Clinical Biochemistry at the Extremes of Age
This chapter looks at clinical biochemistry at the extremes of age, that is, in neonates and the elderly.
NEONATES
Some preliminary definitions are useful. A premature or preterm baby is one born before 37 completed weeks’ gestation. A neonate is a live baby within 1 month of birth. A low-birthweight baby is one less than 2.5 kg and a very low-birthweight baby is one less than 1.5 kg.
One of the most significant advances in recent medical care has been the survival rate of very small preterm infants, which has increased because of improved specialized medical and nursing techniques for treating the neonate. However, these infants can experience a number of abnormal biochemical conditions, which are briefly summarized in this chapter.
Diseases occurring during the neonatal period can be divided into two main groups: those of infants born before term, in whom immaturity contributes to the severity of the disease, and those of full-term infants. The most common disorders in both groups are perinatal asphyxia, the respiratory distress syndrome (RDS), infection and inborn errors.
Compared with about 5 L in a 70-kg adult, the blood volume of a premature infant weighing 1 kg is only about 90 mL. Therefore only a small amount of blood can be taken without causing volume depletion or anaemia. Sometimes capillary samples are used as an alternative to venous (or arterial) samples, although the former may be more prone to contamination from the interstitial and cellular fluids. Prior to requesting tests on small samples, the clinician should contact the laboratory to discuss the best strategy for obtaining test samples and prioritizing of investigations. The interpretation of test results may be influenced by the different reference ranges at different ages, which can be difficult to define.
Renal function
The kidneys usually have a full complement of nephrons by about the 36th week of gestation, but renal function is not fully developed until the age of about 2 years. Glomerular function develops more rapidly than that of the tubules. Fetal urine is produced from about the ninth week.
The glomerular filtration rate (GFR) doubles during the first 2 weeks of life because of an increase in renal blood flow. The GFR (which is corrected for surface area) reaches adult values by about 6 months of age.
The plasma urea concentration is low in newborn infants compared with that in adults, despite the relatively low GFR; the high anabolic rate results in more nitrogen being incorporated into protein rather than into urea than in adults. Plasma urea concentrations fluctuate markedly with varying nutritional states, metabolic rates and states of hydration.
The plasma creatinine concentration (which is inversely related to GFR) at birth is similar to the mother’s. It decreases rapidly at first, averaging about 35 µmol/L, the fall being slower in preterm infants, and then rises before reaching adult values (corrected for the body surface area) by about 6 months.
Renal function in newborn infants can maintain basic homeostasis but may not be able to respond adequately to illness or other stresses. It is often difficult to determine if there is renal impairment because of the unsatisfactory nature of renal function tests at this age. A plasma urea concentration above about 8 mmol/L suggests retention due to glomerular impairment, whether parenchymal or pre-renal in origin, especially if the urinary output can be shown to be low. Management depends to a large extent on clinical criteria. In the neonate, neither the plasma urea nor the creatinine concentration is a very sensitive indicator of renal function.
Renal dysfunction in neonates can be due to factors such as hypotension, birth asphyxia, renal toxic drugs, renal agenesis, septicaemia or dehydration or can occur post surgery.
The daily excretion of urine is about 15-60 mL in full-term neonates, increasing to about 500-600 mL/day at about 1 year (see Chapter 3).
Water
About 80 per cent of the weight of a neonate consists of water, compared with about 60 per cent of that of an adult; the relative amount of water is related more to the proportion of fat to lean tissue than to total body weight. Proportionally, there is probably more water in the extracellular than in the intracellular compartment at birth. During the first week of life, the extracellular fluid (ECF) compartment contracts; urinary loss may account for the relatively high total amount of sodium excreted by preterm infants and does not necessarily indicate a disturbance in sodium homeostasis.
Renal concentrating capacity is lower in the newborn infant than in the adult and increases during the first 2 years; the maximum urinary osmolality that can be achieved in response to water deprivation, even if stimulated by exogenous antidiuretic hormone (ADH), is between 500 and 700 mmol/kg. The countercurrent mechanism is relatively inefficient, partly because the loops of Henle do not penetrate as deeply into the renal medulla as in the adult and partly because of the relatively low rate of urea production and therefore excretion. Reabsorption of urea from the collecting ducts contributes to the interstitial medullary osmolality, so facilitating water reabsorption in response to ADH; renal concentrating ability may rise if urea production is increased when a high-protein diet is given.
‘Insensible’ water loss is proportionately much higher in infants than in adults because the ratio of surface area to body volume is high, more fluid is lost through the skin because the epidermis is not fully developed before about the 28th week of gestation, there is very little subcutaneous fat, and metabolic and respiratory rates are high. Insensible transepidermal water loss can increase by up to 50 per cent due to phototherapy or overhead baby warmers – hence the importance of maintaining high ambient humidity. Daily fluid requirements are therefore up to five times higher per kilogram of body weight than in adults. It is important to pay close attention to this, as high insensible losses can occur, leading to renal and circulatory failure and haemoconcentration and hypernatraemia. The average basal water loss in a neonate is about 20 mL/kg per day (see Chapter 2).
Sodium
The total body sodium content of a newborn infant of 1 kg is less than 100 mmol, compared with about 3000 mmol in the adult. A premature infant may need up to 6 mmol/kg of sodium a day because of the high losses, and a normal full-term infant about 2-4 mmol/kg, compared with about 1 mmol/kg in an adult. In preterm infants, plasma renin, aldosterone and angiotensin II concentrations are high.
Renal conservation of sodium is inefficient in premature infants, despite relatively high plasma renin activities and aldosterone concentrations compared with adults, because of immature renal tubular function. Excretion of a sodium load is impaired, perhaps because of the low GFR. Sodium balance should be carefully controlled, bearing in mind the very low total body sodium content at this age.
The total body sodium and the plasma sodium concentrations (and therefore osmolality) may fluctuate because renal function is immature. Sodium excretion declines after birth. Preterm babies may have a failure of sodium tubular reabsorption leading to sodium deficit, particularly if there is low sodium input. The newborn infant, unlike fully conscious children and adults, is unable to make its need for water clear and it may be difficult to assess the requirements accurately. Rapid changes in extracellular osmolality due to inappropriate intake of water cause significant shifts of water between the intracellular and extracellular compartments; signs varying from listlessness to convulsions, or even coma, may be caused by changes in cerebral hydration. The plasma sodium concentration should be monitored to ensure that the proportion of sodium to water is correct.
Hyponatraemia
The following are some of the causes of hyponatraemia:
Prolonged maternal infusion of oxytocin or other hypo-osmolal fluid, for example 5 per cent dextrose, during labour. Oxytocin has an antidiuretic action similar to that of ADH and may cause dilutional hyponatraemia in both mother and infant.
Inappropriate ADH secretion: following post-partum intracranial haemorrhage or meningitis in severe pulmonary disease, such as respiratory distress syndrome (RDS; also called hyaline membrane disease) or pneumonia.
Infusion of water in excess of sodium to the baby, either as 5 per cent dextrose or as hypo-osmolal sodium solutions.
Acute renal disease, including acute tubular necrosis, especially if hypo-osmolal fluid is given to a child with glomerular dysfunction.
Diuretic treatment, particularly in premature infants.
Congenital adrenal hyperplasia, adrenal insufficiency, hypothyroidism and cystic fibrosis should always be
considered as a possible cause of hyponatraemia at this age.
considered as a possible cause of hyponatraemia at this age.
Clinical signs of hyponatraemia may be associated with hypotension, drowsiness and convulsions.
Hypernatraemia
Hypernatraemia develops if water loss exceeds that of sodium, or if an excess of sodium relative to water is infused or fed.
If replacement is inadequate, the high ‘insensible’ water loss, aggravated by impaired urinary water retention, may cause rapid ECF depletion with hypotension and, if water depletion is predominant, hypernatraemia. This is particularly likely if ‘insensible’ loss is increased through the:
skin – for example due to sweating caused by pyrexia or overhead heaters or to phototherapy for jaundice,
lungs – if the infant is hyperventilating, for example because of pneumonia,
intestine – if there is vomiting or diarrhoea.
Iatrogenic causes in infants include giving sodium bicarbonate or excess salt. Neonates are more susceptible than adults to developing hypernatraemia because the addition of a given amount of extra sodium increases the very low extracellular sodium content proportionally more than if it were diluted in a larger pool, as it is in adults; this tendency is aggravated by impaired renal excretion. Nephrogenic diabetes insipidus is a rare cause of neonatal hypernatraemia.
Potassium
The total body potassium of a newborn infant of 1 kg is less than 100 mmol, compared with about 3000 mmol in an adult. A normal full-term infant requires about 2-4 mmol/kg of potassium a day (compared with about 1 mmol/kg in an adult) to replace losses.
As in the adult, artefactual causes of abnormal plasma potassium concentrations must be excluded. Pseudohyperkalaemia is especially likely if capillary samples are used, because tissue cells may be damaged if the skin is squeezed as in a heel prick. It may also be due to in vitro haemolysis, or to withdrawal of blood from a cannula through which a potassium solution is being infused. Conversely, pseudohypokalaemia may result if the infused fluid is potassium free.
Hypokalaemia
Hypokalaemia may be caused by increased gastrointestinal loss due to diarrhoea or an alkalosis, for example pyloric stenosis. In this age group also consider renal tubular acidosis (type I or II). Iatrogenic causes include diuretic use.
Hyperkalaemia
Iatrogenic causes include excess potassium treatment. Other common causes include acute kidney injury, exchange transfusion and tissue damage resulting from hypoxia or birth trauma, for example severe bruising or haematoma. Consider also congenital adrenal hyperplasia or adrenal insufficiency (see Chapter 5).
Pulmonary function
Perinatal asphyxia
Renal complications and disturbances of electrolyte balance are more likely to develop in infants with perinatal asphyxia. Cerebral oedema or haemorrhage may stimulate ADH secretion, causing oliguria and a dilutional hyponatraemia with hypo-osmolality, accompanied by a high urinary sodium concentration due to plasma volume expansion.
The hypotension occurring during asphyxia may reduce renal blood flow enough to cause acute oliguric renal failure (acute kidney injury). In addition to the oliguria and hyponatraemia, there may be uraemia and hyperkalaemia, with proteinuria. Hypoglycaemia and hypocalcaemia may also occur.
Fetal pH can be measured during delivery, usually from a capillary sample from the baby’s scalp. This may be indicated if changes in cardiotocograph or fetal heart rate indicate fetal distress. A pH value below 7.2 indicates the need for urgent delivery.
Hypoxaemia
Fetal lung fluid production ceases at birth, probably as a result of increases in adrenaline concentration during delivery. Labour squeezes liquid from the mouth, and absorption takes place into the pulmonary lymphatics and capillaries. Type 2 pneumocytes produce surfactant, which serves to reduce lung surface tension, thus facilitating lung expansion. Deficiency of surfactant as in prematurity can cause RDS.
Normal term neonatal arterial PO2 breathing air is about 8-11 kPa. Oxygen therapy can cause toxicity, including bronchopulmonary dysplasia and stiff lungs, and should be closely monitored, for example by transcutaneous gas analysis. Retinopathy of prematurity (previously known as retrolental fibroplasia) may lead to blindness.
Acid-base homeostasis
The plasma bicarbonate concentration is normally about 3 mmol/L lower in newborn infants than in adults. This is due partly to renal immaturity and partly to the low concentration of urinary buffers; both these factors impair bicarbonate ‘reabsorption’ and regeneration. However, the plasma concentrations must be interpreted with caution, as they are likely to be artefactually low in very small samples. The neonate is more vulnerable to acid-base disturbances (for further discussion see Chapter 4). The specific conditions that affect neonates are discussed here.
Metabolic acidosis
The causes of metabolic acidosis include the following:
renal dysfunction,
lactic acidosis due to:
tissue hypoxia resulting from poor tissue perfusion caused by hypotension and the low PO2 accompanying asphyxia or sepsis,
some inborn errors of metabolism, such as glucose-6-phosphatase deficiency,
inborn errors of amino acid or organic acid metabolism (see Chapter 27).
Also consider:
congenital heart disease and patent ductus arteriosus,
acute blood loss.
Metabolic alkalosis
This can result from pyloric stenosis, with which projectile vomiting can occur. This is associated with hypokalaemic alkalosis.
Respiratory acidosis
Respiratory distress may be caused by pulmonary disorders such as RDS, pneumonia and meconium aspiration during birth. The most common cause in the preterm infant is RDS, the incidence of which is inversely related to the gestational age of the infant at birth. Respiratory distress syndrome is due to immaturity of the enzymes responsible for the intrauterine synthesis of pulmonary surfactant, which maintains the patency of the alveoli. Surfactant synthesis begins at about the 20th week of gestation and increases rapidly after about the 34th week following maturation of the alveolar cells. Surfactant deficiency is more common in male infants, those of diabetic mothers and those with asphyxia and hypothermia.
The condition presents with pulmonary collapse (atelectasis), with secondary lung infections being common. The blood PCO2 is high, causing respiratory acidosis. The low blood PO2 and reduced blood flow due to hypotension cause tissue hypoxia with lactic acidosis; renal dysfunction may aggravate the metabolic acidosis. The combination of respiratory and metabolic components may cause severe acidosis.
An infant with RDS may benefit from the administration of surfactant, given through an endotracheal tube, and from positive-pressure ventilation, which help to expand the lungs and correct blood gas abnormalities. If these treatments are successful, the improved general condition increases tissue perfusion, correcting the lactic acidosis and improving renal function.
The PO2 and PCO2 of cutaneous capillary blood can be monitored continuously using electrodes placed on the skin. The electrodes bring the capillary PO2 and PCO2 to near arterial levels by heating the skin to about 44°C, thus increasing cutaneous blood flow. The electrodes must be repositioned every 4 h to prevent local burns and need to be recalibrated frequently. Transcutaneous blood gas monitoring supplements, but does not replace, arterial blood gas analysis (see Chapter 4).
Respiratory alkalosis
The conditions associated with a respiratory alkalosis that occur in neonates include septicaemia, meningitis, hyperammonaemia and hepatic failure.
Gastrointestinal tract
The fetal gastrointestinal tract has limited functional development before 26 weeks. This helps to explain why premature babies may have poor tolerance of enteral feeding. Lactose may be poorly absorbed within the first week of life.
Liver
The metabolism of the neonatal liver is initially slower than in adults. As will now be seen, this is important with regard to neonatal bilirubin metabolism, particularly that of conjugation.
Plasma transaminase activities are up to twice the upper limit of the adult reference range during the first 3 months of life and fall to adult levels by the age of about 1 year. The plasma total alkaline phosphatase activity is higher in infancy and during childhood because of the contribution from actively growing bone; it falls to adult levels after the pubertal growth spurt.
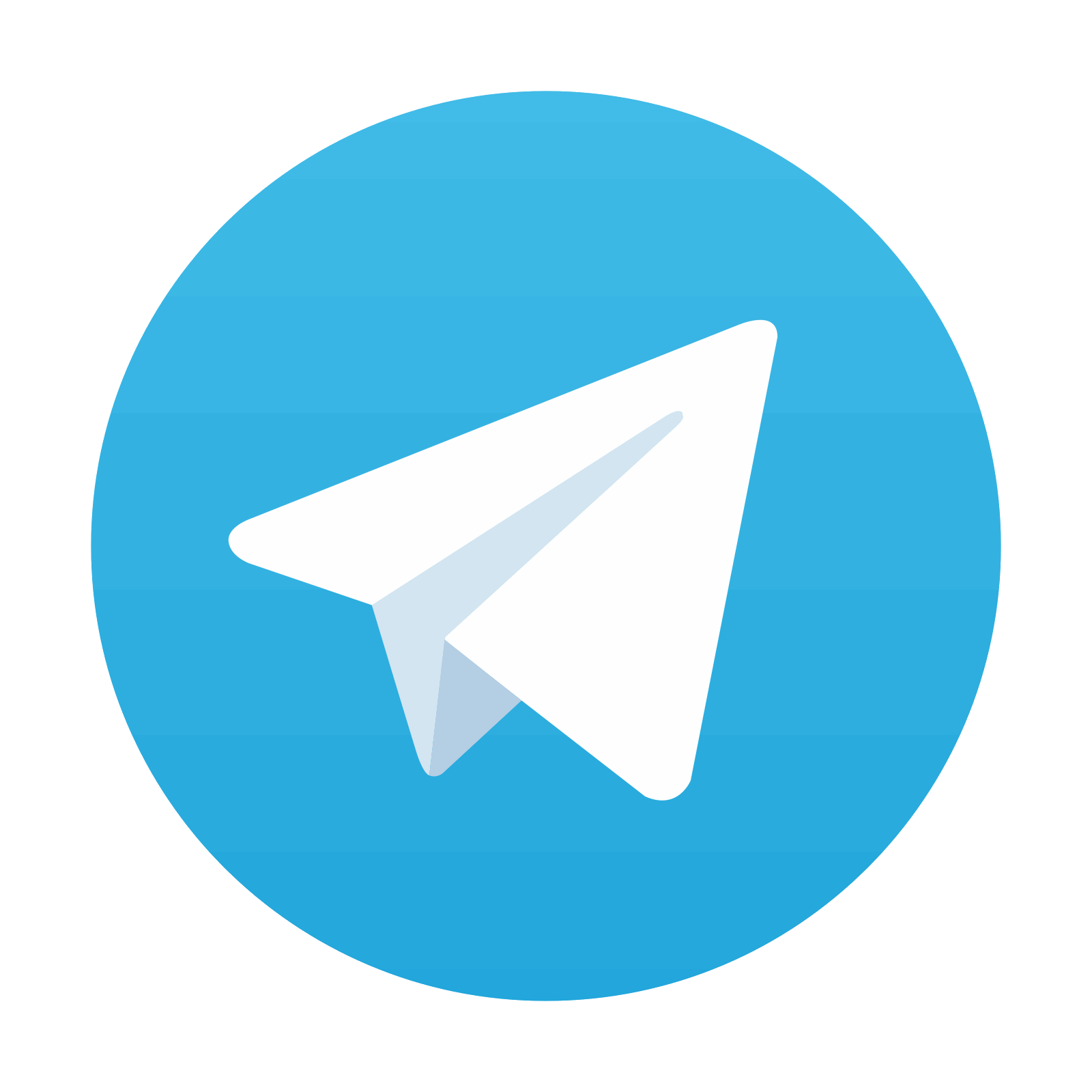
Stay updated, free articles. Join our Telegram channel

Full access? Get Clinical Tree
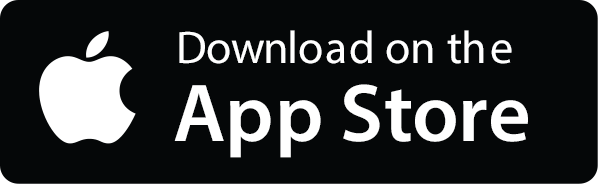
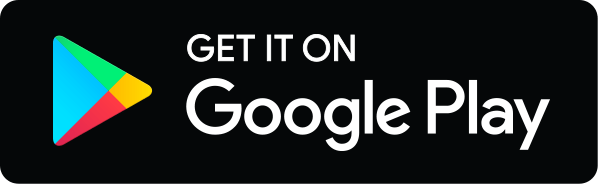