9 Zhong-Dong Shi Federico González, and Danwei Huangfu Developmental Biology Program, Memorial Sloan-Kettering Cancer Center, USA Human embryonic stem cells (hESCs) are derived from the inner cell mass (ICM) of cultured pre-implantation human blastocysts [1]. With their ability to proliferate indefinitely in vitro (self-renew), while maintaining the potential to differentiate into virtually any cell type of the human body (pluripotency), they provide an unlimited source of cells suitable for regenerative medicine, drug discovery, and the study of human development or disease [2]. The major limitation of hESCs for cell therapy resides in their origin. In addition to ethical controversies, transplantation of hESC-derived cells is complicated by potential immune responses to allogeneic tissues [3]. To ensure the histocompatibility needed for transplantation, it would be ideal to use pluripotent stem cells derived from the patient. To accomplish this goal, somatic-cell nuclear transfer (SCNT) represents an attractive option. More than 3 decades separate the first tadpoles cloned by John Gurdon [4] from Dolly the Sheep, the first cloned mammal [5]. Since then, SCNT has allowed the generation of viable adult clones in many mammalian species [6–13] and permitted the creation of pluripotent stem cells from mouse [14], bovine [15], and rhesus macaque [16]. These successes suggest that the same approach could apply to humans. However, SCNT has yet to succeed in our species, due to the limited availability of human oocytes for research and potentially reflecting inherent barriers of the human model [17]. These problems have prevented the success of human therapeutic cloning up to now. SCNT [4] and somatic-cell fusion experiments with germ cells [18] or embryonic stem cells (ESCs) [19,20] demonstrate that the somatic genome is amenable to reprogramming, and suggest that pluripotency can be restored in a terminally differentiated cell by factors present in oocytes or pluripotent stem cells. This concept led to the groundbreaking experiment by Yamanaka and colleagues, who managed to induce pluripotency in differentiated mouse and human adult fibroblasts by ectopically expressing four pluripotency-associated transcription factors: Oct4, Sox2, Klf4, and c-Myc [21,22]. This process, referred to as reprogramming, generates induced pluripotent stem cells (iPSC) that closely resemble ESCs in global gene-expression profile, epigenetic signature, and developmental potential [23–29]. Reprogramming represents a simple way of generating patient-specific human pluripotent stem cells (hPSCs) useful for a wide range of applications, including autologous cell-transplantation therapy, modeling of both monogenic and polygenic diseases, and provision of relevant cell types for drug toxicity, differentiation, and therapeutic screens. These applications require efficient and reliable reprogramming methods, which are being optimized [30]. In most cases, the generation of human induced pluripotent stem cells (hiPSC) involves ectopic expression of a set of core pluripotency-related transcription factors (Oct4, Sox2, Klf4, and c-Myc, or other combinations) in adult fibroblasts over a course of ∼2–4 weeks [22,27]. Reprogramming of human fibroblasts is a slow and inefficient process: less than 0.01% of donor cells become iPSCs in initial studies [31]. Hence, recent years have seen cohorts of publications aiming to improve the original protocol by using alternative donor cells [32–34] or identifying novel reprogramming factors [35–37]. So far, the most efficient protocols make use of constitutive- [22,27], or inducible- [38] integrative viral vectors to deliver the reprogramming factors. However, these approaches generate genetically and phenotypically heterogeneous cell lines, limiting their use for therapy due to concerns over tumorigenicity caused by potential insertional mutagenesis or reexpression of the reprogramming factors [25,39]. Various approaches have been developed to avoid potentially detrimental genetic modifications, using alternative methods to deliver reprogramming factors, such as integration-defective viruses [40–42], episomes [43–46], transposons [47,48], cell-permeable recombinant proteins [49,50], RNAs [51], and microRNAs [52,53], often combined with treatment with chemical compounds that induce or facilitate reprogramming [54,55]. Beside this increasing number of alternative approaches, a mechanistic dissection of the process may reveal cell-intrinsic and -extrinsic signals governing reprogramming and enable the design of rational approaches to improve it. In this regard, small molecules provide a powerful tool by which to address these issues. First, their biological effects are usually rapid, reversible, and dose-dependent, allowing a precise control of specific outcomes through modulation of their concentrations and combinations. Second, the structural diversity provided by synthetic chemistry facilitates functional optimization of compounds of interest. Third, compared to any genetic delivery method, the delivery of chemicals is both convenient and efficient. Using both phenotypic screening and hypothesis-driven approaches, many compounds have been identified that can functionally replace reprogramming transcription factors and enhance the efficiency and/or the kinetics of reprogramming by modulating the activity of epigenetic modifiers or signaling pathways controlling pluripotency or cell proliferation [56]. This chapter reviews recent findings that use chemical compounds to improve reprogramming. These chemical compounds and their roles in reprogramming are also summarized in Table 9.1. Table 9.1 Chemical compounds facilitating somatic cell reprogramming to pluripotency Improves OSK-induced reprogramming and induces iPSC generation from OS-infected human fibroblasts [54,64] Dramatically increases reprogramming efficiency of MEFs transduced with OSKM under hypoxic condition [63] Promotes complete reprogramming and facilitates expansion of fully reprogrammed iPSCs by inhibiting growth of nonreprogrammed cells; generated iPSCs can produce adult mice [48,66] Enables reprogramming of MEFs to iPSCs using recombinant proteins of four or three Yamanaka factors (OSKM or OSK) [50] Enables generation of iPSCs from MEFs by only expressing miR302/367 [52] Enhances efficiency of iPSC generation from human adult and fetal fibroblasts by retroviral delivery of four or three Yamanaka factors (OSKM, OSK, OSM) [65] Significantly improve reprogramming from MSCs established from a patient with sickle-cell disease using a piggyback transposon vector that contains five transgenes (OSKM + Lin28) [65] Enhances reprogramming efficiency of mouse NSCs infected with OK [55] Increases reprogramming using OK in MEFs when combined with a DNMT inhibitor, RG108, and L-calcium channel agonist, BayK8644 [69] Enables reprogramming of mouse NSCs with SKM with a very low efficiency [55] Enables reprogramming of human keratinocytes infected by KO when combined with CHIR99021 [74] Improves reprogramming of MEFs infected by Oct4 in the presence of VPA, CHIR99021, and E-616452 [75] Increases the reprogramming efficiency of OSKM- or OSK-infected MEFs [54] Promotes partially reprogrammed MEFs to fully reprogrammed iPSCs [24] Enhances reprogramming efficiency of MEFs transduced with OK when combined with BIX [69] Increases reprogramming efficiency of human fibroblasts transduced with OSKM [65] Replaces Sox2 during reprogramming of MEFs [81] Increases human fibroblast reprogramming with OSKM when combined with PD0325901 [86] Enhances human keratinocyte reprogramming when combined with PD0325901 [106] Improves ultimate reprogramming and maintenance of iPSCs generated from rat-liver epithelial cells transduced with OSK when combined with PD0325901 and CHIR99021 [85] Enables reprogramming of MEFs transduced with Oct4 alone when combined with a protein arginine methyltransferase inhibitor, AMI-5; the iPSCs can give rise to liveborn pups through tetraploid complementation [87] Promotes “pre-iPSCs” into fully reprogrammed cells under LIF treatment when combined with PD0325901 [97] Enables reprogramming of MEFs transduced with OK [74] Enables reprogramming of human keratinocytes transduced with OK when combined with Parnate [74] Promotes transition to mature iPSCs [55,97] Enhances hiPSCs generation when combined with TGFβ inhibitors [86] Improves reprogramming efficiency of mouse and human fibroblasts [76,110] Improves quality of iPSCs reprogrammed from mouse fibroblasts and B lymphocytes, allowing generation of all-iPSC mice [122] O, Oct4; K, Klf4; S, Sox2; M, c-Myc. Reprogramming involves the genome-wide reshuffling of the transcriptome and chromatin statuses of a somatic cell to that of a pluripotent cell. At the epigenetic level, this event is reflected in the dynamic and opposite remodeling of histone marks and DNA methylation patterns present in the regulatory regions of somatic- and pluripotent-specific genes [57]. Under physiological conditions, the capacity of transcription factors to bind their target sequences is modulated by the accessibility of DNA, resulting from chromatin organization. The nucleosome, the basic subunit of eukaryotic chromatin, is composed of two molecules of each of the core histone proteins H2A, H2B, H3, and H4, around which DNA is wrapped. DNA accessibility is regulated at different levels by the deposition of specific histone marks on nucleosomes, the inclusion of different histone variants, the activity of ATP-dependent chromatin remodeling factors, and DNA methylation status [58]. A major mechanism controlling chromatin organization is the covalent modification of histones, the building blocks of nucleosomes. Specific enzymes such as histone acetyltransferases, histone deacetylases (HDACs), histone methyltransferases, and histone kinases can target different residues of histones and modulate their function. At the DNA level, the methylation of CpG islands found in the promoter regions of many genes has profound effects on their transcriptional status. Thus, chromatin-modifying enzymes play critical roles in both reactivating silenced loci and reinstating the closed domains of heterochromatin during the global epigenetic remodeling of differentiated cells to pluripotency, suggesting that these enzymes may act as facilitators or barriers to cell-fate transitions [59]. Therefore, by modulating these specific epigenetic barriers, one may promote reprogramming efficiency or even reduce the number of reprogramming factors. For example, inhibition of the H3K79 histone methyltransferase Dot1L by gene knockdown can accelerate reprogramming, significantly increase the yield of iPSC colonies, and substitute for Klf4 and c-Myc [59]. In principle, small molecules specifically modulating these different aspects of chromatin and DNA structure may also have significant effects on reprogramming. Indeed, a number of studies have shown that inhibitors of HDACs, histone methyltransferases, and histone demethylases or inhibitors of DNA methyltransferases can increase reprogramming efficiency and/or replace the activity of certain reprogramming factors [54,60]. Although HDAC inhibitors have shown only a modest effect on the success rate of SCNT (two- to fivefold) [61,62], they have proved to significantly increase the efficiency of iPSC generation. Using mouse embryonic fibroblasts (MEFs) derived from Oct4-GFP reporter mice, Huangfu et al. [54] first showed that three HDAC inhibitors, valproic acid (VPA), trichostatin A (TSA), and suberoylanilide hydroxamic acid (SAHA) greatly improve reprogramming efficiency. Of the three, VPA exerts the most significant effect. VPA (2 mM) induced >10% Oct4-GFP+ cells in MEFs infected with four-factor (Oct4, Sox2, Klf4, and c-Myc) in 1 week, which amounts to >100-fold improvement compared to the control. Furthermore, VPA promoted reprogramming efficiency in a dose-dependent manner and its EC50 was ∼1.9 mM [54]. VPA has a synergistic effect with hypoxia when reprogramming. Yoshida et al. reported that hypoxia and VPA alone could enhance iPSC generation in MEFs transduced with four factors by ∼40-fold and ∼50-fold respectively, while a combination of VPA and hypoxia could increase the reprogramming efficiency more than 200-fold [63]. Notably, VPA can also increase the percentage of Oct4-GFP+ cells by 50 times when using only three factors (Oct4, Sox2, and Klf4), which is still much higher than the four-factor reprogramming without chemical treatment [54]. Further study has shown that VPA can also improve both four- and three-factor reprogramming efficiency by 10–20-fold when used in primary human fibroblasts [64,65]. Importantly, VPA enables reprogramming of human fibroblasts with only two factors, Oct4 and Sox2, without the need for the oncogenes Klf4 or c-Myc [64]. The two-factor reprogramming efficiency with VPA treatment is comparable to that of three-factor reprogramming without chemical treatment in human fibroblasts [39,64]. Microarray analysis shows that VPA preferentially upregulates ESC-specific genes and downregulates MEF-specific genes in the uninfected MEFs, although VPA treatment alone is not sufficient to generate iPSCs from MEFs [54]. Mechanistically, VPA promotes histone acetylation, and appears to induce in MEFs a global transcriptional program that leans toward an ESC-like state. Besides its enhancing role in reprogramming, VPA has also been suggested to promote complete reprogramming and facilitate expansion of fully reprogrammed iPSCs by inhibiting the growth of nonreprogrammed cells [48,66]. Addition of VPA in four-factor reprogramming generates more homogeneous iPSC colonies that highly resemble ESC colonies [66]. Furthermore, some of these iPSC lines can produce adult mice using tetraploid complementation assays, suggesting that fully pluripotent iPSCs can be generated by combination of VPA with four factors [66]. In addition to reprogramming using the four Yamanaka factors, VPA also promotes reprogramming using cell-permeable recombinant proteins or microRNAs. Zhou et al. showed that VPA is essential to the generation of stable iPSCs from MEFs using recombinant proteins (Oct4-11R, Sox2-11R, Klf4-11R, and c-Myc-11R), a method that circumvents genetic modifications associated with the classic approach, which introduces reprogramming genes through retroviral infections [50]. Three GFP+ colonies per 5 × 104 MEF cells were obtained when they were transduced with four proteins (Oct4-11R, Sox2-11R, Klf4-11R, and c-Myc-11R) and treated with VPA, and one GFP+ colony per 5 × 104 cells when they were transduced with only three proteins (Oct4-11R, Sox2-11R, and Klf4-11R) and treated with VPA. However, no stable GFP+ colonies were obtained from transduction of the three or four reprogramming proteins without VPA for the same period of time [50]. Anokye-Danso et al. showed that combination of VPA treatment and miR302/367 cluster expression can generate iPSCs from MEFs without using any exogenous transcription factors, while miR302/367 alone cannot efficiently reprogram MEFs [52]. Further experiments demonstrated that VPA induced degradation of Hdac2 proteins and low levels of Hdac2 permitted miR302/367 reprogramming. However, using human foreskin and dermal fibroblasts, miR302/367 expression generates iPSCs without VPA, presumably due to low levels of Hdac2 being expressed in human fibroblasts [52]. Sodium butyrate (NaB), a naturally occurring fatty acid commonly used as a nutritional supplement and differentiation agent and an analog of VPA, has also been shown to greatly enhance the efficiency of iPSCs from human adult or fetal fibroblasts [65]. After transient butyrate treatment, the iPSC derivation efficiency is enhanced by 15–51-fold. Butyrate has a stronger effect (100–200-fold) on reprogramming in the absence of either Klf4 or c-Myc transgene. BIX01294 (see Section 9.2.2) can synergize with butyrate to further enhance the butyrate-stimulated reprogramming by three factors in human fibroblasts or mesenchymal stem cells (MSCs). By day 6–12 during reprogramming, butyrate treatment enhances histone H3 acetylation, promoter DNA demethylation, and the expression of endogenous pluripotency-associated genes [65]. Since NaB is an analog of VPA, their reprogramming-enhancing mechanisms might be the same. Histone acetylation and deacetylation regulate chromatin structure and transcriptional activity. The genome-wide acetylation induced by VPA and other HDAC inhibitors could allow fibroblasts to adopt a more open chromatin structure that facilitates the binding of ectopically expressed reprogramming factors or downstream secondary factors [67], and thus promotes reprogramming efficiency. Since NaB and VPA appear to have stronger effects on reprogramming than other HDAC inhibitors, it is possible that these two compounds also act through additional, yet unidentified mechanisms to regulate reprogramming. Histone methylation and demethylation also play important roles in regulating gene expression and reprogramming. BIX01294 (BIX), an inhibitor of the G9a histone methyltransferase [68], improves the reprogramming efficiency in mouse neural stem cells (NSCs) and fibroblasts that were transduced with only two factors (OK) [55,69]. The two-factor (OK) reprogramming efficiency of BIX-treated NSCs is comparable to four-factor (OSKM) reprogramming [55]. Although the overall efficiency is improved, the reprogramming process is not significantly shortened by the presence of BIX. Interestingly, BIX treatment enables reprogramming of NSCs to iPSCs with KSM, without the need for Oct4, albeit at a low frequency [55]. Because G9a promotes histone H3 methylation on Lys 9 and inhibits Oct4 by attracting de novo DNA methylases to the Oct4 promoter [70], by inhibiting G9a, BIX may release repression of endogenous Oct4 and thus reduce the need for exogenous Oct4 during reprogramming of NSCs. It has been shown that Sox2 can maintain ESC pluripotency by regulating the expression of Oct4 [71]. NSCs endogenously express Sox2 and c-Myc, and expression levels of Sox2 and c-Myc are comparable to those in ESCs [72,73]. Generation of iPSCs from NSCs can be achieved by expressing only Oct4 and Klf4 [73]. Therefore, it is not clear whether BIX actually functionally replaced Sox2 and c-Myc in the cases of reprogramming of NSCs. In a further study using OK-infected MEFs, Shi et al. showed that, unlike NSCs, BIX treatment did not effectively enhance generation of iPSCs [69]. However, when combined with a DNMT inhibitor, RG108, and an L-calcium channel agonist, BayK8644, BIX significantly increased reprogramming of MEFs in the absence of Sox2 and c-Myc [69]. The mechanism by which BayK8644 acts remains unclear, however, as BayK8644 alone or in combination with BIX did not alter cell proliferation or Sox2 expression. In a reprogramming study using human primary keratinocytes, the cells were transduced with two-factor combinations (Oct4/Klf4, Oct4/Sox2, and Sox2/Klf4) and treated with CHIR99021 (a glycogen synthase kinase 3 (GSK3) inhibitor) alone or combined with epigenetic modifiers, including inhibitors of DNA methyltransferase (DNMT) (RG108), histone methyltransferase (BIX01294), HDAC (VPA), and lysine-specific demethylase 1 (LSD 1) (Parnate). Using the human pluripotency cell-surface marker Tra-1-81, hESC-like colonies were identified only from cells infected by Oct4 and Klf4 and treated with CHIR99021 and parnate [74]. Parnate, also known as tranylcypromine, is a monoamine oxidase inhibitor. It exhibits a potent inhibitory effect on LSD1 and inhibition of H3K4 demethylation, but it does not affect the acetylation of H3K9/K14. Parnate may facilitate the reprogramming of human keratinocytes by inhibiting H3K4 demethylation. In another study, parnate together with VPA, CHIR99021, and E-616452 (a transforming growth factor β (TGFβ) inhibitor) was sufficient to induce reprogramming in MEFs in combination with a single transcription factor, Oct4, without the need for Sox2, Klf4, or c-Myc [75]. However, how histone demethylase inhibitors enhance reprogramming remains unclear. Chemical inhibitors of histone demethlyases (the iron chelator desferrioxamine or the α-ketoglutarate analog dimethyloxalylglycine) inhibit Sox2/Klf4/Oct4 (SKO) reprogramming in a dose-dependent manner [76]. The combination of the histone demethylases Jhdm1a and 1b can promote reprogramming of MEFs using three-factor SKO, and even more efficiently in the presence of vitamin C [76]. In addition, through its histone demethylase activity, Jhdm1b enables reprogramming with only Oct4 in the presence of vitamin C [76]. In a recent study, a new class of chemical compounds that specifically inhibit histone demethylase LSD1 has been developed [77]. Unlike monoamine oxidase inhibitors, the new LSD1 inhibitors specifically interact with LSD1 and inhibit its activity without forming a covalent bond. These new inhibitors enhance H3K4 methylation and derepress epigenetically suppressed genes in vivo. Strikingly, these compounds inhibited the proliferation of pluripotent cancer cells (including teratocarcinoma, embryonic carcinoma, and seminoma) and ESCs that expressed the stem-cell markers Oct4 and Sox2 while displaying minimum growth-inhibitory effects on nonpluripotent cancer or normal somatic cells [77]. It would be interesting to see whether these new LSD1 inhibitors have a different effect on cellular reprogramming to pluripotency compared with parnate, and such studies might be able to provide further mechanistic understanding of reprogramming. DNA methylation is one of the common epigenetic mechanisms regulating gene silencing. Pluripotent stem cells have a less methylated epigenetic signature than somatic cells. Therefore, inhibition of DNMTs can reactivate the expression of genes that have been repressed by DNA methylation, which may facilitate cellular reprogramming. A prototypical inhibitor of DNMT, 5-Aza-cytidine (5-aza), is a chemical analog of the DNA and RNA nucleoside cytidine. 5-aza needs to be incorporated into DNA to covalently trap DNMTs [78]. 5-aza has a half-life of 17 hours and is considerably cytotoxic [78]. Not only can it enhance reprogramming efficiency, but it also improves reprogramming quality by pushing partially reprogrammed cells forward to a complete reprogrammed state. 5-aza treatment increases the percentage of Oct4-GFP+ cells in both four- and three-factor-infected MEFs in a dose-dependent manner, and increases the number of ESC-like colonies formed during reprogramming [24,54]. Dexamethasone (1 μM), a synthetic glucocorticoid, improves the effect of 5-aza further, although dexamethasone alone has no effect [54]. Furthermore, 5-aza induces a rapid and stable transition to a fully reprogrammed iPSC state when used on partially reprogrammed MEFs [24]. Partially reprogrammed MEFs treated with 5-aza reactivated endogenous Oct4, exhibited demethylation at the promoters of pluripotency genes, and formed teratomas when injected into severe combined immunodeficiency (SCID) mice, just like fully reprogrammed iPSCs [24]. These findings suggest that DNA demethylation is a critical step in direct reprogramming, and that inhibition of DNMT facilitates the transition to pluripotency by lowering this epigenetic barrier [24]. RG108 is a non-nucleoside DNMT inhibitor with a mean half-life of 20 days [78]. It significantly inhibits DNA methylation in cells at 10 μM without detectable toxicity, distinguishing it from nucleoside-based inhibitors like 5-aza. Furthermore, RG108 can inhibit free DNMTs; the inhibition of DNMT activity is achieved by blocking the enzyme active site [78]. RG108 enhances the reprogramming efficiency of MEFs transduced with Oct4 and Klf4 in the presence of a histone methyltransferase inhibitor, BIX01294 [69]. It also increases the reprogramming efficiency of human fibroblasts transduced with all four Yamanaka factors (OSKM) by twofold [65]. Although RG108 is a more potent and noncovalent DNMT inhibitor than 5-aza, it appears to have a lesser effect on reprogramming. This could be due to the differences in experimental specifications in different studies. The mechanism by which RG108 enhances reprogramming has not been further elucidated, though it is reasonable to assume that RG108 and 5-aza both promote reprogramming by modulating DNA methylation. More recently, Lee et al. reported that a novel indoleacrylic acid analog, named RSC133, promotes iPSC generation from both mouse and human fibroblasts [79]. Continuous treatment with RSC133 throughout reprogramming increased the reprogramming efficiency by up to fourfold. Under hypoxic conditions (5% O2), RSC133 synergistically increased the reprogramming efficiency to five- to sixfold compared to untreated controls [79]. On treatment with RSC133, OSKM-transduced human fibroblasts displayed a rapid activation of pluripotency genes and highly proliferative hESC-like colonies in 10 days, suggesting that RSC133 significantly enhanced both the efficiency and kinetics of the reprogramming process [79]. The enhanced reprogramming efficiency was most likely due to RSC133 promotion of cell proliferation, ablation of pro-senescence phenotypes, inhibition of DNMT1 activity, and subsequent reduction of HDAC activity [79]. 5-aza, RG108, and RSC133 are structurally very different DNMT inhibitors, and the ways in which they modulate DNMT activities are also different. It is not clear whether these differences have any effects in their promotion of reprogramming.
Chemicals Facilitating Reprogramming
9.1 Introduction
Chemical
Category
compounds
Working conditions and effects
HDAC inhibitor
VPA, TSA, SAHA
Enhance iPSC formation from four-factor-infected MEFs [54]
VPA
NaB
G9a HMTase inhibitor
BIX01294
LSD1 inhibitor
Parnate
DNMT inhibitor
5-aza
RG108
RSC133
Enhances reprogramming efficiency and kinetics of mouse and human fibroblasts transduced with OSKM [79]
TGFβ inhibitor
E616451
Replaces Sox2 to induce iPSCs from OKM-transduced MEFs only in the presence of VPA [81]
E616452 (RepSox)
Functionally substitutes transgenic Sox2 and alleviates the need for c-Myc for reprogramming in MEFs [81, 82]
SB431542
A-83-1
Wnt signaling
Wnt3a
Wnt3a-conditioned medium significantly promotes reprogramming of MEFs transduced with OSK, but not OSKM [94]
GSK3 inhibitor
CHIR99021
Kenpaullone
Induces iPSC generation from MEFs transduced with OSM [98]
Rock inhibitor
Y27632
Widely used in hiPSC generation, improving cell survival and colony formation
Thiazovivin
Promotes reprogramming when combined with SB431542 and PD0325901 [86]
Aurora kinase inhibitor
BIM0086660
Enhances reprogramming of MEFs transduced with OSKM [102]
Src kinase inhibitor
EI275
Enables reprogramming of MEFs transduced with OKM when combined with VPA [81]
Dasatinib, PP1, and iPYrazine
Sufficient to support reprogramming of MEFs in the absence of exogenous Sox2 [104]
MEK inhibitor
PD0325901
L-calcium-channel agonist
BayK8644
Improves reprogramming of MEFs transduced with OK when combined with BIX01294 and RG108 [69]
PKA activator
8-Br-cAMP
Improves reprogramming efficiency of human neonatal foreskin fibroblasts [105]
PDK1 activator
PS48
Promotes iPSC generation from human somatic cells transduced with only Oct4 when combined with NaB and A-83-01 [106]
E-cadherin inducer
Apigenin and Luteolin
Enhance mouse iPSC generation [100]
Senescence alleviator
Resveratrol
Improves reprogramming efficiency [142]
Vitamin C
9.2 Chemicals Modulating Epigenetic Barriers
9.2.1 Histone Deacetylase Inhibitors
9.2.2 Histone Methyltransferase Inhibitor and Demethylase Inhibitor
9.2.3 DNA Methyltransferase Inhibitors
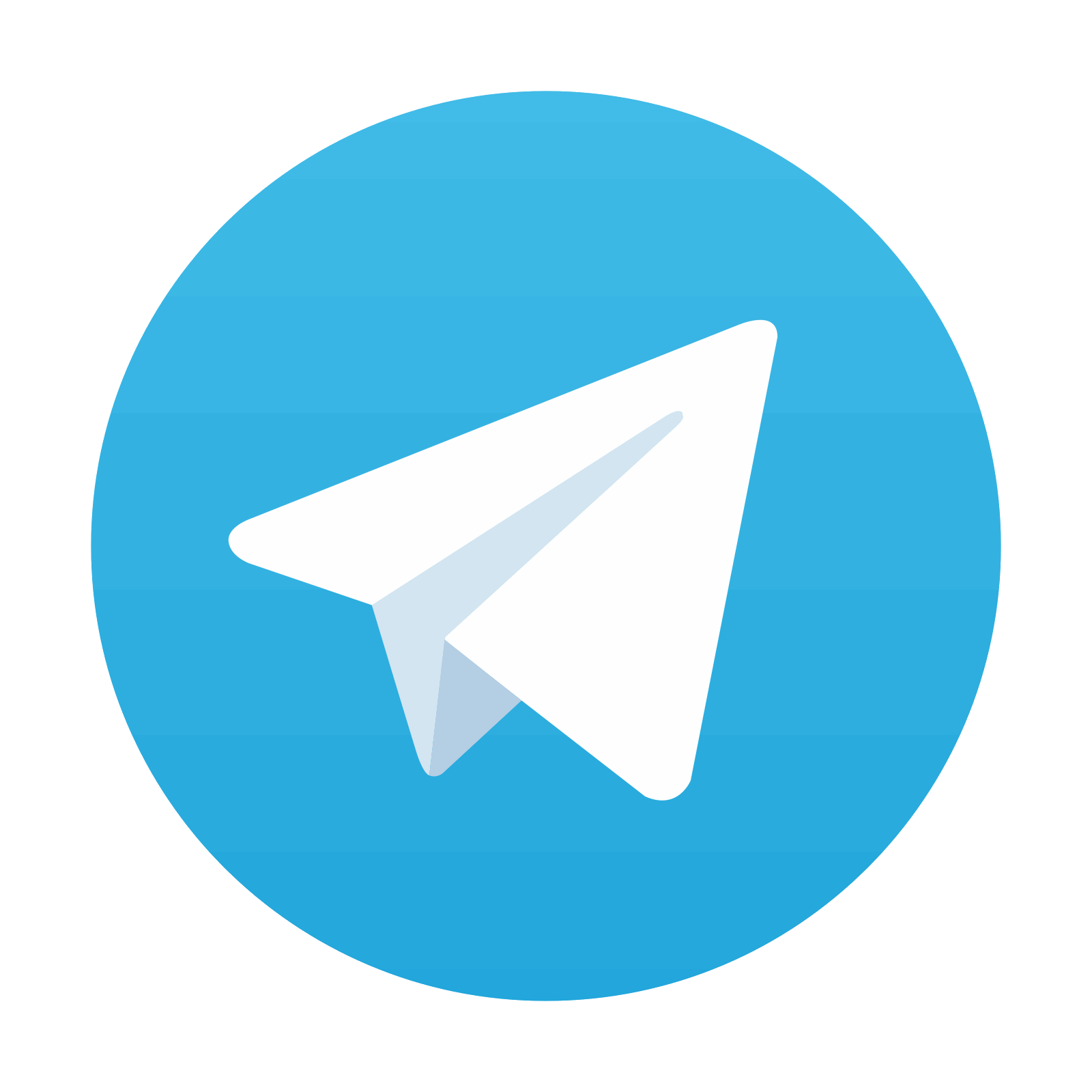
Stay updated, free articles. Join our Telegram channel

Full access? Get Clinical Tree
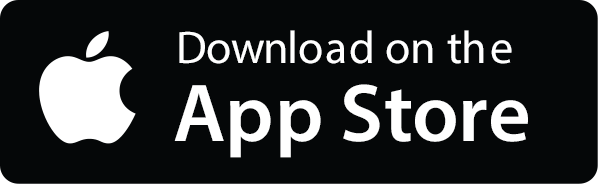
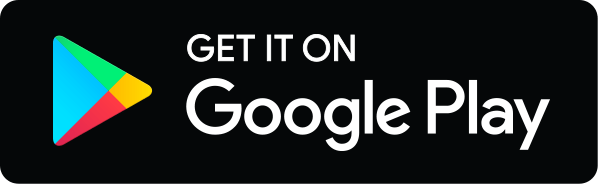