Chlorine Dioxide
Zhao Chen
Mark A. Czarneski

Chlorine dioxide is a yellow-green gas that was first prepared by Chenevix in 1802.1 Humphrey Davy independently prepared this compound in 1811, elucidated its composition, and proposed the name of euchlorine.2 Although chlorine dioxide is the most widely accepted English name for this compound, the names chlorine oxide, anthium dioxide, chlorine(IV) oxide, chlorine peroxide, chloroperoxyl, and chloryl radical also have been used. The Chemical Abstracts Service Compound Registry Number (CAS RN) for chlorine dioxide is 10049-04-4.
Structural Properties
Chlorine dioxide contains one atom of chlorine and two atoms of oxygen and exists entirely or almost entirely as a free radical monomer (Figure 27.1).3,4 Microwave spectra of chlorine dioxide in the gaseous phase have given chlorine-oxygen distances of about 0.147 nm; electronic diffraction indicates 0.149 nm. This chlorine-oxygen distance is approximately that of an average chlorine-oxygen double bond. The angle formed by the oxygen-chlorineoxygen bonds is in the range of 117.7 + 1.7 degrees.4,5 Chlorine dioxide has considerable unsaturated bond character,6 but, in solution, there is no evidence of dimerization or polymerization,7 and at neutral pH, it does not hydrolyze.8 Crystalline hydrates of chlorine dioxide have been reported, including a hexahydrate, an octahydrate, and a decahydrate.4,9,10,11
Chlorine dioxide is one member of a series of oxides that also includes chlorine monoxide (Cl2O); chlorine peroxide [Cl(O2)], which has the same molecular formula as chlorine dioxide but a different structure; chlorine trioxide (ClO3); chlorine tetroxide (ClO4); chlorine heptoxide (Cl2O7); as well as dimers and mixtures of these oxides. Although all are sometimes called chlorine oxide, each has distinctly different properties from chlorine dioxide.
Spectral Properties
The ultraviolet adsorption spectrum of chlorine dioxide dissolved in carbon tetrachloride shows maxima at 375 and 355 nm with a minimum at 263 nm.8 The absorption spectrum in aqueous solution has a broad band near 360 nm.4 The molar extinction coefficient is frequently reported as 1150 M/cm-1,12 but when using high-resolution, narrow bandwidth spectrophotometers, it has been determined to be 1250 M/cm-1.11,13 The extinction coefficient is temperature, acid, and ionic strength independent from 25°C to 50°C, 0.2 to 4 N, and 2 to 4 M, respectively,4,11 and is unaffected by chloride concentration up to 0.3 M.11,14 The chlorine dioxide gas-phase spectrum is the same as that in aqueous solution,4,11 providing a convenient method for monitoring concentration in various processes.
In the infrared, the fundamental vibrational frequencies of chlorine dioxide in the gaseous phase, measured as wave numbers, are 946 cm-1, 448 cm-1, and 1110 cm-1, corresponding to the symmetric stretch, bend, and asymmetric stretch, respectively.5
Physical Properties
Under standard pressure, chlorine dioxide freezes at a temperature of -59°C and boils at 11°C.15 Thus, it is a true gas at room temperature, where its density is about 1.6 g/cm3.16 The gas has an odor similar to that of chlorine.
Chlorine dioxide is soluble in water, and a formula has been developed to predict solubility under various conditions.12,17 Solubilities at 0°C, 15°C, and 30°C were demonstrated to be linear,15 and extrapolated solubility
curves have been published.8 At 20°C, chlorine dioxide gas present at a concentration of 4% by volume has a solubility of about 4 g/L. The partition coefficient of chlorine dioxide between water and the gaseous phase is expressed as:
curves have been published.8 At 20°C, chlorine dioxide gas present at a concentration of 4% by volume has a solubility of about 4 g/L. The partition coefficient of chlorine dioxide between water and the gaseous phase is expressed as:

and is equal to 70 ± 0.7 at 0°C, 45 at 15°C, and 26.5 + 0.8 at 35°C.8
Chlorine dioxide in the gas phase is stable in concentrations of less than 10% in air at atmospheric pressure. The gas tends to be unstable at higher concentrations and can be destabilized further by contact with light or with substances that catalyze its decomposition. When decomposition occurs, the volume increase is relatively small, and the resulting explosion has sometimes been described as a “puff.” Using chlorine dioxide in gas form for area disinfection/sterilization, the concentrations are typically 0.04% to 0.18% (1-5 mg/L). These concentrations are far below the “puffing” threshold for chlorine dioxide. Detonation has never been observed, even at much higher concentrations at temperatures less than 42°C.18 Attempts to store chlorine dioxide in a compressed form, with or without other gases, have been unsuccessful12 until recently when CDG Environmental obtained US Department of Transportation permission to ship 3000 ppm solutions (CDG Solution 3000 liquid concentrate). Recent studies have confirmed the lower limit for explosive decomposition at 9.5% (chlorine dioxide/air).19 Thus, when the concentration of chlorine dioxide gas in air is below 9.5%, there is no explosion hazard.

Because chlorine dioxide is not sufficiently stable to be stored, it is typically produced at the site of use. Many methods exist for the preparation of chlorine dioxide, and the method chosen for a specific application will depend on the amount required, the amount of side products that can be tolerated, and whether the gas is required in solution or the gaseous form. The large-scale commercial production of chlorine dioxide, as is required in the bleaching of paper, generally involves the reduction of sodium chlorate by a suitable acid.4 Several alternative processes use this method, and the details can be found in the extensive compilation of Masschelein and Rice.8
Sterilization and disinfection applications are smaller scale processes in which production quantities do not exceed 2000 kg/d. For such processes, sodium chlorite is the preferred starting material.11 In potable watertreatment facilities, all the chlorine dioxide used is generated from sodium chlorite.12 As pointed out by Gordon et al,4 the advantages of using chlorite in the generation of chlorine dioxide are its ease of use and the purity of chlorine dioxide that is produced. In some methods, acid is added to the chlorite/hypochlorite mixture.20 Chlorine dioxide also has been generated by oxidation of chlorite with nitrogen trichloride.4 Today, most small generators use an oxidative process in which chlorine (either as a gas or in solution) is mixed with sodium chlorite solution (either as a solid or in solution).12 This reaction is rapid, readily goes to completion, and proceeds with following stoichiometry:
2NaClO2 + Cl2 → 2ClO2 + 2NaCl
A convenient application of this method of production consists of passing diluted chlorine gas through a column or tower of sodium chlorite to yield uncontaminated chlorine dioxide.21 Chlorine dioxide generated by this method can be used immediately or dissolved in a solvent (typically water) for temporary storage. This method also can be used to remove traces of chlorine from a stream of chlorine dioxide gas prepared using another method.4
Numerous alternative methods for generating chlorine dioxide for smaller scale applications have been reported. Some examples include the electrolysis of chlorite solutions,4,8 by passing nitrogen dioxide, obtained from the effluent gasses of an electric arc machine, through a column of sodium chlorite.22 For the preparation of small quantities of chlorine-free chlorine dioxide, the oxidation of chlorite with persulfate, as illustrated by the following reaction, has been used4,8:
2NaClO2 + Na2S2O8 → 2ClO2 + 2Na2SO4

Chlorine dioxide is a strong oxidizing agent and, in contrast to chlorine, does not tend to react with organic materials to form chlorinated species or with ammonia to form chloramine. The inorganic chemistry and reactions of chlorine dioxide with organic matter have been well studied and can be found in the comprehensive references written by Gordon et al,4 Masschelein and Rice,8 and Aieta and Roberts.23
Decomposition
As a free radical species, chlorine dioxide is not stable for long periods in storage and can decompose. Whereas the
gas can be stable in concentrations of less than 10% by volume in air, decomposition is facilitated at higher concentrations. Although its instability precludes storage of chlorine dioxide in compressed form,12 it is sufficiently stable to permit its routine use, and studies have been done to elucidate the factors that influence its stability.
gas can be stable in concentrations of less than 10% by volume in air, decomposition is facilitated at higher concentrations. Although its instability precludes storage of chlorine dioxide in compressed form,12 it is sufficiently stable to permit its routine use, and studies have been done to elucidate the factors that influence its stability.
The decomposition reaction of high concentration of chlorine dioxide has been studied in depth.24,25,26,27 For concentrated chlorine dioxide gas (15%-30% by volume), the ignition temperatures are approximately 130°C; the presence of light, dust, petroleum-based lubricants, and sulfur all lower the decomposition temperature.28 Further, chlorine dioxide can undergo autocatalytic decomposition, which may or may not involve explosion, and the ratios of products formed during decomposition depend on the concentration of water vapor and temperature.24 Surface area can accelerate the decomposition of chlorine dioxide, but sufficiently large surface areas appear to inhibit catalytic decomposition by adsorption of the intermediates.
Exposure to light leads to decomposition of chlorine dioxide,8,25 and in the gas phase, the primary photochemical reaction is the homolytic fission of the chlorine-oxygen bond to form ClO • and O •.8 The reaction mechanism for the light catalyzed decomposition of gaseous, dry chlorine dioxide is postulated as:
ClO2 + hv → ClO• + O•
ClO2 + O• → ClO3
2ClO• → Cl2 + O2
Interestingly, when moisture is present along with gaseous chlorine dioxide, exposure to light may induce the formation of a visible mist that does not contain chlorine but rather consists of a complex mixture of acids.25 The following mechanism has been proposed for the photolytic decomposition of chlorine dioxide in the presence of moisture7:
ClO2 + hv → ClO• + O•
ClO2 + O• → ClO3
2ClO3 → Cl2O6
ClO• + ClO2 → Cl2O3
Cl2O6 + H2O → HClO3 + HClO4
Cl2O3 + H2O → 2 HClO2
2 HClO2 → HClO + HClO3
In solution at neutral pH, in the absence of light, and at room temperatures (18°C-25°C) or cooler, chlorine dioxide is quite stable. The primary decomposition process, when it occurs, is hydrolysis and disproportionation of chlorine dioxide into chlorite and chlorate ions:
2ClO2 + 2OH– → ClO2– + ClO3– + H2O
The rate of hydrolysis is impacted by temperature and pH, increasing rapidly at elevated temperature and at pH values above 10, and is more rapid in the presence of chlorine and hypochlorite, producing chlorate and hydrochloric acid.11
In reducing environments, chlorine dioxide may undergo single electron transfer processes, which ultimately result in the formation of chloride. The standard potential (E°) for chlorine dioxide is 1.511 V,29 although in solution, the electron potential is dependent on the pH and the number of electrons transferred.
Reactions With Organic Compounds
Chlorine dioxide is a selective yet versatile oxidant for many organic compounds. It does not act via chlorination, and thus, trihalomethane formation does not occur.12 The chemistry and mechanisms of reactions of chlorine dioxide with organic compounds have been reviewed extensively,8,30,31 and a compilation of kinetic data is also available.32 The oxidation of amines with chlorine dioxide was studied in detail by Rosenblatt et al33,34 and Hull et al35,36 who found that, in aqueous solutions, primary and secondary amines react slowly or not at all with chlorine dioxide but that tertiary amines were readily oxidized, producing a secondary amine and an aldehyde.
Chlorine dioxide does not react with saturated aliphatic hydrocarbons, whereas alcohols, aldehydes, and ketones are oxidized to form carboxylic acids.11 Chlorine dioxide reacts with carbohydrates, such as glucose, to oxidize the primary hydroxyl groups, first to aldehydes and then to carboxylic acids.8 The reaction with lipids is mainly an oxidation at the double bond. Although most amino acids do not react readily with chlorine dioxide, tyrosine, tryptophan, and cysteine are exceptions.31,37 Peptides and proteins are subject to oxidation, substitution, and addition reactions.38 Chlorine dioxide solutions have been shown to denature proteins,39 and the gas phase has been shown as an effective way of inactivating β-lactams from old production facilities.40 Additionally, chlorine dioxide can cause destruction of lysozyme function via protein denaturation and degradation.41
Chlorine dioxide rapidly oxidizes phenolic compounds42 and has been used to oxidize chlorinated phenolic compounds to reduce their toxicity.11 Occasional chlorination of aromatic or unsaturated aliphatic hydrocarbons has been reported,43 but no trihalomethanes were formed. Because chlorine dioxide does not tend to form dioxins or trihalomethanes or react with ammonia to form chloramines, it has great appeal for the treatment of water and wastewater.

The antimicrobial properties of chlorine dioxide solutions were documented in the 1930s by Schaufler44 and Kovtunovitch and Chemaya.45 Later, Ridenour and Ingols46 reported on the addition of chlorine dioxide along with chlorine to commercial water supplies to produce better tasting water, a practice that continues today. Numerous studies have been carried out since then to validate the biocidal properties of chlorine dioxide in an aqueous environment and have demonstrated broad-spectrum biocidal properties. Chlorine dioxide has several registered uses in water treatment, disinfection, and sterilization in both dissolved in water and applied as a gas.
Biocidal Activities
Studies have proved the efficacy of aqueous and gaseous chlorine dioxide on a wide variety of microorganisms, including bacteria, fungi, spores, viruses, and protozoa. The effect of chlorine dioxide has been proved in laboratory conditions on major bacterial pathogens responsible for principal outbreaks, such as Escherichia coli O157:H7, Listeria monocytogenes, and Salmonella enterica.47 Meanwhile, some early studies have shown that chlorine dioxide is capable of inactivating enteroviruses, polioviruses, rotavirus, and human immunodeficiency virus (HIV).48,49,50,51,52,53,54,55 The increasing interest in the potential biocidal uses of chlorine dioxide has also stimulated studies into the activity of this agent against various protozoal, fungal, and algal species, such as Cryptosporidium parvum oocysts, Streptomyces griseus, and yeasts.56,57,58,59
With the current concerns about prions and prionassociated diseases, it is not surprising that studies into the inactivation of these agents by chlorine dioxide have been undertaken. Brown et al60,61,62 studied the inactivation of prions by chlorine dioxide and other disinfectants. Sodium hypochlorite produced consistently marked inactivation (3-4 log within 15 minutes), whereas chlorine dioxide (50 ppm) exhibited moderate to substantial inactivation. It was suggested that doubling or tripling the concentration of chlorine dioxide would be more effective in inactivating the agent of Creutzfeldt-Jakob disease.
Microorganisms differ greatly in the sensitivity to chlorine dioxide (Figure 27.2).47 One of the most distinct features of some bacteria is the ability to produce stress-resistant spores. Because of sporulation, resistance to heat, radiation, desiccation, extreme pH, chemicals, enzymes, and high pressure are largely increased.63 This resistance enables the bacteria to survive many antisepsis and disinfection (eg, pasteurization) processes. As noted in the previous sections, the broad-spectrum activity of chlorine dioxide in solution was well established in the 1940s and the years following; however, the sporicidal activity of gaseous chlorine dioxide and, thus, its efficacy as a gas sterilant were not demonstrated until the 1980s. The lethal activity of chlorine dioxide gas on spores of Bacillus subtilis was first reported by Rosenblatt et al64 in their patent of the use of chlorine dioxide as a gas sterilant. Sporicidal activity was present at concentrations as low as 11 mg/L. In a work reported subsequently, it was found that, analogous to ethylene oxide, adequate hydration of the spores was required for optimal activity.65 Activity was also demonstrated against the anaerobic spore former Clostridium sporogenes.66 In 1988, chlorine dioxide gas was registered as a sterilizing agent by the US Environmental Protection Agency (EPA) under the Federal Insecticide, Fungicide, and Rodenticide Act (FIFRA). Work by Jeng and Woodworth67 confirmed the sporicidal activity of chlorine dioxide and provided further information about the conditions required for its effective use.
Biofilm can be defined as a community of microorganisms, generally associated with or attached to abiotic or biological surfaces (see chapter 67).69 These cells are frequently embedded in exopolymeric substances. The tolerance of vegetative bacterial cells in biofilms to environmental stresses, such as routinely used disinfectants, can be profoundly increased than planktonic bacteria. The exopolysaccharide (EPS) matrix restricts the access of antimicrobial substances to cells within the biofilm by reducing diffusion and by maintaining cells at slow growth rate. For example, the survival of Pseudomonas fluorescens M2 cells after exposure to a chlorine dioxide solution was apparently enhanced by the presence of P fluorescens DL5 in binary biofilms.70 Vaid et al71 reported that a 10-minute treatment of 0.3 mg/L gaseous chlorine dioxide and 7 mg/L of aqueous chlorine dioxide resulted in reductions of 3.21 and 3.74 log CFUs/cm2 of L monocytogenes within a biofilm matrix on stainless steel coupon, respectively.
Interestingly, Martin et al72 isolated a B subtilis strain from a washer-disinfector whose vegetative form demonstrated unique resistance to 0.03% chlorine dioxide. It has been postulated that additional efficient intracellular mechanisms may be involved to explain its significant resistance to in-use concentrations of commonly used highlevel disinfectants.
The effectiveness of chlorine dioxide on inactivating microorganisms also largely depends on treatment conditions, such as chlorine dioxide phase, concentration, contact time, temperature, pH, total suspended solids, and organic matter.73 The efficacy increases at higher concentrations and treatment times but decreases with a decrease in temperature and pH. Concerning total suspended solids, their presence promotes pathogen aggregation and interferes with the disinfection performance of chlorine dioxide.74 Nonetheless, when compared to chlorine, chlorine dioxide is a more effective biocide in the presence of high levels of organic matter, depending on the concentration.
Another factor associated with treatment conditions is the phase of chlorine dioxide. Studies have demonstrated that the application of chlorine dioxide in a gaseous form is more effective in inactivating microorganisms compared with its aqueous form, presumably due to its higher penetrability.47
Biocidal Mechanism
The possible modes of action of chlorine dioxide over microorganisms have been widely studied (see Figure 27.2). Research into the mechanism of the biocidal action of chlorine dioxide has involved the identification of specific chemical reactions between chlorine dioxide and biomolecules and evaluation of the effect of chlorine dioxide on physiological functions. The most widely reported mechanism is the disruption of cell protein synthesis primarily due to the oxidations of cell surface membrane proteins12,75 and free fatty acids76 and the increase in the permeability of the microbial cell.77 Cho et al78 reported that chlorine dioxide caused some levels of both surface damage and inner component degradation in E coli. Furthermore, Cho et al78 explained that only after a certain level of surface damage, intracellular components were attacked because the initial lag phase in enzyme degradation was noticeable and was related to the time required for chlorine dioxide to penetrate the cell.
Chlorine dioxide has also been related to membrane damage on bacterial spores.79,80 Chlorine dioxide caused damage to the inner cell membrane, change in cell permeability, and interruption of the complete germination of B subtilis spores.79 In Bacillus cereus, chlorine dioxide caused surface roughness, indentations, and elongation of cells that resulted in the inhibition of division and associated metabolic damage of bacterial cells.79 With respect to viruses, chlorine dioxide inactivated poliovirus by altering viral capsid proteins and reacting with RNA separated from the capsids, which impaired RNA synthesis.48 Li et al81 reported that the inactivation mechanism of hepatitis A virus (HAV) by chlorine dioxide was due to the loss of the 5’nontranslated regions (5’NTR) (the sequence from bp 1 to 671) and/or destruction of the antigenicity, which is different from that of chlorine.
However, most of the published studies have been focused on the inactivation mechanisms of chlorine dioxide on bacteria and viruses. And there are very few studies on the fungicidal mechanisms of chlorine dioxide. Zhu et al82 has showed that the metal ion leakage, the inhibition of enzyme activities, and the alteration of cell structure were critical events in Saccharomyces cerevisiae inactivation by chlorine dioxide.

Chlorine dioxide is used in large quantities, and experience has demonstrated that it can be used safely. Because it is a chemical disinfectant and sterilizing agent, however, it is toxic to living systems. Its application, therefore, should be carefully managed, and steps should be taken to prevent unacceptable exposure. Smith and Willhite83 indicated that although there are concerns about chlorine dioxide’s acute toxicity, they concluded that the human experience with chlorine dioxide in both prospective studies and in actual use situations has failed to reveal adverse long-term health effects.
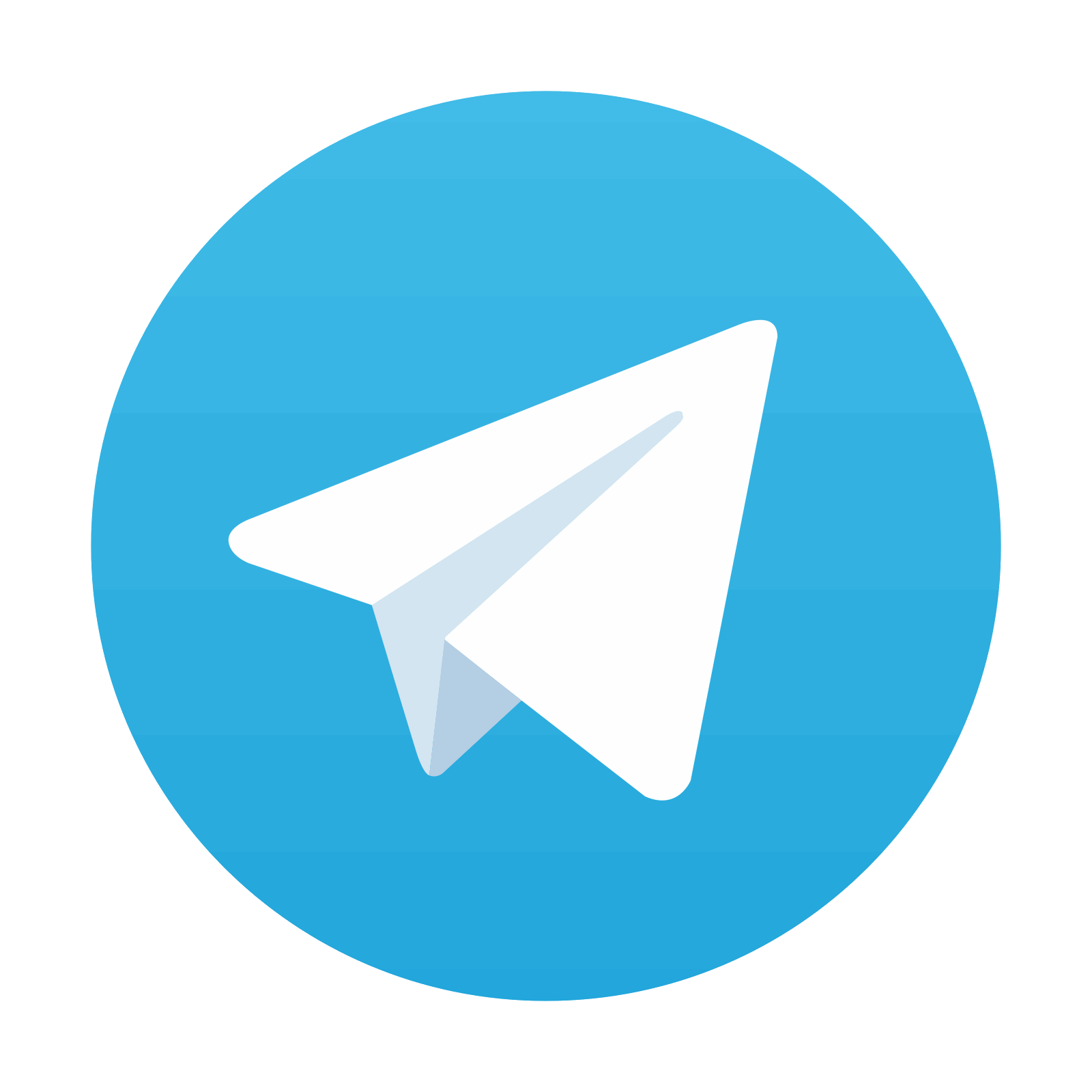
Stay updated, free articles. Join our Telegram channel

Full access? Get Clinical Tree
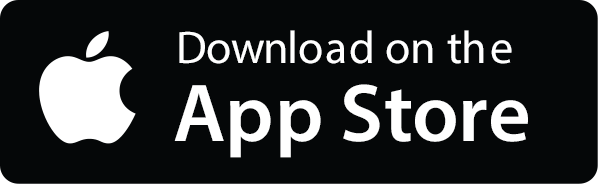
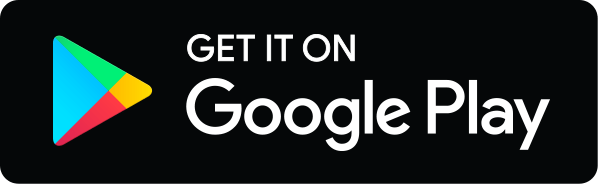