGF-BB neutralizing antibody (Yao et al. 2011b).
Another important player in cocaine/HIV-1 interaction is the NMDA receptor. HIV-1 gp120 and Tat induce NMDAR-dependent neuronal death (Pattarini et al. 1998; Haughey et al. 2001). The mechanism of this phenomenon is not well understood. HIV infection results in release of excess glutamate by glia resulting in excitotoxicity via activation of glutamate receptors. NMDA receptor antagonists prevent gp120 neurotoxicity in vitro (Lipton et al. 1991). It was shown that gp120 directly binds to mouse NMDAR heterodimer subunits NR1/NR2A and NR1/NR2B (Xin et al. 1999) and that Tat binds to NR1/NR2A (Li et al. 2008). Moreover, gp120, through release of IL-1, and Tat, by recruitment of active src, increase tyrosine phosphorylation of NMDARs, which result in overactivation of the receptors by increasing delivery and potentiation of NMDAR currents (Haughey et al. 2001; Viviani et al. 2006; King et al. 2010). In addition, gp 120-activated glia release cytokines and other mediators, which may inhibit the reuptake of glutamate and induce its release (Barbour et al. 1989; Vesce et al. 1997). As such, NMDAR mediates damaging effects of HIV in the brain. Similarly, NMDAR is a target of cocaine.
Cocaine has complex influence on NMDARs in brain regions involved in reward circuitry (Ortinski 2014). Cocaine alters subunit composition of NMDARs and their synaptic distribution. NMDA receptor function can also be affected by D1 dopamine receptors. Interestingly, it was shown that σ-1R forms heteromers with D1 dopamine receptor (Navarro et al. 2010). As the effect of cocaine is mediated by the dopamine system, this interaction may explain cocaine action on NMDARs. Therefore, NMDAR is a common target of HIV-1 and cocaine and, thus, it could be the intersection point where cocaine and HIV-1 may exert their reciprocal effect on HIV progression and cocaine addiction.
Taken together, cocaine promotes HIV-1 infection and replication by upregulating HIV-1 coreceptors and inducing cytokine production. Via its effect on brain microvasculature and NMDAR, cocaine enhances neurotoxicity, therefore exacerbating HAND. In light of the described molecular interactions between HIV-1 and cocaine, the impact of a combined cocaine-HIV vaccine or immunotherapies on cocaine- and HIV-associated phenotypes has the promise of synergy at the molecular level.
6.13 Cocaine, High-Risk Sexual Behavior, and HIV
Cocaine produces short-lived, intense euphoric effects in human subjects along with an immediate profound craving for more (Breiter et al. 1997). Human neuroimaging and rodent neurobehavioral studies point to a shared neural substrate for sexual arousal and orgasm and cocaine’s motivating and rewarding effects (Carboni et al. 1989; Childress et al. 2008; Frascella et al. 2010; Meisel et al. 1993; Pfaus et al. 1990). Further, the same molecular and morphological changes in brain reward circuitry that mediate transition from psychostimulant use to addiction are induced by certain patterns of “excessive” sexual behavior (Hedges et al. 2010; Meisel and Mullins 2006; Nestler 2008). It is therefore unsurprising that behavioral cross-sensitization between repeated psychostimulant exposure and sexual behavior has been demonstrated in animal models (Levens and Akins 2004; Fiorino and Phillips 1999; Bradley and Meisel 2001).
Although the determinants of human behavior are more complex than those isolated in controlled animal studies, the basic science findings suggest a neurobiological basis for the relationship between cocaine abuse and sexual promiscuity. It is not uncommon for individuals to use cocaine strategically to facilitate sexual activity (Volkow et al. 2007), and stimulant-induced increase in sexual desire has been identified as a potential contributing factor to HIV transmission (Volkow et al. 2007). In general, cocaine use is strongly associated with high-risk sexual behavior (Kopetz et al. 2014; Lejuez et al. 2005), and crack cocaine appears to pose a unique risk. Increasing rates of HIV infection are consistently associated with crack use (Marx et al. 1991) and individuals who continue to use crack after HIV diagnosis report a high prevalence of unprotected sex, multiple partners, and exchanging sex for drugs or money (Harzke et al. 2009). An exacerbating factor in this destructive spiral is prefrontal cortical dysfunction in cocaine users, leading to a loss of self-control (Garavan et al. 2008). Moreover, recent evidence suggests that prefrontal function may be further degraded by interactive neurotoxic effects of cocaine and HIV (Meyer et al. 2014).
6.14 The Impact of a Combination Medicinal Intervention for Cocaine and HIV
With a molecular picture of a combination cocaine-HIV vaccine, HIV prevention and treatment, and the pathophysiological overlap between HIV and cocaine in mind, we now turn our attention to the impact of these molecular and/or pharmacologic elements on cocaine use and HIV infection in real populations. Theoretically, in populations affected by both HIV and cocaine use, a combination cocaine-HIV vaccine could synergize to profoundly lower HIV acquisition rates, even if each component of the vaccine were only partly effective as the current prototypes. The reality of HIV transmission networks might predict a very different outcome, however.
One target population might be amenable to a combination cocaine and HIV vaccine while another is more suitable for cocaine and HIV immunotherapies. A true combination vaccine would only physiologically make sense for administration to HIV-negative individuals who have never used cocaine. For HIV-positive individuals who have never used cocaine, the physiological parallel to a combination vaccine at this point in time, if any, would be cocaine vaccine administration in combination with proven HIV prevention measures.
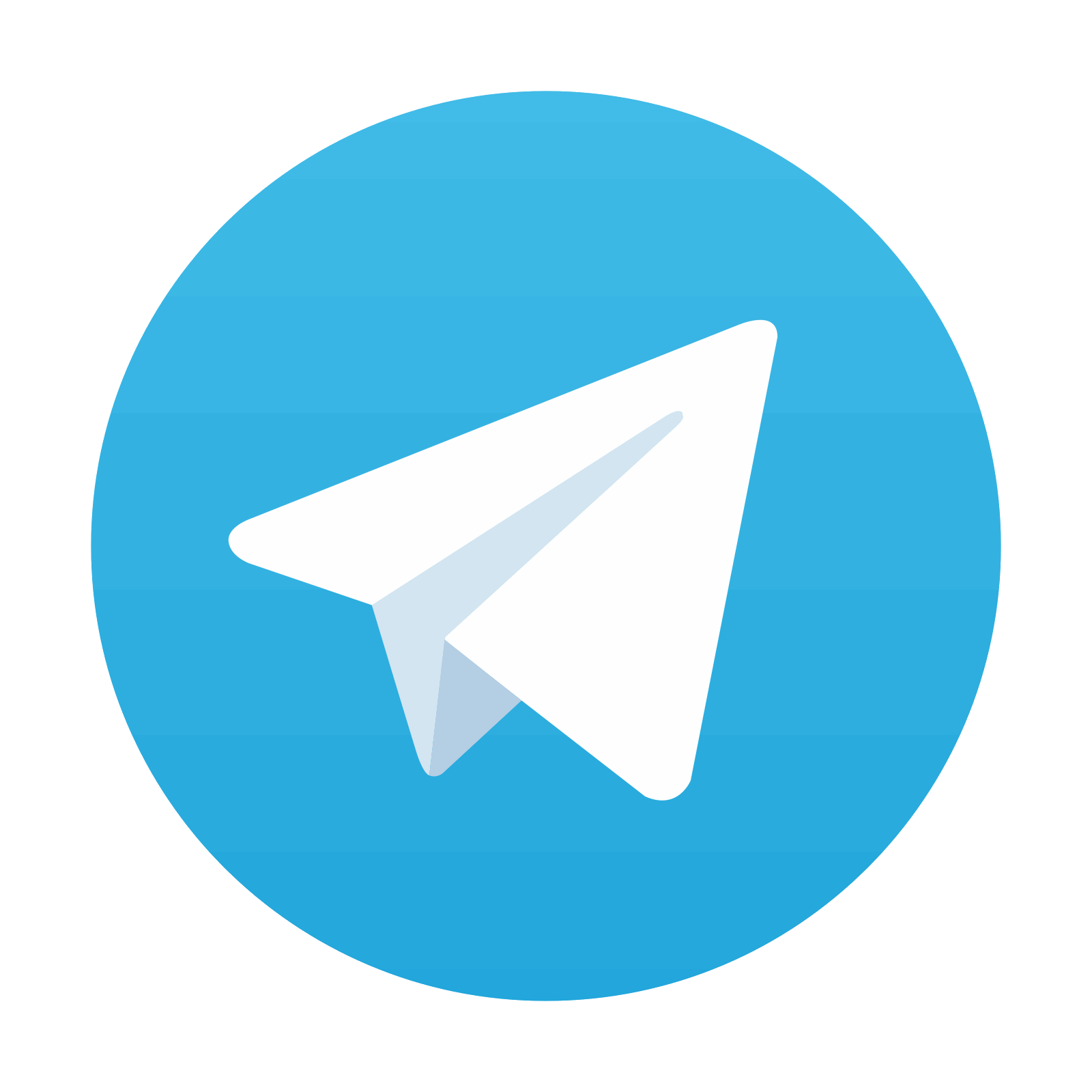
Stay updated, free articles. Join our Telegram channel

Full access? Get Clinical Tree
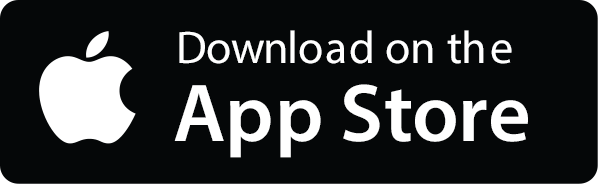
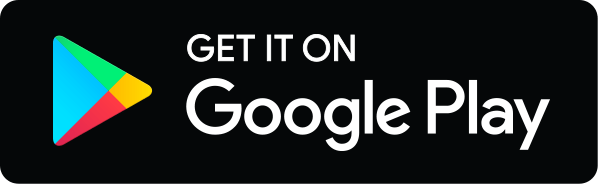