Prior to the decade of the 1980s practitioners of traditional medicine viewed medical genetics as a laboratory science or as an arcane and esoteric discipline. But in the last three decades medical genetics has clearly emerged as a recognized specialty in mainstream medicine. In the 1960s the fields of biochemical genetics, clinical cytogenetics, and dysmorphology (the study of abnormal physical development) arose as disciplines primarily within pediatrics. The 1970s witnessed the establishment of the techniques necessary for the prenatal diagnosis of genetic disorders. By the end of the 1970s discussions about forming the American Board of Medical Genetics had occurred, and in 1981 the first certification examination was administered. The American Board of Genetic Counseling was established in the early 1990s, and now various types of geneticists, including genetic counselors, clinical geneticists, laboratorians, and medical biochemical geneticists, can be certified as specialists within the discipline. In 1991 the American Board of Medical Specialties recognized this new field, and medical genetics became an integral part of medicine. In the last decade the discipline of genomic medicine emerged as advances in next-generation sequencing led to the use of genomic information in direct patient care. The term “genomics” is now regularly added to the title of organizations and academic units of medical genetics.
Whereas medical genetics involves the study of the genetics of human disease, clinical genetics deals with the direct clinical care of persons with genetic diseases. The diagnostic, counseling, and management issues surrounding genetic disease are the principal foci of clinical genetics.
In this chapter, we summarize the principles of clinical genetics and the process of genetic counseling. In addition, we provide an overview of the disciplines of dysmorphology and teratology, because the growth of these fields has influenced and paralleled the emergence of clinical genetics. Lastly, we will close the chapter with a discussion of the important themes in bioethics that relate to medical genetics.
The Principles and Practice of Clinical Genetics
As discussed in Chapter 1 , genetic conditions as a disease group are common and are a significant cause of human mortality and morbidity. Typically, genetic disorders individually are complex, rare, systemic conditions, and the care of persons with these disorders can often involve multiple medical specialties. Thus genetic disorders are among the differential diagnosis of most symptoms and clinical presentations. For example, when evaluating an infant with a blistering skin disease, the ability to distinguish between one of the many forms of epidermolysis bullosa (an inherited disorder of keratinocytes in which skin blisters develop after mild trauma) and staphylococcal skin disease must be part of the clinician’s repertoire.
Because of the complexity and number of human genetic diseases, their clinical diagnosis and treatment can seem overwhelming. To help manage this information, we provide an overview of the most important concepts, including the importance of accurate diagnosis, the application of the tenets of medical genetics to medical practice, and the role of genetic counseling in the care of persons with genetic disease.
Accurate Diagnosis
The significance of the basic medical principle of accurate diagnosis cannot be overemphasized. The process of genetic counseling, one of the principal components of the service of medical genetics, begins with correct diagnosis. All discussions of natural history, prognosis, management, risk determination, options for prenatal diagnosis, and referral to genetic support groups (also termed genetic advocacy groups ) depend on an accurate diagnosis of the patient’s condition. For example, genetic counseling for a family who has a son with an intellectual disability usually includes questions of risk for this condition in future offspring. An accurate answer requires the clinician to identify a condition of known etiology. Once a specific diagnosis (e.g., fragile X syndrome, see Chapter 5 ) is reached, then the genetic counseling process begins. Current information can be shared, management can be initiated, and referral to a support group and/or online resources can occur ( Clinical Commentary 15.1 ).
In clinical genetics, as in all of medicine, accurate diagnosis is the most important first step in patient care.
The long list of syndromes associated with congenital malformations can be overwhelming to the clinician. More than 400 conditions are listed in Smith’s Recognizable Patterns of Human Malformations, and more than 1000 are accessible through the POSSUM or London Dysmorphology computerized databases (see Internet Resources at the end of this chapter). This number imparts a sense that the diagnosis of a malformation syndrome lies in the arena of academic trivia. However, this is not the case.
Consider, for example, the child who is large for gestational age and has a number of physical abnormalities: omphalocele (intestinal protrusion at the umbilicus), large tongue, facial angioma, flank mass, and asymmetrical limb length ( Fig. 15.1 ). His family has questions such as, “What does he have?,” “How will he do?,” “Will he look different?,” “Will he have developmental delays?,” “What is the chance of his condition occurring again in another child?”

By putting these features together and making the pattern recognition diagnosis of the Beckwith–Wiedemann syndrome (BWS), the clinician is able to answer all of the parents’ questions fairly precisely. Most cases of Beckwith–Wiedemann syndrome occur sporadically, but some are inherited. In addition, the genes that are involved in causing the condition exhibit imprinting effects (see Chapter 5 ). If the child has the typical methylation alteration that causes the majority of cases, the sibling recurrence risk is low and less than 1%. If the results of the methylation analysis are normal, the recurrence risk can be higher, and linkage or mutation analysis can provide a more precise risk estimate. In future pregnancies, prenatal diagnosis using sonogram can test for an omphalocele in the second trimester and for large size for gestational age, excessive amniotic fluid (polyhydramnios), and large tongue. If a fetus is thought to have BWS, then the delivery plan would change and the baby should be born in a tertiary care center.
Children with the syndrome do not usually have cognitive disabilities. Although the large tongue can cause orthodontic problems, speech difficulties, and occasionally upper airway problems, these conditions usually improve as the child gets older. The facial appearance is not strikingly abnormal in later childhood.
Chromosome analysis using a cytogenomic microarray (see Chapter 6 ) should be considered, although most Beckwith–Wiedemann patients do not have the chromosome 11p duplication that has been reported in a small number of cases.
The main foci of the medical care plan include regular tumor surveillance because children with BWS have a 5% to10% risk of developing specific embryonal tumors, and the neoplasms are treatable if detected early. The surveillance consists of abdominal sonograms to look for intraabdominal malignancies, especially Wilms tumor and hepatoblastoma, every 3 months, along with serum alpha-fetoprotein (AFP), until age 4 years; thereafter the recommended screening consists of renal sonograms until age 7 years.
In this example, it was important to diagnose the Beckwith–Wiedemann syndrome. The correct label led to precise information for genetic counseling, prediction of natural history (including reassurance), organization of appropriate laboratory studies, a health supervision plan, and referral to the specific genetic support group. Accurate diagnosis was helpful to the parents, the family physician, and the child.
The process of diagnosing a genetic disorder is a complex sequence of events. It depends upon diagnostic decision making, recognition of important phenotypic signs, application of principles of dysmorphology and medical genetics, and laboratory diagnosis. For diseases in which the diagnostic criteria are well established, the practitioner has guidelines for establishing a diagnosis. An example of such guidelines is the criteria recommended by the National Institutes of Health Consensus Development Conference for the diagnosis of neurofibromatosis type 1 (NF1; see Chapter 4 ); these are widely used in clinical practice both in the primary care setting and the medical genetics clinic. For conditions that are defined by a specific laboratory marker, such as an abnormal karyotype or biochemical assay, the diagnostic procedure is generally straightforward. Many genetic diseases qualify as a rare disease, where the definition and delineation of the disorder is not well established, making the process of diagnosis challenging. Additionally, many persons present with a condition that is presumed to be a genetic disease, but is in fact undiagnosed. The undiagnosed patient represents a unique and complex dilemma. Recognition of these challenges led to the establishment of an Undiagnosed Disease Program at the NIH (see Suggested Readings, Gahl, et al.), and later led to the development of a network within the US of such clinics; these advances led to the emergence of such programs throughout Europe and Japan ( Box 15.1 ).
Life Before Diagnosis
At the birth of our second child, nothing was noticeably different. Tosh was a healthy, happy, normal baby boy. We were happy to have a baby that was everything that every parent wants them to be. However, as time went on, we noticed that something was wrong, right around when Tosh was 9 months old. Limbs would give out. He’d fall over a lot as if he had some sort of balance issue. His legs would give out. He’d collapse for no reason. Our pediatrician recommended we see a wide variety of medical specialists; over the next 4 years, we had multiple visits with at least 10 to 12 various doctors and specialists which, unfortunately, resulted in negative tests across the board. This included two genetic tests, multiple MRIs, a spinal tap, EMGs, and many more blood tests etc. This was very frustrating and left us feeling helpless, confused, and without answers. Lots of tears, lots of struggles, sleepless nights, which added stress to the entire family. As a parent, you want the absolute best for your child.
The Next Phase—Discovery
Fortunately for Tosh, and our family, the Penelope Program (our local children’s hospital undiagnosed disease program) was relatively in its infancy. Our team of physicians was aware of our journey, and our case was of interest due to Tosh’s current undiagnosed state. Tosh was a bit of a mystery. We met with our team of doctors, and they were able to explain to us what this new phase meant. It allowed us to gain a better understanding and it gave us something that was running dry … hope. Hope of an answer. As a parent of an undiagnosed child, the thought of there being light at the end of the tunnel is an absolute paradigm shift in how we experience this whole journey. Our sleepless nights were now sleepless because of excitement, because of what we were about to discover. The Penelope team, including the nurse practitioner, the doctors, and many more—too many to list—were so helpful, so compassionate, so thoughtful, and so mindful to be walking us through the genome sequencing, the next step for us. They explained how we would all be tested, including our oldest son. Very exciting, but worrisome to find out what could potentially be wrong with us as parents! LOL.
Diagnosis
Once the results came back, our geneticist took the time to put all this information into more simple terms, to sit us down, and to walk through the results. Tosh’s condition was due to KCND3 , a very rare genetic mutation. So rare, only one other child on the planet was diagnosed at the time. It presents itself as apraxia, ataxia, intellectual plus speech delays, and behavioral issues. (Side note: since we first learned of his mutation, much has changed: at least 20 more cases have been diagnosed around the globe.) This is a big moment. This is a total game-changer. This shifts us into a new zone. And our geneticist knew that, and was very sensitive to that. Forever grateful.
Life After Diagnosis
Our family has since embraced the “Pay-It-Forward” mentality—we wanted another family to receive the services that we did. We wanted another family to have clarity. To have an answer. To have a solution. To have resolve. So, we created a 5K/1M Fundraiser, Tunes for Tosh . That process was rewarding in and of itself. So much support from friends, family, community, the Pediatric Program, etc. After the event last April, the greatest reward was presenting a check back to the program leaders, and the amazing cast of professionals within the Penelope Program. Wow, talk about a tear-jerker. We were able to meet the actual geneticist who found Tosh’s genetic mutation. We could see it in her eyes, we could feel it when we hugged. It was a very moving experience for everyone in the room. One point we’d like to also make: having a diagnosis doesn’t make the world perfect. There’s not an absolute treatment. There’s no “Fix.” We still have questions. We still have bad days. We still get frustrated. We’re human. But we have gained a sense of peace. A sense of knowing. A sense of ownership.
The universe gives special-needs children to regular everyday parents, who choose to step up and be strong for their child! That special child teaches you life lessons that you never could have anticipated, and throughout your journey together, you learn how to become the parent that your child needs. In closing, together, through awareness, love, and community, we can all keep this possibility alive for future families.
Dysmorphic syndromes require knowledge and skills in the recognition of mild malformations, minor anomalies, and phenotypic variations. The diagnosis of other genetic diseases, including cancer syndromes and inborn errors of metabolism, can require expertise from a variety of disciplines. For instance, the diagnosis of any of the forms of retinitis pigmentosa (see Chapter 8 ) necessitates input from an ophthalmologist who is familiar with this group of retinal degenerative conditions. The diagnostic process is further complicated by the variable expression, incomplete penetrance, and heterogeneity of many genetic diseases. These concepts are discussed in Chapter 4 .
Application of the Principles of Medical Genetics
Developing a genetic approach to human disease in the clinical setting requires the application of all of the basic principles of medical genetics discussed in this book. This mind-set is often called “thinking genetically.” For example, making or excluding the diagnosis of NF1 requires knowledge of the clinical variability and age of onset of certain features of the condition ( Clinical Commentary 15.2 ). Recognition of the various forms of neurofibromatosis (i.e., heterogeneity) is crucial in developing a management plan for the individual patient.
One of the common discussions on ward rounds is the notation that a person’s family history is negative or “noncontributory.” This is often thought to exclude a genetic disorder. However, the majority of persons who have a genetic disease do not have a positive family history. A quick review of the mechanisms of Mendelian, chromosomal, and multifactorial disease inheritance illustrates that a lack of other affected persons in the family is common and does not by any means exclude the presence of a genetic disease. For example, the sibling recurrence risk is 25% for diseases with autosomal recessive inheritance. Thus a significant number of families with multiple offspring have only one affected child and no family history. Even some well-established autosomal dominant disorders often present a “negative” family history because of high proportions of new (“ de novo ”) mutations (examples include Marfan syndrome, neurofibromatosis type 1 [NF1], and achondroplasia, in which the percentages of cases caused by new mutations are 30%, 50%, and 80%, respectively). Chromosomal syndromes usually have a low recurrence risk. Even when a parent carries a balanced chromosome rearrangement, the recurrence risk among the offspring is usually less than 15%. The sibling recurrence risks for multifactorial conditions are usually 5% or less.
Case
A family comes to clinic with a 6-year-old boy who has 10 café-au-lait spots exceeding 0.5 cm in diameter widely distributed on the trunk and limbs and an optic glioma detected on a brain MRI ( Fig. 15.2 ). The family has questions about the diagnosis and the recurrence risk in future pregnancies. On initial telephone contact it is learned that there is no history of a family member with similar features.

There are several possible explanations for this finding. Exploring them underscores the implications of the so-called “negative family history”:
- ▪
New mutation of the NF1 gene. Because of the relatively high percentage of new mutations for this disorder, this is the most likely explanation.
- ▪
Variable expression. It is also possible that one of the parents carries the gene but has mild expression of the phenotype. Occasionally a parent has multiple café-au-lait spots and a few unrecognized neurofibromas, but a diagnosis of NF1 has never been made. Thus it is important to evaluate the parents for a mild expression of NF1.
- ▪
Incomplete penetrance. This is a possibility; however, it is unlikely for NF1, in which penetrance is close to 100%. If a family has two children with NF1 and neither parent has the gene, germline mosaicism would be the more likely explanation.
- ▪
Incorrect diagnosis. One of the assumptions and basic principles of medical genetics is accurate diagnosis. This patient meets the National Institutes of Health established criteria for NF1 (see Chapter 4 ). However, if this patient had only café-au-lait spots, then the certainty of diagnosis would have been an issue. One would need to know the differential diagnosis of multiple café-au-lait spots.
- ▪
False paternity. Although it is relatively unlikely, this possibility must be kept in mind.
We began with an individual who had a classic autosomal dominant disorder with no family history. This can be explained in a number of ways. The statement that there is “a negative family history” should not be considered conclusive evidence against the presence of a heritable condition.
Knowledge of the other formal principles of medical genetics is also necessary in the care of persons with genetic conditions. The accumulation of family history data and the interpretation of pedigree information are important in answering a family’s questions regarding risk of recurrence. An understanding of the various modes of inheritance is required in any explanation of recurrence risk. Discussion of the concepts of new mutation and pleiotropy are commonplace in reviewing the cause and pathogenesis of a genetic disease with a family. Even an understanding of meiosis is a requirement for discussions of the process of nondisjunction with the family of a newborn with Down syndrome (See Chapter 2 and Clinical Commentary 15.3 ).
The birth of a newborn with Down syndrome presents many challenges. Typically, the infant is not acutely ill, and unless prenatal diagnosis has been carried out (see Chapter 13 ), the parents will not be aware of the diagnosis before the birth. Thus the practitioner must approach the parents, often strangers, with unexpected and sometimes difficult news. The family can experience a series of emotions that are somewhat similar to the reactions after a loss: anger, denial, sadness, and then usually reorganization and adaptation. Families face these situations with markedly different backgrounds; varying attitudes toward crisis, different demographic and socioeconomic circumstances, and a wide range of differences in the cultural meaning of a disability or birth defect all play a role in this setting. All of these variables, plus the fact that physicians are often not trained in being the bearers of unexpected news, can make this a challenging situation. Parents remember in detail the way the news is presented. The practitioner has both the opportunity and the challenge to help and guide the family through these events.
A number of practical suggestions have come from studies investigating the recommendations of parents who have experienced this event:
- ▪
Prepare yourself. Set up the interview scenario, and think about how you will begin the discussion. Think about the script. Starting with “Congratulations on the birth “ of their son or daughter establishes a caring tone from the outset.
- ▪
Talk to both parents together whenever possible. This is sometimes not practical, but when it can be accomplished, it is critical.
- ▪
Communicate the diagnosis as soon as possible. All studies of parental interviews show that they prefer early communication of the diagnosis.
- ▪
Choose a place that is private and quiet where both the parents and the professionals can sit down. Avoid standing up with the parents seated. Always be sure to introduce yourself. Structure the interview from the beginning.
- ▪
Humanize the situation as much as possible. Learn the baby’s first name if it has been decided on, and always know the baby’s gender. Refer to the infant by name or as a son or daughter, and be aware of the use of all language. Phrases such as “mental retardation” have great impact, and currently that term is not considered the appropriate designation by professionals in pediatrics, psychology, and medical genetics (it has been replaced by intellectual disability or cognitive disability). Terms such as “mongolism” are not appropriate because they are stigmatizing, pejorative, and incorrect.
- ▪
Develop a sense of realistic positivism. It is important to discuss the developmental challenges in a person with Down syndrome, but it is also important to have an optimistic and positive attitude. Phrases or language conveying a loss of hope are not appropriate. This suggestion comes from the advocacy and parents’ organizations that have developed in the last six decades.
- ▪
Answer the parents’ questions, but avoid technical overload. It is important to be accurate and current on the biological and medical aspects of the condition under discussion. When an answer is not known, mention that the question can be reviewed or referred to a consultant.
- ▪
Listen actively. Assume that almost all feelings are natural and that parents will be wrestling with their own guilt and shame. Validate all feelings that arise. Most parents can meet this challenge quite effectively and do not require psychiatric consultation.
- ▪
Refer the family to the appropriate resources early. This would include early intervention programs, parent advocacy groups, and even individual parents who have a child with Down syndrome. Share available written material and online resources, but make certain that they are accurate and current.
Above all, be aware of the unique plight of families in such a situation, and make an effort to spend time with them. Although it is difficult to present in written form how one can develop attributes such as kindness and empathy, it is important for physicians in training to learn from their mentors and use their own individual communication style as a strength. Clearly, the recommendations provided here apply not only to genetic counseling, but also to any situation in which difficult information is presented to patients or families. (See Suggested Readings at the end of the chapter.)
Genetic Counseling: Definition and Principles
Genetic counseling represents one of the central skills of medical genetics and along with accurate diagnosis comprises the cornerstones of the field. At first glance, the use of the term “counseling” implies that this service lies in the domain of mental health, social work, or psychotherapy. But in fact, genetic counseling is centered in the conventional medical model because it depends significantly on accurate diagnosis and knowledge of medical genetics. As a tradition, genetic counseling grew out of the field of human genetics rather than from behavioral science, unlike other counseling disciplines.
In 1975 the American Society of Human Genetics adopted a definition of genetic counseling. Newer language has been proposed recently to modernize and clarify this definition, but the original language helps define the goals of care both then and now:
Genetic counseling is a communication process that deals with the human problems associated with the occurrence or risk of occurrence of a genetic disorder in a family. This process involves an attempt by one or more appropriately trained persons to help the individual or family to (1) comprehend the medical facts, including the diagnosis, probable course of the disorder, and the available management; (2) appreciate the way heredity contributes to the disorder and the risk of recurrence in specified relatives; (3) understand the alternatives for dealing with the risk of recurrence; (4) choose a course of action that seems to them appropriate in their view of their risk, their family goals, and their ethical and religious standards, and act in accordance with that decision; and (5) make the best possible adjustment to the disorder in an affected family member and/or to the risk of recurrence of that disorder.
This definition illustrates the complex tasks that face the practitioner. The first task involves establishing the diagnosis and discussing the natural history and management of the condition. In this regard, the medical care of a patient with a genetic disease does not differ from that of a patient with any type of human disease.
The second task requires an understanding of the basic tenets of medical genetics, especially the principles of human genetics and risk determination discussed throughout the text. For chromosomal and multifactorial disorders, empirical figures are used to estimate recurrence risk. Knowledge of inheritance patterns is applied to predict the recurrence risk of Mendelian disorders. However, the clinical issues are often complicated by incomplete penetrance, variable expression, delayed age of onset, and allelic and locus heterogeneity. In some cases, incorporation of additional information using the Bayesian probability approach can significantly assist in providing estimates ( Box 15.2 ).
The estimation of recurrence risks was treated at some length in Chapter 4, Chapter 5 . A typical example of recurrence risk estimation is a case in which a man with hemophilia A, an X-linked recessive disorder, produces a daughter (individual II-1 in the following diagram). Because the man can transmit only the X chromosome carrying the hemophilia A mutation to his daughter, she must be a carrier. The carrier’s daughter, individual III-6, has a 50% chance of receiving the X chromosome carrying the mutation and being herself a carrier. Even though the daughter in generation III has five normal brothers, her risk remains 50% because we know that the mother in generation II is a carrier.

Suppose now that the woman in generation III produces three sons (generation IV), none of whom has hemophilia A. Intuitively, we might begin to suspect that she is not a carrier after all. How can we incorporate this new information into our recurrence risk estimate?
A statistical principle that allows us to make use of such information is called Bayes theorem (the application of Bayes theorem is often termed Bayesian analysis or Bayesian inference). The table below summarizes the basic steps involved in Bayesian analysis. We begin with the prior probability that the woman in generation III is a carrier. As its name suggests, the prior probability denotes the probability that she is a carrier before we account for the fact that she has produced three normal sons. Because we know her mother is a carrier, this woman’s prior probability must be 1/2. Then the prior probability that she is not a carrier is 1−1/2, or 1/2.
She is a Carrier | She is Not a Carrier | |
---|---|---|
Prior probability | 1/2 | 1/2 |
Conditional probability | 1/8 | 1 |
Joint probability | 1/16 | 1/2 |
Posterior probability | 1/9 | 8/9 |
Next we take into account the woman’s three normal sons by estimating the probability that all three of them would be normal given that she is a carrier. Because this probability is conditioned on her carrier status, it is termed a conditional probability. If she is a carrier, the conditional probability that all three of her sons are normal would be (1/2) 3 , or 1/8. We also estimate the probability that all of her sons would be normal given that she is not a carrier. This conditional probability is, of course, very close to 1.
Next we want to find the probability that the woman is a carrier and that she is a carrier with three normal sons. To obtain the probability of the cooccurrence of these two events, we multiply the prior probability times the conditional probability to derive a joint probability (i.e., the probability of both events occurring together, a concept discussed in Chapter 4 ). The joint probability that she is a carrier is then 1/2 × 1/8 = 1/16. Similarly, the joint probability that she is not a carrier is 1/2 × 1 = 1/2. These joint probabilities indicate the woman is 8 times more likely not to be a carrier than to be a carrier.
The final step is to standardize the joint probabilities so that the two probabilities under consideration (i.e., being a carrier versus not being a carrier) sum to 1. To do this, we simply divide the joint probability that the woman is a carrier (1/16) by the sum of the two joint probabilities (1/16 + 1/2). This yields a posterior probability of 1/9 that she is a carrier and 8/9 that she is not a carrier. Notice that this standardization process allows us to provide a risk estimate (1/9, or 11%), while preserving the odds of noncarrier versus carrier status indicated by the joint probabilities.
Having worked through the Bayesian analysis, we see that our intuition was confirmed: The fact that the woman in question produced three normal sons reduced her risk of being a carrier substantially, from an initial estimate of 50% to a final probability of only 11%.
Another common application of Bayesian analysis is illustrated in part A of the diagram that follows. The male in generation II is affected with Duchenne muscular dystrophy (DMD), a lethal X-linked recessive disease (see Chapter 5 ). Either his unaffected mother is a carrier of the mutation, or he received a new mutation on the X chromosome transmitted by his mother. It is important to determine whether the mother is a carrier or not, because this fact will influence recurrence risks for DMD in her subsequent offspring. If the mother has only one affected offspring, the probability that she is a carrier can be evaluated directly, because one-third of all cases of X-linked lethal recessive disorders arise as a result of new mutations. (To understand this, consider the fact that because females have two X chromosomes and males have only one, 2/3 of all X-linked disease-causing mutations in a population must be found in females. For a lethal X-linked recessive, all of the male X chromosomes are eliminated from the population in each generation. Yet the frequency of the mutation remains the same, generation after generation. This is because new disease-causing mutations arise at the same rate as the loss of mutation-containing X chromosomes. Because one-third of the mutation-containing X chromosomes are lost each generation, it follows that one-third of the mutations in the population must occur as the result of new mutation.) If the probability that the affected son received a new mutation is 1/3, then the probability that the mother is a carrier—the alternative possibility—must be 1−1/3, or 2/3.
In the table below, we use Bayesian analysis to evaluate the probability that the mother is a carrier. As in the previous example, we derive a prior probability that she is a carrier, assuming no knowledge that she has produced an affected son. This probability is given by 4μ, where μ is the mutation rate for the DMD locus (i.e., the probability, per generation, that a disease-causing mutation arises at this locus in an individual). The derivation of the probability, 4μ, is beyond the scope of this text, but it can be found elsewhere (see Suggested Readings, Hodge, 1998). Because the prior probability that the mother is a carrier is 4μ, the prior probability that she is not a carrier is 1−4μ, which is approximately equal to 1 because μ is very small. The conditional probability that the woman transmits the mutation given that she is a carrier is 1/2 (there is also a very small probability that she transmits her normal allele, which is then mutated, but this can be ignored). The conditional probability that she transmits a mutation given that she is not a carrier (i.e., the probability that a new mutation arises in the gamete she transmits) is μ. We then multiply the prior probability that she is a carrier, 4μ, by the corresponding conditional probability, 1/2, to obtain a joint probability of 2μ. The same procedure produces a joint probability of μ that she is not a carrier. Finally, we standardize the joint probabilities to get the posterior probabilities. The posterior probability that she is a carrier is 2μ ÷ (2μ + μ) = 2/3, and the posterior probability that she is not a carrier is μ ÷ (2μ + μ) = 1/3. As expected, these probabilities correspond to the ones we obtained by simple direct observation.
She is a Carrier | She is Not a Carrier | |
---|---|---|
Prior probability | 4μ | 1 − 4μ ≈ 1 |
Conditional probability | 1/2 | μ |
Joint probability | 2μ | μ |
Posterior probability | 2/3 | 1/3 |
Suppose, however, that the woman has had an affected son and an unaffected son (see part B of the figure below). This gives us additional information, and intuitively it increases the possibility that she is not a carrier (i.e., that the one affected offspring is the result of a new mutation). In the table below, we incorporate this new information. The prior probabilities remain the same as before (i.e., we assume no knowledge of either of her offspring). But the conditional probability of transmission, given that she is a carrier, changes to account for the fact that she now has two offspring: 1/2 × 1/2 = 1/4 (i.e., the probability that she did not transmit the mutation to one offspring times the probability that she did transmit the mutation to the other offspring). The conditional probability that she transmitted a new mutation to the affected offspring is μ, and the probability that she did not transmit a mutation to the unaffected offspring is 1−μ. Thus the probability of both events, given that she is not a carrier, is μ × (1−μ) ≈ μ. The joint and posterior probabilities are obtained as before, and we see that the woman’s chance of being a carrier is now reduced from 2/3 to 1/2. Again, this confirms (and quantifies) our expectation.

She is a Carrier | She is Not a Carrier | |
---|---|---|
Prior probability | 4μ | 1 − 4μ ≈ 1 |
Conditional probability | 1/4 | μ × (1 − μ) ≈ μ |
Joint probability | μ | μ |
Posterior probability | 1/2 | 1/2 |
Before the advent of disease diagnosis through linked markers or mutation detection, Bayesian analysis was often the only way to derive a risk estimate in situations such as these. Now, of course, an attempt would be made to identify the factor VIII or DMD mutation that causes hemophilia A or DMD in these families directly, or, failing that, linked markers would be used. This is a much more direct and accurate approach for determining carrier status. However, as discussed in Chapter 13 , it is not always possible to identify the responsible mutation, particularly when a large number of mutations can cause the disorder (as is the case for hemophilia A, DMD, or cystic fibrosis). Bayesian inference can be used in such cases to incorporate the sensitivity of the genetic test (e.g., if a standard mutation analysis of the CFTR gene reveals 85% of the mutations [see Chapter 13 ], there is a 15% probability that the person in question has the mutation even though the test did not reveal it). In addition, linkage analysis is not always informative. Thus Bayesian analysis is still sometimes a useful tool for refining risk estimates.
The additional information incorporated in Bayesian analysis is not confined to the assessment of health status in relatives, as was shown in these examples. Another type of information is a biochemical assay, such as factor VIII activity level, that could help to indicate carrier status. Because there is usually overlap between carriers and normal homozygotes for such tests, the assay cannot determine carrier status with certainty, but it does provide a probability estimate for incorporation into Bayesian analysis. In diseases with delayed age of onset, such as adult polycystic kidney disease, the probability of being affected at a certain age can be used in a Bayesian analysis. Here, one considers the fact that the at-risk person is less and less likely to possess the disease gene if he or she remains unaffected beyond a certain age.
The third and fourth objectives of the genetic counseling process underlie the primary differences between the genetic model and the traditional biomedical approach. These tasks involve discussing reproductive options and facilitating personal decision making. Implicit in the fourth part of the definition is the notion of respect for the family’s autonomy and their perceptions of risk and of the disorder itself. This approach conventionally has been called nondirectiveness: the counselor leaves all decisions about future reproduction and medical care up to the family. This differs somewhat from the more traditional medical approach, in which recommendations for treatment or intervention are often made in a more directed fashion. This is an important issue, because nondirectiveness sometimes conflicts with the broader view of preventive medicine, which might suggest that the principal goal of genetic counseling should be the reduction of the incidence of genetic diseases.
In recent years conventional and mainstream medicine has embraced a similar approach to counseling with the emergence of the concept of shared decision making . The central tenets of this approach are remarkably similar to what has been integral to genetic counseling for 40 years.
Historically, the principle of nondirectiveness developed in the arena of reproductive counseling and in the context of decisions surrounding prenatal diagnosis. If prevention or reduction of disease is the primary goal, then one’s approach would logically be more directive. However, the main goal of genetic counseling is to help an individual family understand and cope with genetic disease and make the best decision in the context of their individual values, not to reduce the incidence of genetic disease.
Although most geneticists subscribe to the principles of autonomy and nondirectiveness, it may be challenging to the clinician to be entirely nondirective, simply because of the limitations of a time-restricted session and the inherent biases of the clinician. It is challenging as a clinician to avoid reflecting one’s own biases. For example, an explanation of nutritional management of an infant with a disease detected by newborn screening (see Chapter 13 ) would require a more directive approach than the discussion of disease risks in future pregnancies. Nondirectiveness may be challenging when the consequences of disease are serious, as in high-risk cancer counseling (see the discussion of the newly proposed definition below). In addition, information may be presented quite differently in different contexts. Information about Down syndrome, for example, may be communicated differently depending on whether the diagnosis was made prenatally or after the birth of an affected newborn (see Clinical Commentary 15.3 ), although we would suggest that the information needs to be presented in a balanced manner in either situation.
In 2006 leaders in the field of genetic counseling and the National Society of Genetic Counselors reached a consensus on an updated and revised definition of genetic counseling. This effort was relevant and timely because in the three decades since the original definition, the field had grown remarkably to include the disciplines of adult and cancer genetics:
Genetic counseling is the process of helping people understand and adapt to the medical, psychological, and familial implications of genetic contributions of disease. The process integrates the following: (1) interpretation of family and medical histories to assess the chance of disease occurrence or recurrence; (2) education about inheritance, testing, management, prevention, resources, and research; and (3) counseling to promote informed choices and adaptation to the risk of the condition.
The revised definition emphasizes the dual and integrated roles of education and counseling.
The majority of geneticists subscribe to the principle of nondirectiveness in reproductive counseling. Information about risks, natural history, treatment, and outcome is presented in a balanced and neutral manner, and decisions about reproduction are left to the family.
The facilitation of discussion surrounding decision making is central to the task of genetic counseling regardless of the setting (i.e., prenatal, pediatric, or cancer clinic). Several factors are involved in a family’s decision about future pregnancies when there is an increased risk. The obvious ones are the magnitude of the risk figure and the burden or impact of the disorder. However, these are not the only significant issues. The individual family’s perception of the impact of the condition is likely more important in their decision making than the professional’s perception of the burden. The meaning of children to the individual family, according to their own cultural, religious, or personal preferences, is weighed heavily in the reproductive decision-making process. In addition, families frequently play out the scenario of coping with a recurrence of the condition in another child. Identification of these issues for a family often helps to stimulate their own discussions. Some families perceive a recurrence risk qualitatively rather than quantitatively. They consider themselves to be either “at risk” or not, with the actual risk estimate being a secondary consideration. The fact that there is so much variation in the importance people assign to each of these factors (perception of risk, perception of impact, meaning of children, and possibility of recurrence) underscores the point that the professional should be a facilitator and not the decision maker. Awareness of the family’s views and values is crucial to help in this complex undertaking.
A central task of genetic counseling is to help the family cope with the presence of the disorder or its recurrence risk, or both. This task is similar to the physician’s support of a family dealing with any chronic disease, cancer, or disability. What is unique, perhaps, is the family’s perception of the meaning of a genetic disorder ( Boxes 15.3 and 15.4 ). In many acquired conditions, such as infections or accidents, the ultimate meaning of the condition is externalized. In genetic disorders, the condition is more intrinsic to the individual and the family; it thus often presents a complex personal dilemma. Validation of the plight of families is vital and is probably more effective than simplistic attempts to remove guilt. Feelings of guilt and shame are natural to the situation and also require acknowledgment. These concepts exemplify opportunities in the scenarios for the counselor to show empathy for the plight of the family.
Our daughter Juliett arrived on a beautiful summer afternoon. Late in my pregnancy, an ultrasound had showed an enlarged heart, dilated left kidney, and possible malformation of the cerebellum. During labor, our daughter’s heart rate decelerated significantly, and we were given the option of an emergency C-section. Without hesitation, we opted for the C-section. A drape was hung so that I could not see, and I only knew that the baby was born when the pediatrician ran out of the room with something in his arms. My husband quickly followed, and then I waited for what seemed an eternity.
Juliett weighed 4 pounds, 6 ounces and was 18 inches long. I graduated as an RN just before Juliett was conceived and had worked in a pediatric ICU. I had just enough experience to pick up a few of her obvious problems, but many escaped me. She was clearly much too thin, and her rib cage looked too short and prominent. But compared to the mental images I had formed after the ultrasound, I was relieved to see how beautiful she was. Her most striking feature was her incredible blue eyes, which were wide open and very alert. Her nose and mouth were beautifully formed and very petite. As my husband and I sat in awe over her, a neonatologist entered the room. He pointed out several characteristics, the only one of which I clearly remember was the clenched fist with the index finger lying over the middle finger. He concluded that she probably had trisomy 18. Of the grim things he rattled off, the only thing I remembered was that he said she would be a vegetable and that she would most likely die within the next couple of days. He then walked away, and we sat there, stunned. In this state of grief and turmoil I tried to understand how this clenched fist could lead to death, and how these bright, alert eyes could belong to a vegetable.
In the days that followed, I often opened up her fist and laid her fingers straight, hoping that the blood tests would not confirm the doctor’s suspicions. Our bonding with Juliett had been instant, and our great desire was to be able to take her home before she died. As she began to tolerate feedings and was weaned away from oxygen, our pleas to take her home were granted.
We left with no follow-up or care plan. Each time she went to sleep, we prayed she would wake up again and that we could complete another feeding. At 3 months of age, she started to smile at us, and our hopes brightened. We have been fortunate to see her outlive the grim statistics, and we have learned that there is no clear explanation why some children with this condition live longer than others. Juliett’s heart was enlarged because of a defect similar to a tetralogy of Fallot. Mild scoliosis at birth has now progressed to a 100-degree lumbar curve and a 90-degree thoracic curve. Despite her many physical challenges, Juliett has continued at her slower pace to learn and develop new skills. Her personality is delightful, and people are often surprised to see how responsive and interactive she is.
We have often been asked if we were afraid to have more children. Perhaps we were crazy, but we felt that another like Juliett would be great. We have had four more girls. To everyone’s surprise, our fifth child, Camille, was born with Down syndrome. With Juliett, the grieving process had been covered up with the gratitude we felt that she was even alive. With Camille, we experienced the more typical grieving process.
On the day of Juliett’s birth, a pediatrician came forward, put his arms around us, and told us he thought she was beautiful and to love her for as long as she could be with us. He turned her into a human being with a life to be highly valued. In the 13 years that have followed, Juliett has seen many doctors. Most of them, although they could not cure her problems, gave us the most important thing we needed: to know that our daughter’s life was of great worth and that, if they could, they would do anything to help her.
Tommy was born via an emergency cesarean, because 1 week before his delivery date his fetal movements markedly decreased. At birth, he weighed only 4 pounds, and the first time that I saw him he was in an incubator connected to all sorts of tubes. He spent his first month of life in the neonatal intensive care unit so that his weight gain could be closely monitored. Because he was so small, he was fed through a feeding tube for many months, and as a consequence, he refused to drink from a bottle. Eventually, he overcame his aversion to using his mouth to eat but only after substantial training. Nevertheless, despite our care, Tommy remained small for his age.
The following summer, Tommy developed dark red marks on his cheeks and under his eyes. Our pediatrician referred us to a dermatologist, who suspected that the marks on Tommy’s face were related to his growth failure. We were very surprised. How could these two findings be related? That is when we were told that Tommy might have a genetic disorder called Bloom syndrome. We hoped that the doctor was wrong, but soon thereafter Tommy had a genetic test that measured the number of sister chromatid exchanges per cell (see Chapter 2 ). This test confirmed that Tommy had Bloom syndrome. Although I insisted that it was a false-positive result, I learned to accept that our son had a very rare cancer syndrome.
We were barraged by questions from family, friends, and doctors. As a result, we became very protective of our son and his privacy. Nevertheless, there was only so much we could do to protect him because he is such a social little boy, loving to play with family and friends. This also made choosing an appropriate elementary school a very difficult decision for us. We expected that children would pick on him because of his small size. However, to our surprise, he easily developed friendships and adjusted well to his classmates. In fact, the problems that he did develop were largely because of his misbehavior. Thus we struggled to find a balance between protecting Tommy while not permitting him special privileges because of his small stature.
In our home we try to treat Tommy like any of our other children. One challenge for us is that because of Tommy’s small size, people wrongly perceive that he is much younger than his chronological age. This is very frustrating for Tommy, yet we occasionally reinforce this image because of our concern for his safety. For example, although Tommy is 6 years old, he weighs only 21 pounds. Thus he must sit in an infant seat when he travels in a car, and we explain to Tommy’s friends that it helps him see through the windows. Another safety problem is that many of the sensors for the automatic doors at supermarkets cannot detect his presence and easily slam into him.
Overall, Tommy has adapted well. He climbs or jumps in order to reach things. To keep up with his peers, he often runs, hops, or jumps instead of walking. We constantly worry about his safety, but we cannot control all that happens to him. To date, he has been healthy, and although it seems like we have been riding an emotional roller-coaster, we wouldn’t trade our experiences for anything.
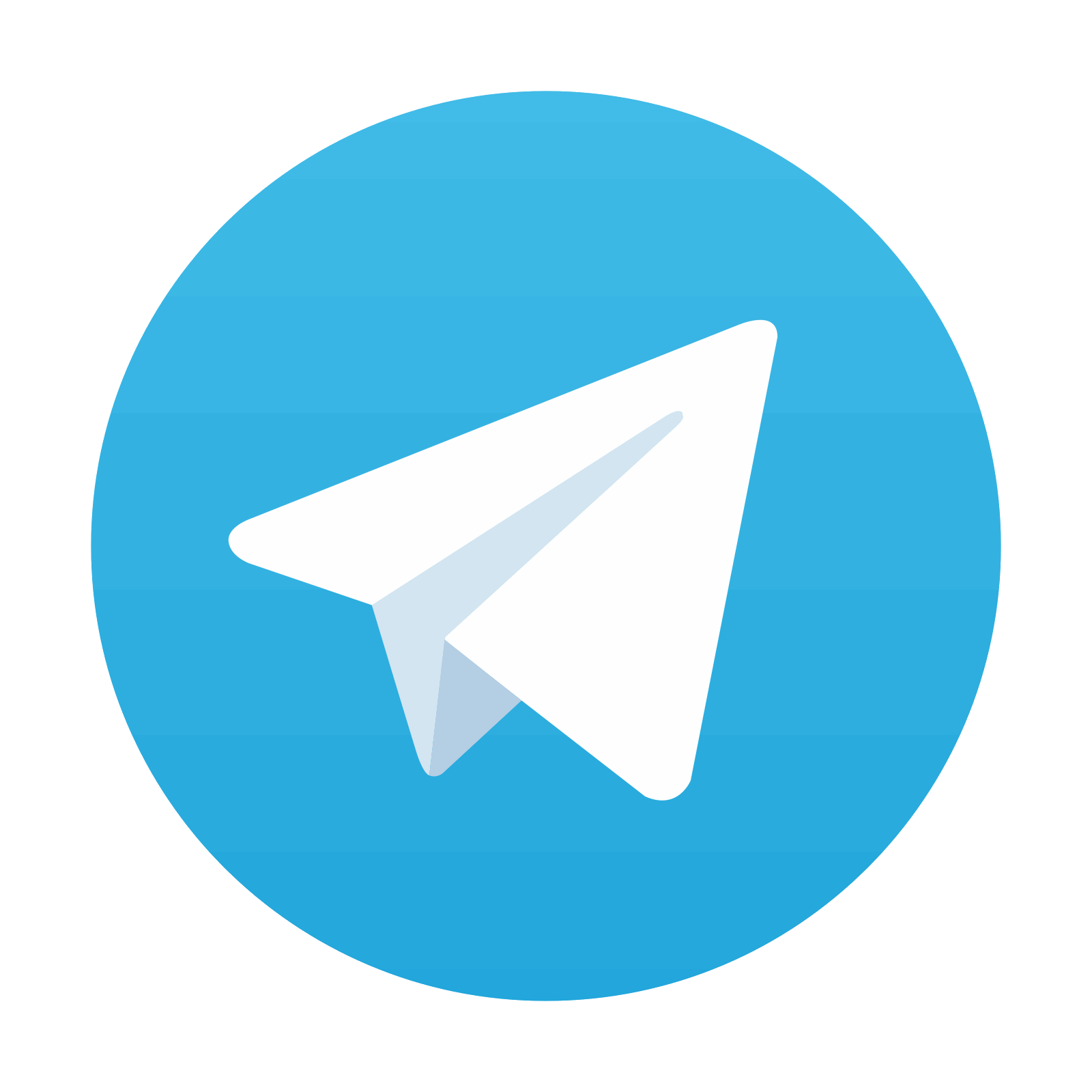
Stay updated, free articles. Join our Telegram channel

Full access? Get Clinical Tree
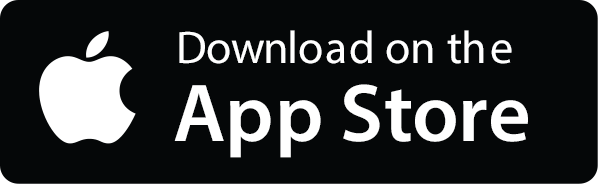
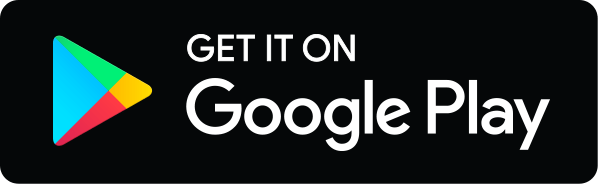
