6 Dikshya Bastakoty1, Sarika Saraswati2, and Pampee P. Young3 1Department of Pathology, Vanderbilt University, USA 2Department of Pathology, Microbiology and Immunology, Vanderbilt University School of Medicine, USA 3Department of Pathology, Microbiology and Immunology, Vanderbilt University, USA Living beings possess an inherent ability to repair normal wear-and-tear of tissue caused by daily activities; this attribute is known as physiological regeneration [1]. Examples of physiological regeneration include replacement of blood cells, turnover of epithelial cells of the gut and skin, and renewal of endometrium after menstrual cycle. On the other hand, reparative regeneration refers to the replenishment of cells or tissues after post-traumatic injury. Large cellular or tissue loss due to trauma leads to the activation of specific signaling pathways aimed at repairing injury, which in some cases completely heals the injury and preserves the functionality of the lost tissue and in others leaves a healed but nonfunctional (fibrotic) scar tissue. The former type of healing occurs in many vertebrates, such as zebrafish, while the latter is more common in mammals. Through a better understanding of the process of regeneration in these diverse species, the field of regenerative medicine works toward developing ways of maximizing repair and minimizing fibrosis or scarring in humans. As the world’s aging population grows, there is an increasing demand on medicine to slow down or reverse the degeneration of organs and tissues. The US National Institutes of Health (NIH) reported in 2010 that the world market for replacement organ therapies is $350 billion, and that for regenerative medicine it is $500 billion [2]. As a result of this increasing demand, the field of regenerative medicine, which started with surgical implants and transplants in the 1950s, has grown to encompass cell therapy, novel chemicals and biologics, and increasingly sophisticated biomaterials for scaffold or delayed delivery of chemicals. With the discovery of stem cells and their potential to replace various cells in the adult organisms, stem-cell therapy has become the mainstay of modern regenerative medicine. The potential of stem cells to regenerate lost tissue depends on several parameters, including their delivery into the injured tissue, their differentiation into the desired cell type, their cooperative integration in the injury site to form functional tissue and – most important of all – their survival in the injured microenvironment. Hence the intrinsic healing potential of stem cells is better harnessed with the use of growth factors, biologics, small molecules, and biomaterials that aid their delivery, survival, and integration into the wound. In this review we will outline the use of these approaches to promote regeneration both in conjunction with stem cells and independent of cell therapy. We will particularly focus on the use of small molecules in stem-cell therapy in modern regenerative medicine. Biologics, in the widest sense, are agents derived from proteins produced by organisms. Growth factors, antibodies, and vaccines are some of the most common biologics that have been used to treat or prevent illnesses of various forms. In regenerative medicine, growth factors are used extensively and successfully for a number of indications [3,4]. With increased understanding of the signaling pathways implicated in tissue regeneration, antibodies or proteins that antagonize or activate certain pathways have emerged as therapeutic candidates. Some common biologics-based approaches in regenerative medicine are discussed in this section. Ever since their Nobel Prize-winning discovery by Rita Levi-Montalcini and Stanley Cohen in the 1950s [5,6], growth factors have been reported to play increasingly diverse and important roles in cell growth and differentiation. These roles lend them importance not only in development but also in regeneration after injury [3] (clinicaltrials.org: NCT00000842). Epidermal growth factor (EGF) is found to enhance healing of wounds in the skin and cornea [7]. Nerve growth factor (NGF) and brain-derived or glial cell-derived neurotropic factors (BDNF and GDNF, respectively) have been shown in rat models of nerve damage to enhance neuronal regeneration [8,9]. Similarly, platelet-rich plasma (PRP) has been shown in numerous animal studies to enhance tissue regeneration after injuries [10]. The plasma extracted from blood is enriched in the laboratory for platelets, which contain presynthesized growth factors packaged in α-granules. Upon activation of clotting (after infusion into the injured tissue) these growth factors are secreted into the tissue microenvironment. Autologous transplant of PRP for the treatment of various degenerative diseases of tissue or bone has reached clinical trials (clinicaltrials.gov: NCT00761423, NCT01355549). Although the results from many of the trials and existing clinical reports have been promising (but inconsistent or ambiguous at best [11,12]), there still seems to be anticipation that with proper standardization of the techniques for plasma isolation, enrichment, storage, and delivery, better and more consistent outcomes can be expected. Pro-angiogenic factors such as vascular–endothelial growth factor (VEGF), fibroblast growth factor (FGF), and platelet-derived growth factor (PDGF) are another subclass of growth factors that have been utilized to improve regeneration by inducing neovascularization at the site of injury [13,14]. The immune system plays an important role in the resolution of injury. Early after injury, it clears the site of injury of debris and infectious agents, and allows repair to begin. However, persistent and uncontrolled inflammation can be detrimental to wound repair [15], so drugs that modulate the inflammatory response have long been utilized to enhance it. Thrombin peptide (THP508) was reported to enhance fracture healing through activation of tumor necrosis factor-α (TNFα) and other pro-inflammatory factors [16]. On the other hand, attenuation of inflammatory response has also been shown to enhance regenerative repair. Several reports have shown that administration of high doses of nonsteroidal anti-inflammatory drugs improve regeneration of brain tissue after the ischemic injury induced by a stroke in rodents [17,18]. Even the ability of stem-cell therapy using mesenchymal stromal or stem cells (MSCs) to enhance tissue regeneration is attributed in part to their immune-modulatory properties [19]. The extracellular matrix (ECM) plays a key role in each step of injury repair. Within days after injury, a fibrous matrix is synthesized to replace lost or damaged tissue. The matrix is remodeled over time as the repair progresses. All the steps, from inflammation to reepithelialization to contraction, involve very close interactions with matricellular proteins, which sequester growth factors, regulate collagen turnover, and provide scaffold and support to allow cells to grow during tissue repair [20]. ECM components, such as heparin, bind many signaling molecules and growth factors and enhance their signaling by sequestering them to the microenvironment or by stabilizing them. They have been used to enhance signaling by proteins of interest [21]. Moreover, a polymer mimetic of heparan sulfate proteoglycan itself has demonstrated remarkable healing properties in both topical wounds and internal injuries [22,23]. A clinically available formulation is already in use in Europe and the Middle East to treat corneal ulcers and diabetic wounds [24]. Since heparan sulfates are important for the signaling of growth factors and cytokines, their degradation and cleavage in a wound microenvironment impairs healing. The heparan sulfate mimetics are uncleavable by heparanases and glycanases and hence aid repair of the wounded tissue [25]. Biologics such as proteins and growth factors have the potential to significantly improve regeneration. However, in order for the healing agent to be effective it is important to ensure that it is delivered to the tissue of interest at a substantial concentration and that it is bioavailable at the site of injury. Hence, modern regenerative medicine is integrating diverse disciplines such as chemistry, material physics, and bioengineering in order to devise strategies for optimizing regeneration. Small molecules, bioactive scaffolds, and drug-delivery tools are the major new outcomes of these multidisciplinary investigations. A small molecule is a low-molecular-weight (smaller than 500 Daltons) organic compound. Small molecules that are investigated for use as therapeutics typically bind to a protein, nucleic acid, or polysaccharide and alter its enzymatic activity or biological function [26]. Small molecules are identified by cell phenotypic, reporter-based, or organism-based screening of chemical libraries [27]. Phenotypic screening is based on the cellular phenotype or cell-surface markers in response to the chemical library. In order to identify the modulators of specific pathways, promoter-driven reporters are used as readouts for the pathways of interest. Zebrafish and xenopus are routinely used for organism-based screens [28]. There is increasing interest in finding small molecules that can modulate signaling pathways as they have a number of advantages over biologics such as proteins and antibodies. For one thing, they are cheaper to synthesize. Due to their small size, they are often orally bioavailable, and hence delivery may not require injection. Additionally, they are more likely to be cell-permeable and thus to allow modification of the pathway at multiple steps; an antibody would rely solely on the sequesterization of a ligand or the blocking of the receptor. Hence, small molecules are very useful in blocking intracellular protein–protein interactions, which has to date been the sole domain of antisense oligos. Small molecules thus offer a safer, more effective, and easier alternative to traditional signaling pathway-modulation approaches [26]. Given their superiority in modifying molecular signals, many types of small molecule have been investigated for potential roles in regeneration. Small-molecule antioxidants such as quercetin, allopurinol retinoids, and uric acid are reported to enhance wound healing [29]. Ascorbic acid, another small molecule, commonly used for stem-cell differentiation, is also known to regulate ECM by increasing collagen synthesis [30]. In a model of combined injury (fracture/irradiation) in mice, Greenberger and colleagues showed that the small molecule GS-Nitroxide attenuates ionizing radiation-driven delay in fracture healing [31]. Another small molecule, Pirfenidone, has been evaluated in clinical trials as an antifibrotic agent for idiopathic pulmonary fibrosis. Already reported to reduce fibrosis in lung, liver, heart, and kidney through the modulation of the transforming growth factor beta (TGFβ) pathway, the molecule has potential for therapeutic application in tissue injury, through promoting regeneration by minimizing scarring [32]. Work by our group has also shown that in a mouse myocardial infarct model, treatment with the small-molecule Wnt inhibitor Pyrvinium alleviates adverse cardiac remodeling post-infarct [33]. The same molecule, upon topical treatment on mouse-ear punch injury, caused dramatic closure of the holes with remarkable restoration of tissue structure, including hair-follicle regeneration [34]. However, careful and thorough screenings of these molecules need to be performed before they can be proposed for therapeutic purposes, since some may have toxicity and off-target effects. Nonetheless, with careful considerations of their shortcomings, the exciting prospects for small molecules in advancing regenerative medicine cannot be overlooked. As the field of regenerative medicine has become more multidisciplinary, innovative approaches to drug delivery, in vitro organ regeneration, and cell therapy have been introduced. These new technologies have revolutionized the field of regenerative medicine by increasing the efficacy and versatility of the traditional methods of enhancing regeneration through cell- or drug-based approaches. Tissue engineering has been an important part of the field of regenerative medicine for many decades now. With the design and application of sophisticated biomaterials, tissue engineering is moving even further toward improving regenerative therapy [35]. Degradable biomaterials composed of collagen, fibronectin, chitosan, and so on have been used for the delivery of cells and to provide ECM components for the survival or directed differentiation of injected cells. Alginate, an algae-derived polysaccharide, has been used in the form of sponge or gel to enhance the regeneration of peripheral nerve [36], spinal cord [37], or excised axons [38] in rodent or feline models when used alone or in combination with neural progenitor (neurosphere) cells [39]. In a remarkable study, Atala and colleagues used a cell-polymer matrix composed of a Poly (Lactide-co-Glycolide)-poly(D,L-lactide-co-glycolide) (PGA-PLGA) scaffold lined with urothelial cells on one side and muscle cells on the other to show for the first time the reconstitution of an autonomous and functional hollow organ using a tissue-engineering approach [40]. Another avenue in which bionics and biomaterial science are contributing to better tissue regeneration is in the sustained delivery of stabilized growth factors or drugs. In 2003, Hyongbum Kim et al. reported successfully using PLGA scaffolds for the slow release over a month of ascorbate-2-phosphate (AsAP) and dexamethasone to induce osteogenesis from MSCs [41]. Another recent study showed that an injectable polyvalent coacervate of a polycation and heparin used as a matrix for the sustained delivery and stabilization of FGF2 increased FGF2-mediated angiogenesis in a rodent model [21]. Stem cells were discovered by Becker et al. when they noted that bone-marrow cells injected into irradiated mice developed clonal nodules [42]. Subsequent studies by multiple groups have shown that stem cells are, by definition, self-renewing, multipotent cells. They exist in the embryo and in specific microenvironments called stem cell niches of tissues of the adult organism [43]. Although stem cells from different sources may vary in their degree of multipotency, virtually all stem cells (or progenitor cells, which are stem cells partially committed to a specific lineage) possess some degree of multipotency and capacity for self-renewal. These fundamental properties have placed stem cells on the forefront of regenerative biology. Also, there is increasing evidence to support the hypothesis that in almost all of the regenerative approaches mentioned in the preceding sections, true regeneration is driven by stem or progenitor cells in the wound milieu [44]. Although this statement may be partly contested, stem cells have for the past 2 decades been the buzzword in the context of regenerative therapies. By the year 2000, multiple studies had shown that stem cells from various tissues could give rise to unrelated cell types under special circumstances [45]. For example, Clarke et al. observed that neural stem cells (NSCs) transferred to a blastocyst could contribute to tissues belonging to all three germ layers [46]. These observations pointed toward their value in the regeneration of tissues that normally do not regenerate [45]. Ethical controversies surrounding embryonic stem cells (ESCs) stalled progress in stem-cell research, but the identification of stem cells in tissues of adult organisms opened up new avenues for research. Stem cells from various organs have now been used for many diseases and multiple types of injury, from bone injury, to degenerative diseases such as Alzheimer’s, Parkinson’s, and Huntington’s, to cardiac, renal, and lung injuries [47]. In 2003, Lin et al. reported that hematopoietic stem cells (HSCs) transplanted into kidney after ischemia/reperfusion injury contributed to the regeneration of renal tubules [48]. MSCs from the bone marrow have perhaps been used most extensively in regenerative medicine for their ease of isolation and characterization, their multipotency, and most importantly their immunosuppressive properties. The latter have made allogeneic transplantation possible, increasing the feasibility of MSC transplant therapy [49]. Multiple studies using diverse injury models have reported improved regeneration by stem-cell transplantation [50,51]. This has led to several clinical trials aimed at improving regeneration using stem-cell therapy (clinicaltrials.gov: NCT01763099, NCT00877903). Not all of these studies have unambiguously shown remarkable success. However, with improved mechanistic insight and technical standardization, there is potential for improvement. Moreover, progress in tissue engineering and chemical biology is now providing excellent tools by which we can better understand stem cell biology and enhance the efficacy of stem-cell therapy. In order to be useful for clinical purposes, stem cells should maintain their self-renewal ability and be able to differentiate into specific lineages. Several biologics and genetic manipulations have been used to control the fate of stem cells, driving their self-renewal, differentiation, survival, or reprogramming. However, modulation of these complex biological events with small molecules seems to be safer and more effective in regulating stem-cell fate. Using high-throughput screening of chemical libraries, many molecules have been identified that target specific cellular pathways to maintain stem-cell self-renewal, direct stem-cell differentiation, and enable or enhance stem-cell reprogramming [27, 52]. Here we will focus on recent interventions introduced by small molecules in affecting and/or improving the behavior of different types of stem cells commonly used in regenerative biology. ESCs are stem cells derived from the blastocyst stage of the embryo [53,54]. These cells are pluripotent, clonal, and self-renewing, and can differentiate into the cells of three germ layers. They are therefore a strong candidate for regenerative therapies [55]. However, the use of ESCs is limited due to ethical considerations, potential rejection problems following their allogeneic transplantation, and the formation of teratomas after in vivo injection. Traditionally, ESCs require feeder cells (growth-inactivated fibroblasts), fetal bovine serum, growth factors, and knockout serum replacement in order to grow in culture. However, there is a lack of fully defined and reproducible culture conditions that would allow them to grow in vitro. Additionally, the need for interventions such as feeder cells and other animal serum products imposes risk of contamination. To overcome this issue, Chen et al. performed a high-throughput cell-based screening on a mouse ESC line. This led to identification of a small molecule (Pluripotin) that could maintain the self-renewal status of ESCs under serum-free, feeder cell-free, and growth factor (leukemia inhibitory factor, LIF)-free conditions [52]. Pluripotin exerts its effect by inhibiting Ras GTPase-activating protein (RasGAP) and extracellular signal-regulated kinase-1 (ERK1). This was a breakthrough in the area of ESC research, since it was thought to be impossible to culture ESCs without feeder cells or any extrinsic growth factors. Following this study, another group used a combinatorial approach in which a mitogen-activated protein kinase kinase (MEK) inhibitor, PD0325901, and a glycogen synthase kinase 3 (GSK3) inhibitor, CHIR99021, were used to maintain mESC culture under cytokine-free and feeder-free conditions [56]. One persistent hurdle in the expansion of ESCs was the induction of cell death upon single-cell dissociation; this has been overcome by using the Rho-associated coiled coil-containing protein kinase (ROCK) inhibitor Y-27632 [57].
Chemicals and Stem Cells in the Promotion of Regeneration
6.1 Introduction
6.2 Biologics in Regenerative Medicine
6.2.1 Growth Factors and Pro-Angiogenic Agents
6.2.2 Immune-Modulatory Therapies
6.2.3 Extracellular Matrix-Based Approaches
6.3 Chemicals and Biomaterials for Healing
6.3.1 Small Molecules
6.3.2 Biomaterial Scaffold and Sustained Delivery
6.4 Stem-Cell Therapy
6.4.1 Chemical Manipulation of Stem Cells in Regeneration
6.4.2 Embryonic Stem Cells (ESCs)
6.4.2.1 Small Molecules for the Culture and Maintenance of ESCs
6.4.2.2 Small Molecules for ESC Differentiation
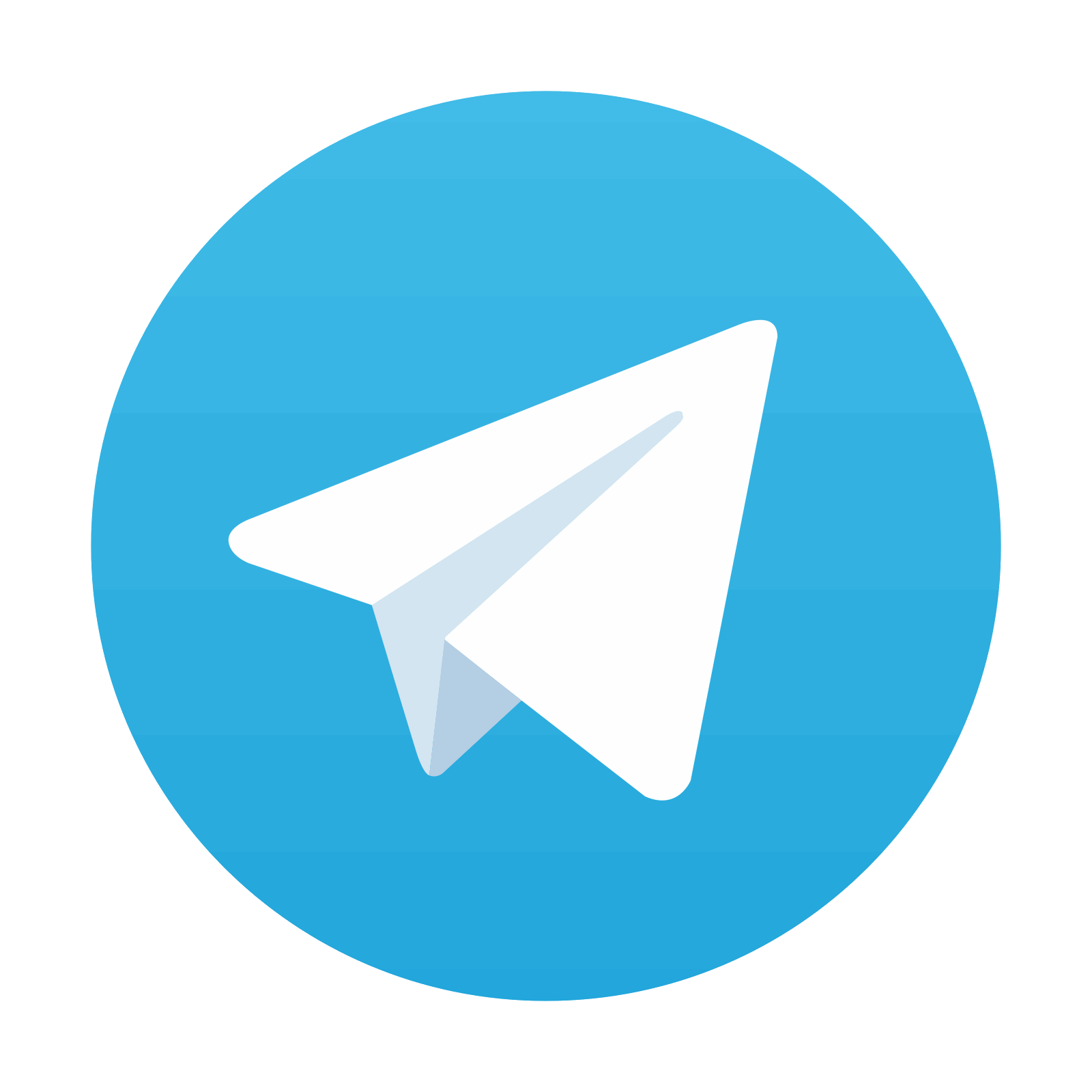
Stay updated, free articles. Join our Telegram channel

Full access? Get Clinical Tree
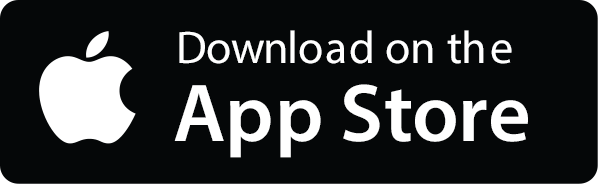
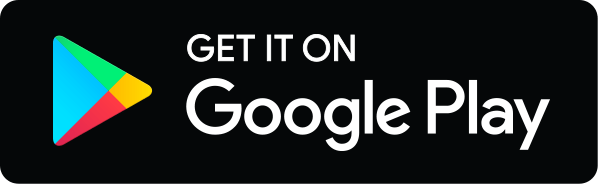