3 Daqing Jin1, Qiao Li1, and Tao P. Zhong2 1Department of Genetics, Fudan University School of Life Sciences, China 2State Key Laboratory of Genetic Engineering, Fudan University School of Life Sciences, China; Department of Medicine, Vanderbilt University, USA Myocardial infarction and ischemic heart disease are caused by an acute reduction of blood to the myocardium, which has the direct effect of diminishing oxygen supply. This results in a loss of large numbers of cardiomyocytes, leading to hypertrophy and heart failure. Care of such patients places enormous burdens on the health care system, requiring 1 million hospitalizations every year at an annual cost of $35 billion in the USA [1]. Development of therapeutics that can facilitate new cardiomyocyte generation would be of enormous medical and economic impact. One option for replenishing a depleted cardiomyocyte population is transplantation of exogenous cells. Over the past decade, many cell types have been evaluated in order to find the best source for cardiac cell therapy. A wide variety of stem cells, including bone marrow mononuclear cells (BMCs), mesenchymal stem cells (MSCs), cardiac stem cells (CSCs), and embryonic stem cells (ESCs), have been examined regarding their capacity to repair the infarcted myocardium and improve functionally the diseased heart [1,2]. These stem cells have been transplanted in animal models of myocardial infarction and have been demonstrated to reduce infarct size, attenuate left-ventricle remodeling, increase vascular density, and improve myocardial function [1,2]. However, a recent clinical trial to test BMC therapy in patients with ischemic heart failure revealed no beneficial effects [3], raising considerable concerns that BMCs and other exogenous stem cells may have low capacities to integrate and differentiate into cardiomyocytes in the patient heart. Other trials are underway to examine the potential clinical effects of c-Kit-positive and cardiosphere-derived stem cells for the treatment of acute myocardial infarction. These findings highlight the importance and urgency of developing alternative therapeutic approaches. The advent of induced pluripotent stem cells (iPSCs) has offered a possible solution in terms of generating functional cardiomyocytes. Somatic cells can be reprogrammed into an embryonic-like state by transferring nuclear contents into oocytes [4] or by fusion with embryonic stem cells (ESCs) [5,6]. In 2006, Yamanaka et al. hypothesized that factors that play essential roles in the maintenance of ESC identity may also play pivotal roles in the induction of pluripotency in differentiated cells [7]. They developed a clever screen in which induction of the pluripotent cell state could be selected by the expression of a neomycin/lacZ fusion gene (βgeo). The βgeo cassette was inserted into the Fbx15 locus in the genome of mouse embryonic fibroblasts. Fbx15 can be specifically expressed in mouse ESCs and is indispensable for the maintenance of ESC pluripotency and mouse development. In the pluripotent stem cells, the Fbx15 promoter activates βgeo expression, resulting in G418 resistance. The initially selected candidate genes were pooled together and delivered to fibroblasts by retroviral transfection to test for reprogramming. Successful combinations of factors capable of G18 resistance were selected. This pool was then narrowed down by reducing factors to Oct4, Klf4, Sox2, and c-Myc (OKSM). iPSCs generate various cell types in teratoma assays and can contribute to tissues in chimeric mice after transplantation into mouse blastocysts. Human pluripotent stem cells (hPSCs) can also be generated from fibroblasts expressing OKSM or slightly different combinations, including Nanog, Lin28, Sox2, and Oct4 [8,9]. Recently, it was demonstrated that OKSM factors initially interact with the genome during cell reprogramming in the first 48 hours [10]. These factors extensively access distal regulatory elements and activate transcription of many genes required for reprogramming. In both undifferentiated stem cells and fully differentiated cells, genes can exist in stable states in which they are transcriptionally silent. During initial reprogramming, a subset of transcription factors termed “pioneer factors” initially access closed chromatin and directly make it competent to be bound by other factors [10]. Oct4, Sox2, and Klf4 together bind as pioneer factors to closed chromatin sites, while c-Myc facilitates the binding of OSK to chromatin. Many of the genes that promote cell reprogramming reside in the closed chromatin regions of the genome. Megabase-scale chromatin domains are spanned by H3K9me3. Knockdown of relevant histone methyltransferases allows OSKM binding and enhances cell reprogramming. H3K9me3-containing regions of the genome are refractory to initial OSKM binding and thereby impede the efficiency of reprogramming [10]. Investigating the initial binding of OSKM to the fibroblast genome helps with understanding the mechanisms of reprogramming, leading to potential therapeutic applications. The iPSC technique represents a breakthrough in the stem cell field and offers new perspectives on generating functional cardiomyocytes, but it comes with safety issues. For example, use of iPSCs is hindered by the potential risks of genetic mutations caused by the integration of exogenous genetic material into chromosomes. Although several nonintegrative methods (e.g. episomal plasmid, protein, and mRNA transfection) have been developed to generate iPSCs, induction efficiency is still quite low [11]. In contrast, small molecules provide several distinct advantages in controlling protein functions (e.g. temporal control, reversibility, tunability, modularity, and tractability). Hence they have attracted much interest in relation to steering reprogramming toward a more efficient and safe process [11]. To date, various strategies have been developed to generate iPSCs with fewer or no exogenous genetic manipulations. Different combinations of chemical compounds have been identified to replace some exogenous transcription factors and enhance the efficiency of reprogramming. Recent reports indicate that reprogramming efficiency can be enhanced by the presence of small molecules, such as valproic acid (VPA, a histone deacetylase inhibitor), AZA (a DNA methyltransferase inhibitor), butyrate (a histone deacetylase (HDAC) inhibitor), and vitamin C (Figure 3.1) [12–15]. Figure 3.1 During iPSC reprogramming, a variety of small-molecule compounds have been identified as enhancing pluripotent reprogramming efficiency in the presence of four transcriptional factors (OSKM) or Oct4 through cell senescence, epigenetic remodeling, metabolism shift, and mesenchymal–epithelial transition (MET) Vitamin C improves iPSC generation mainly by reducing p53 levels and alleviating cell senescence while still maintaining an intact DNA repair machinery (basal levels of p53) [14]. It is possible that it accelerates transcriptome changes during reprogramming in other ways as well. Vitamin C is a cofactor in reactions driven by dioxygenases, including collagen prolyl hydroxylases, hypoxia-inducible factor, and histone demethylases. During reprogramming, vitamin C may increase the activity of these enzymes, promote epigenetic modifications, and enhance iPSC generation. For example, vitamin C may allow the reprogramming to run smoothly by activating histone demethylases, which are important for development and modulate the expression of the ESC master transcription factor Nanog [16]. It is tempting to speculate that vitamin C, as a compelling antioxidant, might improve the efficiency of reprogramming by suppressing reactive oxygen species (ROS) produced by cell metabolism [14]. VPA, an HDAC inhibitor, can increase reprogramming efficiency and enable efficient induction of iPSCs without introducing the oncogene c-Myc, suggesting that chromatin modification is a key step in reprogramming fibroblasts to pluripotent cells [12]. Likewise, butyrate, a small-chain fatty acid, acts as HDAC inhibitor and promotes protein acetylation at targets such as H3K9 [15]. Simultaneously, it also accelerates promoter DNA demethylation and expression of endogenous pluripotency-associated genes such as POU5F1/OCT4 and DPPA2. Furthermore, butyrate may possibly stimulate reprogramming by modulating the activities of nonhistone key regulators, as it exhibits diverse cellular effects in culture, including cell-cycle arrest and induction of protein synthesis [17]. AZA (5-aza-cytidine), acting as an inhibitor of DNA methyltransferase (DNMT), improves the overall reprogramming efficiency, indicating that epigenetic remodeling is a key step in the reprogramming process [13]. Furthermore, the small molecules SB431542 and PD0325901, acting as inhibitors of transforming growth factor beta (TGFβ) and MAPK/ERK pathways, respectively, significantly enhance the reprogramming efficiency of human fibroblasts through the expression of the four transcriptional factors (Figure 3.1) [18]. TGFβ is a prototypical cytokine for the induction of epithelial–mesenchymal transition (EMT) and the maintenance of the mesenchymal state [19]. MAPK/ERK signaling facilitates EMT [20] and occurs downstream of TGFβ [21]. The positive effects of SB431542 and PD0325901 on reprogramming indicate that TGFβ and MAPK/ERK pathway antagonists have a direct impact on the reprogramming process, mainly by promoting the mesenchymal–epithelial transition (MET). Ding’s group was the first to report that a small-molecule combination – the G9a histone methyltransferase inhibitor BIX-01294 (BIX) and the L-type calcium channel agonist Bayk8644 (Bayk) – can enable mouse embryonic fibroblasts (MEFs) to reprogram into iPSCs through the transduction of two factors (Oct4 and Klf4) [22]. BIX treatment causes histone modification at the epigenetic level and thus enhances reprogramming activity. Bayk works synergistically with BIX to further increase reprogramming efficiency and has no impact on reprogramming in the absence of BIX. Bayk might influence the reprogramming process in specific manners rather than at general epigenetic levels [22]. Both mouse and human iPSCs can be generated by ectopic expression of Oct4 plus different combinations of small molecules. A defined small-molecule cocktail, including the histone deacetylase inhibitor NaB, the TGF-G receptor inhibitor A-83-01, the MAPK/ERK inhibitor PD0325901, and PS48 (an activator of 3-phosphoinositide-dependent kinase-1), is sufficient to reprogram human primary somatic cells to iPSCs through the expression of a single transcription factor, Oct4 (Figure 3.1) [23]. It is known that many types of stem cell, including pluripotent stem cells, mainly rely on glycolysis followed by lactic acid fermentation in the cytosol to produce energy [23]. This is in contrast to most differentiated cells, in which oxidation of pyruvate in mitochondria is used to produce energy. This might be advantageous for stem cells, as glycolytic metabolism effectively produces various macromolecular precursors in order to meet metabolic and energy demands, while generating fewer ROS (which induce oxidative damage). Consistent with the hypothesis that PS48 facilitates a metabolic conversion from mitochondrial oxidation to glycolysis during the reprogramming process, treatment with PS48 activates downstream AKT/PKB, upregulates expression of several key glycolytic genes, and consequently enhances glycolysis, as measured by increased lactate production. Notably, several known small molecules that have been widely used to modulate mitochondrial oxidation, glycolytic metabolism, or hypoxia-inducible factor (HIF) pathway activation also show corresponding effects on reprogramming. For example, compounds that promote glycolytic metabolism (such as 2,4-dinitrophenol and N-oxaloylglycine) enhance reprogramming, whereas compounds that block glycolytic metabolism (such as oxalate) inhibit reprogramming (Figure 3.1) [23–25]. A study from Li et al. showed that the combination of four small molecules, VPA (an HDAC inhibitor), CHIR99021 (a glycogen synthase kinase 3β (GSK3β) inhibitor), 616452 (a TGFβ signaling inhibitor), and tranylcypromine (TAYPM, an H3K4 demethylation inhibitor) (referred to collectively as VC6T), was sufficient to generate iPSCs from mouse fibroblasts with a single transcription factor, Oct4, thus replacing Sox2, Klf4, and c-Myc (Figure 3.1) [26]. Based on previous studies, Li et al. concluded that VC6T may facilitate the reprogramming process by lowering four major barriers (two epigenetic barriers and two signaling barriers). The effects of VPA and tranylcypromine suggest that H3K4 demethylation and histone deacetylation are two critical epigenetic barriers to reprogramming that may repress the establishment of a pluripotency transcriptional network. In embryonic stem cells, it has been revealed that Tcf3, one of the key transcriptional regulators downstream of the Wnt pathway, occupies and regulates the promoters of Oct4, Sox2, and Nanog [27,28]. In MEFs, these endogenous pluripotency transcription factors are silenced. During reprogramming, Wnt signaling can directly potentiate the effect of exogenous Oct4, Sox2, and Klf4, as it does in ESCs [27], thereby directly promoting the induction of pluripotency in the absence of c-Myc transduction. Inhibition of GSK3β to activate Wnt signaling could enhance mESC self-renewal and cell reprogramming, possibly by regulating the stability of the c-Myc protein [29,30]. As is known, the MET is a crucial initiating event in the derivation of iPSCs from fibroblasts [31,32]. TGFβ induces EMT by both Smad-dependent and Smad-independent signaling events. Recent studies demonstrate that full reversal of EMT morphology and patterns of gene expression can be accomplished by concurrently inhibiting TβRI (TGFβ type I receptor) kinase. Besides promoting MET [32–34], TGFβ inhibition has also been reported to facilitate Nanog gene expression [35]. Together, these findings indicate that GSK3β and TGFβ signaling may be two major signaling barriers that normally repress the reprogramming process.
Chemical Genetics in Cardiomyocyte Generation
3.1 Introduction
3.2 iPSC Generation
3.3 The Chemical Genetics Approach in iPSC Generation
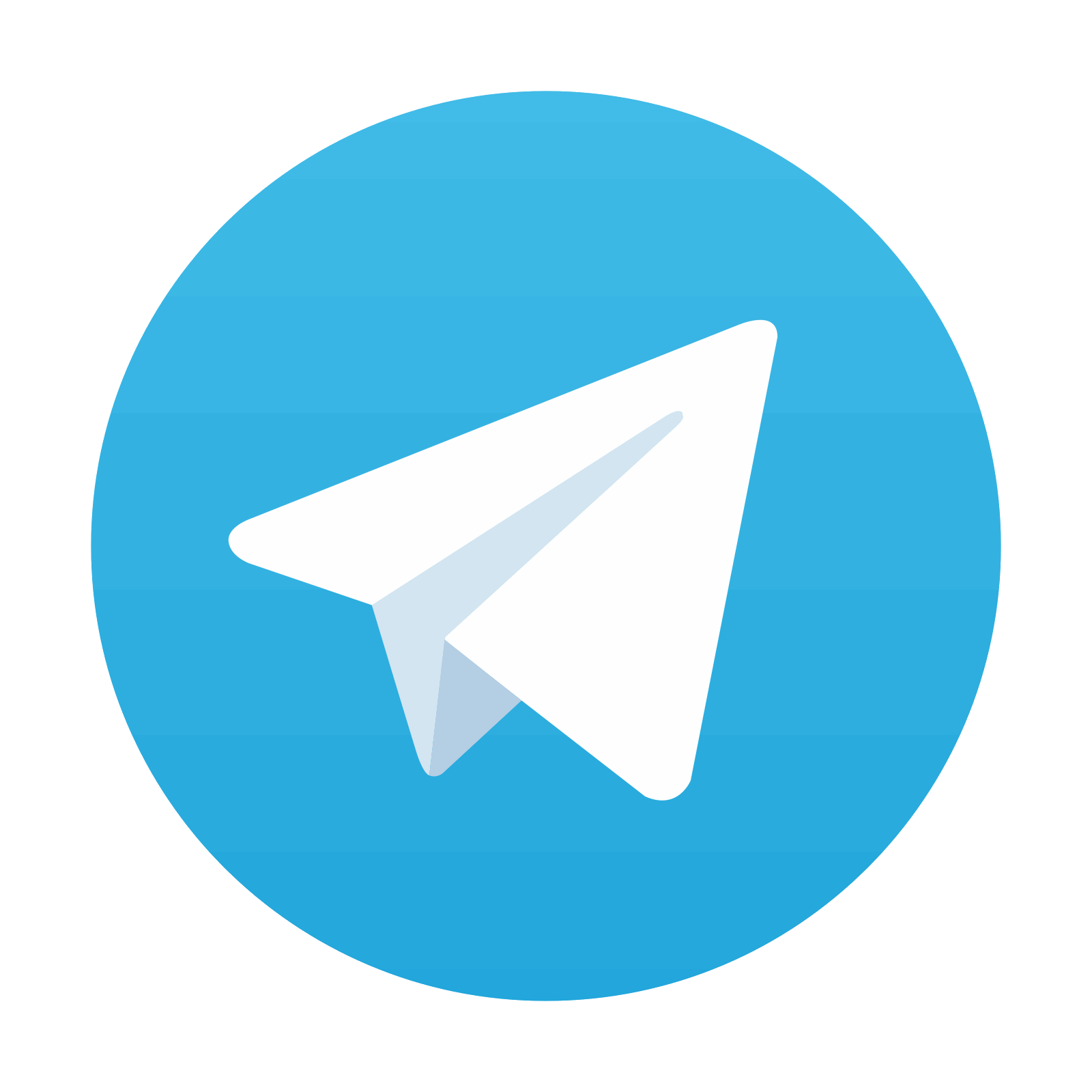
Stay updated, free articles. Join our Telegram channel

Full access? Get Clinical Tree
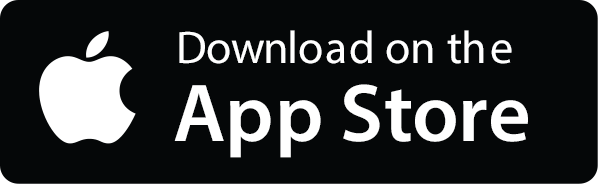
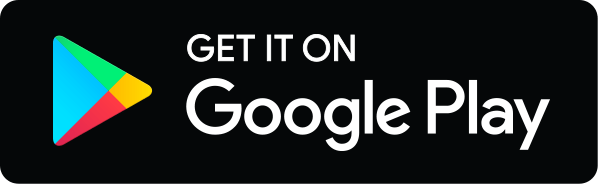