Chapter 20 Clinical nutrition
Vitamin deficiencies
Vitamin deficiency states can arise as a result of:
• inadequate intake (with normal requirements)
• impaired metabolism (if metabolism is necessary for function)
Water-soluble vitamins
Vitamin B1 (thiamin)
Case history 20.1
Investigations
Red cell transketolase activity: | |
without added thiamin pyrophosphate | 2.0 mmol/h/109 red cells |
with added thiamin pyrophosphate | 2.4 mmol/h/109 red cells |
Comment
The patient’s symptoms showed some improvement with the vitamin supplements. Red cell transketolase activity (measured by the decrease in substrate concentration as it is metabolized) was at the lower limit of normal and increased by 20% in the presence of thiamin pyrophosphate. This is consistent with mild thiamin deficiency: an increase of up to 14% is considered normal, while an increase of >25% is clear evidence of deficiency. Peripheral neuropathy is a common clinical problem: thiamin deficiency is but one of many causes (see p. 273).
Nicotinic acid
Part of the body’s nicotinic acid requirement is met by endogenous synthesis from tryptophan. The deficiency syndrome, pellagra (comprising an erythematous skin rash that leads to desquamation, gastrointestinal disturbance, particularly diarrhoea, and dementia) can result from either an inadequate dietary intake of nicotinic acid or decreased synthesis. The latter may be a feature of the carcinoid syndrome (see p. 303), in which there is increased metabolism of tryptophan to hydroxyindoles, with consequently less available for nicotinic acid synthesis, and of Hartnup disease, a rare inherited disorder of the epithelial transport of neutral amino acids, due to decreased intestinal absorption of tryptophan from the gut.
Vitamin C (ascorbic acid)
Ascorbic acid is essential for the hydroxylation of proline residues in collagen and thus for the normal structure and function of this protein. It is a powerful antioxidant and acts by maintaining iron in the hydroxylating enzyme in the reduced (Fe2+) state. Vitamin C also facilitates the intestinal absorption of dietary non-haem iron by keeping it in the Fe2+ state. Subclinical deficiency of ascorbic acid is quite often present in elderly housebound people. The concentration of ascorbate in plasma reflects recent dietary intake and is a poor index of tissue stores of the vitamin. These are better assessed by determination of ascorbate concentration in leukocytes. In practice, this is seldom necessary, because ascorbic acid is cheap and non-toxic, so a therapeutic trial of vitamin supplementation is the simplest procedure to confirm suspected vitamin C deficiency. (See also Case history 20.2.)
Fat-soluble vitamins
Vitamin A
Vitamin A is present in the diet and can also be synthesized from dietary carotenes. It can be measured in plasma, in which it is transported bound to prealbumin and a specific retinol-binding globulin. A low binding protein concentration can cause the plasma concentration of vitamin A to be low and impair its delivery to tissues even when hepatic stores of the vitamin are adequate. Measurements of vitamin A status are rarely required in practice, because deficiency is rare in the western world, but may be useful (together with vitamin E) for monitoring adequacy of pancreatic enzyme replacement in children with cystic fibrosis (see Chapter 16). In areas where deficiency is endemic the facilities required to provide laboratory confirmation of the diagnosis are often not available, although the diagnosis is usually obvious clinically.
Vitamin D
In most individuals, endogenous synthesis is the major source of vitamin D. Privational (dietary) vitamin D deficiency is seen most frequently in people who also have decreased endogenous synthesis, such as the elderly housebound. It is also seen in the UK in people of south Asian origin, particularly women, in whom the effects of low intake may be exacerbated by decreased production by sunlight due to their traditional clothing and darker skin colour. Binding of calcium in the gut by dietary phytates may also contribute to the osteomalacia to which they are prone. Breast milk contains relatively little vitamin D and infants are at risk of vitamin D deficiency particularly if premature (the vitamin is transported across the placenta mainly in the last trimester of pregnancy) or if the mother is vitamin D deficient. Vitamin D insufficiency (Figure 20.1) is increasingly recognized as a public health concern in Europe and North America. At the end of winter, up to one-third of white adults and two-thirds of dark skinned adults may have suboptimal vitamin D concentrations, especially at more northern latitudes.

Figure 20.1 Typical ranges for the interpretation of vitamin D concentration reported by a UK laboratory.
Cholecalciferol itself has little physiological activity. It is hydroxylated first in the liver to 25-hydroxycholecalciferol (25-HCC, calcidiol) and then in the kidney to 1,25-dihydroxycholecalciferol (1,25-DHCC, calcitriol). These metabolites are transported in the circulation by a specific binding protein. Calcitriol is a hormone of vital importance in calcium homoeostasis; its actions and the control of its production are discussed in Chapter 12.
Vitamin D status can be assessed in the laboratory by measurement of the plasma concentration of calcidiol, the major circulating metabolite. This undergoes seasonal variation, being higher in the summer than in the winter. The definition of vitamin D sufficiency has been much debated, but concentrations at which there is no secondary rise in circulating parathyroid hormone are often taken to be optimal. Figure 20.1 shows typical reporting ranges from a UK laboratory.
Decreased synthesis or dietary deficiency of vitamin D causes rickets in children and osteomalacia in adults. Other causes include disordered metabolism of cholecalciferol and malabsorption. The clinical biochemistry of rickets and osteomalacia is considered in more detail in Chapter 15. Vitamin D insufficiency is strongly associated with decreased bone density and an increased risk of fractures, especially in women.
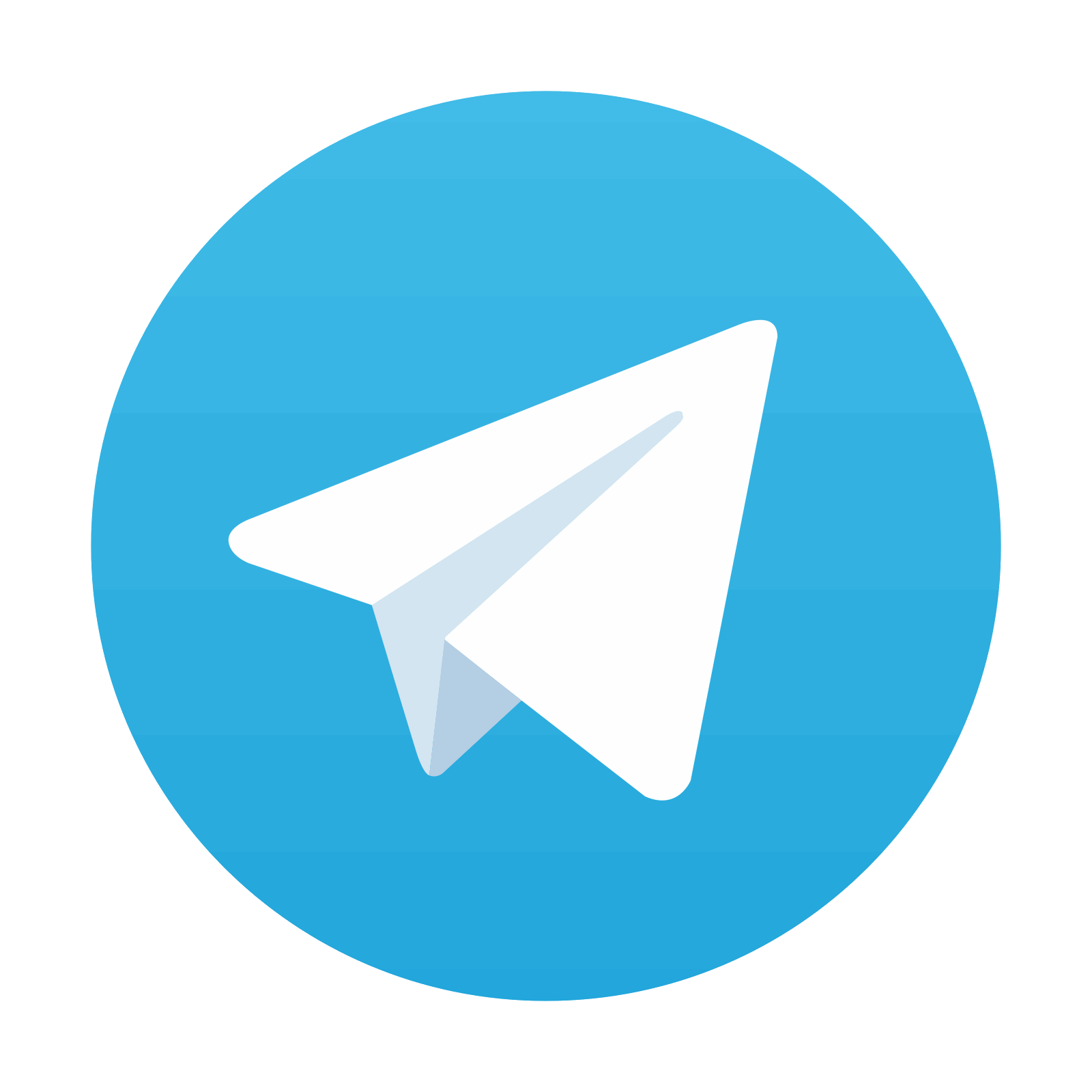
Stay updated, free articles. Join our Telegram channel

Full access? Get Clinical Tree
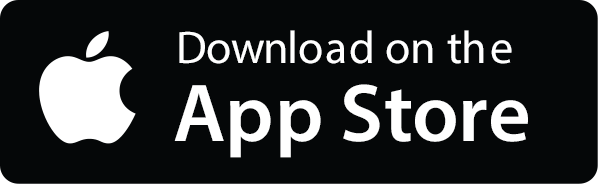
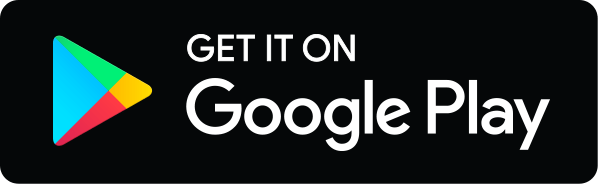