Abstract
Mitochondria are crucial organelles of eukaryotic aerobic cells (somatic and germ cells) because they produce adenosine triphosphate (ATP) and are modulators of ion homeostasis, generators of free radicals and regulators of cell death. Numerous studies reported that mitochondrial functionality was associated with sperm quality. Indeed, mitochondrial activities regulate many important sperm functions including sperm motility, hyperactivation, capacitation, acrosome reaction, and fertilization [1]. The exact mechanisms that link mitochondrial activities and sperm functions are often poorly understood or remain debated. Sperm mitochondria are arranged in the midpiece close to the flagellar. It seemed obvious that mitochondrial injuries can result in decreased sperm motility since motility is an ATP-dependent process, thus reliant on the energetic function of mitochondria for powering the flagellar motion. However, increasing evidence suggests that the dependence on mitochondria-derived ATP for human sperm motility is not unique and that glycolysis may replace mitochondrial oxidative phosphorylation [2]. Furthermore, spermatozoa can adapt their metabolic pathways depending on the availability of substrates. This is feasible since sperm mitochondria possess specific enzyme isoforms with distinct kinetics [2]. In addition to their role as an ATP producer, mitochondria regulate the lifespan of spermatozoa. Reduction in mitochondrial activities judged by the drop in the mitochondrial membrane potential (Δψm) has been regarded as an early cell death event [3]. The relevance of sperm mitochondria in fertility may also be associated with their role in the intermediate metabolism as producer of reactive oxygen species (ROS) or as regulator of intracellular calcium homeostasis, which are known to regulate proper sperm functions [4].
8.1 Introduction
8.1.1 Sperm Mitochondria and Male Fertility
Mitochondria are crucial organelles of eukaryotic aerobic cells (somatic and germ cells) because they produce adenosine triphosphate (ATP) and are modulators of ion homeostasis, generators of free radicals and regulators of cell death. Numerous studies reported that mitochondrial functionality was associated with sperm quality. Indeed, mitochondrial activities regulate many important sperm functions including sperm motility, hyperactivation, capacitation, acrosome reaction, and fertilization [1]. The exact mechanisms that link mitochondrial activities and sperm functions are often poorly understood or remain debated. Sperm mitochondria are arranged in the midpiece close to the flagellar. It seemed obvious that mitochondrial injuries can result in decreased sperm motility since motility is an ATP-dependent process, thus reliant on the energetic function of mitochondria for powering the flagellar motion. However, increasing evidence suggests that the dependence on mitochondria-derived ATP for human sperm motility is not unique and that glycolysis may replace mitochondrial oxidative phosphorylation [2]. Furthermore, spermatozoa can adapt their metabolic pathways depending on the availability of substrates. This is feasible since sperm mitochondria possess specific enzyme isoforms with distinct kinetics [2]. In addition to their role as an ATP producer, mitochondria regulate the lifespan of spermatozoa. Reduction in mitochondrial activities judged by the drop in the mitochondrial membrane potential (Δψm) has been regarded as an early cell death event [3]. The relevance of sperm mitochondria in fertility may also be associated with their role in the intermediate metabolism as producer of reactive oxygen species (ROS) or as regulator of intracellular calcium homeostasis, which are known to regulate proper sperm functions [4].
Irrespective of these mechanistic considerations, numerous clinical studies indicate that the determination of mitochondrial function constitutes a proper method for evaluating sperm quality and therefore male fertility.
8.1.2 Mitochondrial Functionality and the Inner Mitochondrial Membrane Potential (Δψm) in Spermatozoa
In the mitochondrial matrix, the oxidization of substrates leads to the transport of electrons along the electron transport chain complexes of the inner mitochondrial membrane resulting in the extrusion of protons across the inner membrane to generate the proton-motive force. The proton-motive force consists of two components, the transmembrane proton gradient (ΔpH) and the inner membrane potential (Δψm). The electrical gradient, Δψm (around 150 mV, negative inside) makes a larger contribution than the pH gradient (0.5 pH units, inside alkaline). The proton-motive force drives ATP synthesis. Thus, the Δψm is considered as the driving force for mitochondrial ATP production [5]. According to recent findings, Δψm also regulates other non-energetic mitochondrial functions including mitochondrial Ca2+ sequestration, ROS production, and regulation of cell death. Thus, the Δψm can be viewed as a general indicator of mitochondrial functionality, which reflects sperm cell health. That is why the assessment of mitochondrial functionality can be performed by estimating the Δψm in spermatozoa.
For over 30 years, many clinical and research studies have been published unveiling a link between Δψm and sperm motility (for recent review see [2]). One of the first publications reported a positive correlation between Δψm and sperm motility in ejaculates from patients with fertility problems [6]. This result was later validated in a larger cohort of infertile patients [7]. Moreover, it was demonstrated that the detection of Δψm is an effective test to assess sperm quality in the preparatory steps before IVF [7, 8, 9]. There is a significant correlation with Δψm in both neat semen and prepared sperm. Interestingly, Δψm can predict the success of an IVF [8, 9]. The limits in most of these related studies are the recruitment of infertile patients, constituting a selection bias. More recently, analysis of Δψm in men from the general population confirmed the previous data linking Δψm and sperm motility. Overall, these results underline the relevance of using Δψm as a mitochondrial biomarker in male fertility [10].
Interestingly, mitochondrial functionality is also involved in other steps that are required to render sperm competent to fertilize oocytes. Thus, mitochondrial functions are associated with sperm capacitation, sperm acrosin activity and the acrosome reaction [11]. Accordingly, using cell sorting showed that sperm mitochondria with high Δψm constituted a subpopulation prone to undergo calcium ionophore-induced acrosome reaction [12].
In conclusion, the determination of mitochondrial functionality by the assessment of Δψm provides useful information on the overall quality and the fertilizing capacities of human spermatozoa.
8.1.3 Methods to Detect the Inner Membrane Potential in Spermatozoa
The use of different fluorochromes on living spermatozoa allows the detection of Δψm by fluorometric methods including flow cytometry. These fluorochromes have in common the fact that they are lipophilic and cationic. These characteristics make them permeable to the hydrophobic plasma membrane and allow their accumulation into the mitochondrial matrix (cations are attracted to the negative potential) in viable cells depending on the value of the Δψm. According to the Nernst equation, there is a tenfold increase in the concentration of fluorochrome within mitochondrial matrix for every 61.5 mV increase in Δψm. For these reasons, fluorochromes that detect Δψm are known as potentiometric dyes and the fluorescence intensity of the accumulated fluorochromes is correlated to Δψm. Sperm mitochondria accumulating high concentrations of fluorochromes correspond to polarized mitochondria (with Δψm high) whereas mitochondria accumulating lower concentrations of fluorochromes are depolarized (and have a low Δψm) (see Figure 8.1). All of these probes need a minute attention to technical details and adequate controls to allow for a correct analysis. To ascertain that the fluorochrome behavior is related to Δψm in sperm cells, it is important to perform control experiments in which protonophores such as Cl-CCP and FCCP or the K+ ionophore valinomycin are used to depolarize the inner mitochondrial membranes. The incubation of sperm cells with Cl-CCP or valinomycin should result in a drop in the incorporation of the fluorochrome (about 1–1.5 log fluorescence). This highlights that the potentiometric dye incorporation is indeed driven by the mitochondrial membrane potential (see Figure 8.1).
Figure 8.1 Gating strategy and representative cytofluorometric histograms of spermatozoa stained with potentiometric dyes. Figure 8.1A: Left) SSC-A versus FSC-A density plot. Each dot represents an individual sperm cell that has passed through the laser. A gate has been applied to identify spermatozoa (Gate A), and to remove debris (on the lower left corner); (Figure 8.1A: Right). FSC-A versus FSC-W plot. This plot is used to discriminate doublets from single cells. Events out of the Gate B are considered as doublets; Figure 8.1B–D. Analysis of histograms of spermatozoa from one sample. Spermatozoa are stained with the following potentiometric dyes: DIOC6(3) (B), CMXRos (C) and CM-H2XRos (D). Unlabeled cells are represented by dark lines, labeled cells incubated with Cl-CCP as control by light gray profile and labeled cells without Cl-CCP by dark gray profile; Figure 8.1E–G. Two-parameter (dual color fluorescence) JC-1 dot plot. Unlabeled cells are represented in Figure 8.1E (as negative control), JC-1 stained cells in Figure 8.1F and JC-1 stained cells incubated with Cl-CCP (as positive control) in Figure 8.1G.
In past decades, we have observed the availability of new potentiometric dyes that allow for more reliable and accurate measurements of Δψm. Potential sensitive dyes are either rosamines, rhodamines or carbocyanine derivatives. The dyes’ behavior may be influenced by environmental events independent of the Δψm. For instance, some of the potentiometric dyes undergo self-quenching upon overaccumulation in the mitochondrial matrix distorting the Δψm detection. The other major problem of these fluorochromes is the occurrence of non-specific accumulation within spermatozoa independently of the Δψm. These issues must be taken into account when developing any new experiments and require the use of depolarization control (e.g. with Cl-CCP) for each experimental series (see protocol 8.2.3). The main potentiometric probes used in spermatozoa are listed in Table 8.1 with their specific fluorescence characteristics.
Table 8.1 Main characteristics of Δψm-sensitive probes
Probe name | Dye family | Maximum absorption | Laser | Maximum emission | Filter band pass | Estimated threshold for self quenching | Characteristics | Cautions |
---|---|---|---|---|---|---|---|---|
CMH2XRos | Rosamines | 579 nm | 561 nm | 585/15 | 610/20 | >100nm |
| |
CMXRos | ||||||||
JC-1 | Carbocyanines | 514 nm monomer form 585 nm J-aggregate form | 488nm | 529 nm monomer form 590 nm J-aggregate form | 525/30 610/20 | >2nm |
|
|
DIOC6(3) | 484 nm | 488nm | 501 nm | 525/30 | >40nm |
|
| |
TMRE | Rhodamines | 540 nm | 488 nm / 561 nm | 595 nm | 585/15 | >500nm |
|
|
TMRM | 548 nm | 574 nm | ||||||
Rhodamine 123 | 507 nm | 488nm | 529 nm | 525/30 | ND |
|
| |
Live/Dead blue | Amine fixation | 350 nm | 405 nm | 450 nm | 450/50 | ND |
|
|
Rhodamine 123 (Rh123) was the first fluorescent probe used to assess ∆ψm in spermatozoa [6] then widely used in other studies. However, the use of Rh123 is often delicate given major drawbacks such as the existence of non-specific Rh123-binding sites, a risk of self-quenching as well as high photo-instability [5]. For these reasons, we do not recommend its use especially for inexperienced technicians.
The carbocyanine derivative 3,3’-dihexyloxacarbocyanine iodide [DiOC6(3)] was extensively used to detect mitochondrial membrane depolarization preceding nuclear apoptosis. DiOC6(3) bears the major advantage of having a limited self-quenching effect. However, some disadvantages with the use of DiOC6(3) staining were reported at high concentrations such as a mitochondrial uptake also dependent on the plasma membrane potential and a lack of mitochondrial specificity [5]. Thus, DiOC6(3) is also a valuable dye to assess Δψm by flow cytometry in sperm samples when used in low concentrations (<40 nM) [7, 8].
Several potentiometric dyes, including the rhodamine derivatives tetramethylrhodamine ethyl ester (TMRE) and tetramethylrhodamine methyl ester (TMRM), have been developed in the past decades. There are also potential problems with TMRM/TMRE, specifically self-quenching when used at high concentrations [5]. Nevertheless, TMRM/TMRE represent one of the most efficient fluorochromes for Δψm measurements in living cells including spermatozoa [8]. Particularly, the analysis of TMRE/TMRM staining is uncomplicated compared to the analysis of JC-1, which emits a double fluorescence that could be affected by potential-independent parameters.
Chloromethyl-X-rosamine (CMXRos) dye (AKA MitoTrackerRed) and its reduced form CM-H2XRos are more photostable than Rh123 and were suggested to accurately evaluate changes in Δψm [8]. CMXRos contains a thiol-reactive chloromethyl moiety that reacts with mitochondrial thiol groups and therefore CMXRos yields a potential advantage of being fixable [13]. However, the fixation step can potentially disturb CMXRos staining and we recommend comparing results with and without fixation to unsure that CMXRos remains a reliable marker of Δψm after sperm fixation with formaldehyde. More recently, a series of chloromethyltetramethylrosamine analogs (MitoTracker dyes) that differ in their excitation/emission wavelengths were introduced as new potentiometric probes. However, their use in sperm samples has yet to be fully validated.
In clinical studies, the carbocyanine fluorescent probe 5,5’ ,6,6’-tetrachloro-1,1’ ,3,3’-tetraethylbenzimidazolyl-carbocyanine iodide (JC-1) was extensively used to accurately evaluate changes in Δψm [14, 15]. JC-1 selectively labels sperm mitochondria depending on the Δψm and is considered as one of the most sensitive and specific fluorochrome for the detection of Δψm. Unlike other potentiometric dyes, after excitation at 488 nm, JC-1 emits fluorescence in two different wavelengths according to its aggregation state. Cells with high Δψm accumulate in their mitochondrial matrix high concentrations of JC-1 that aggregate and fluoresce orange/red (590 nm) while cells with low Δψm contain less JC-1 that stay at a monomeric state and fluoresce green (527 nm). Due to its dual emission wavelengths, JC-1 has been labeled as more reliable than other potentiometric dyes for monitoring ∆ψm. Typically, Δψm depolarization leads to a shift from red to green fluorescence. However, the intensity of the green fluorescence emitted by the JC-1 monomer form can be dependent on ∆ψm-insensitive parameters. Furthermore, the presence of antioxidants provides unreliable results in human sperm labeled with JC-1 [16]. In spermatozoa, dissipation of Δψm with valinomycin or Cl-CCP used as control (see protocol) does not always increase the green fluorescence. Thus, in our study we found that it is better to rely on the red-orange fluorescence emission of JC-1 aggregates to assess changes in Δψm in sperm samples [8]. Interestingly, depending on sperm samples, the resolution between the two fluorescence peaks (Δψm low and Δψm high) of JC-1 aggregates can be weak thus rending the analysis of JC-1 aggregate histograms more subjective [8]. Subsequently, the analysis of the percentage of Δψm high-stained with JC-1 can be more subjective than with other potentiometric dyes. For these reasons, sperm staining with potentiometric dyes that emit in single-fluorescence should be preferred to the use of JC-1.
In conclusion, fluorochromes TMRM/TMRE, CMXRos, DiOC6(3) and JC-1 are adequate for reliable ∆ψm evaluation in sperm samples. All of these fluorochromes stain mitochondria of live spermatozoa and allow for accurate cytofluorometric analysis. CMXRos is potentially fixable. TMRM/TMRE is simple to use and can accurately monitor Δψm without JC-1 limitations. The choice of the fluochrome will depend on their fluorescence spectra in order to use them in combination with other fluorescent markers.
8.1.4 Flow Cytometric Detection of the Inner Membrane Potential in Spermatozoa
Flow cytometry constitutes a powerful semi-quantitative method to investigate Δψm in spermatozoa. One of the main advantages of flow cytometry is the large number of spermatozoa (thousands of cells) that can be estimated in a few seconds thus increasing the reliability of the results. Flow cytometric Δψm assays can be of great interest in the assisted reproductive technology (ART) laboratory. Their automatic analysis can rapidly determine sperm quality with great accuracy. Flow cytometric Δψm assays give reliable information on sperm cell populations and thus could represent an interesting alternative to the visual estimation of motility, which is particularly time-consuming and remains subjective.
Flow cytometry is especially suited to detect heterogeneity within the sperm cell population. Flow cytometric Δψm determination can be used to discriminate sperm cells with active (Δψm high) and non-active (Δψm low) mitochondria. Flow cytometric analysis of spermatozoa stained with the fluorochromes described above revealed in each case two cellular subpopulations corresponding to two fluorescence peaks with different intensities (see Figure 8.1). One subset of spermatozoa that incorporates more fluorochrome (about 1–1.5 log more) corresponds to spermatozoa with high Δψm (Δψm high cells). The other subset of spermatozoa is less fluorescent and corresponds to Δψm low spermatozoa.
Flow cytometry also allows for the simultaneous evaluation of Δψm in individual spermatozoa along with other sperm parameters. This is why it is important to have a large panel of reliable potentiometric probes emitting in different fluorescence channels, thus increasing the choice of excitation or emission wavelengths. These potentiometric dyes such as CMXRos, DiOC6(3), TMRE/TMRM emit single fluorescent peak and can be used simultaneously in combination with other fluorochromes evaluating other sperm features. JC-1 because of its dual emission wavelengths is much more difficult to use in combination with other dyes in a single laser cytometer. Since they emit a single fluorescence peak, CMXRos, DiOC6(3) and TMRE allow the combination with different supravital fluorochromes. Here, we developed a double-staining protocol dedicated to the simultaneous evaluation of Δψm and cell viability after staining with CMXRos and Live/Dead blue, respectively (see protocol 8.2.3.5).
Interestingly, flow cytometry can be proposed to detect Δψm in conjunction with the acrosome reaction in spermatozoa. Evaluation of acrosome membrane integrity constitutes an important parameter to evaluate semen quality and male fertility. Several fluorescence techniques are available in flow cytometry to determine acrosome integrity. Spontaneous acrosome reaction (acrosomal status) can be assessed by using fluorescein isothiocyanate conjugate–anti CD46 monoclonal antibody [12] or Lectin-FITC staining [17]. In these conditions, acrosome reaction and the Δψm can be measured simultaneously using Lectin-FITC and chloromethyl-X-rosamine (CMXRos) staining, respectively (see protocol).
Overall, the simultaneous detection of several sperm parameters on individual spermatozoa contributes to a better estimation of the fertilizing potential of semen samples.
This chapter gives essential information about flow cytometric methods used for the appropriate assessment of Δψm in spermatozoa. Some details and critical steps are presented to highlight the risks of pitfalls in Δψm determination.
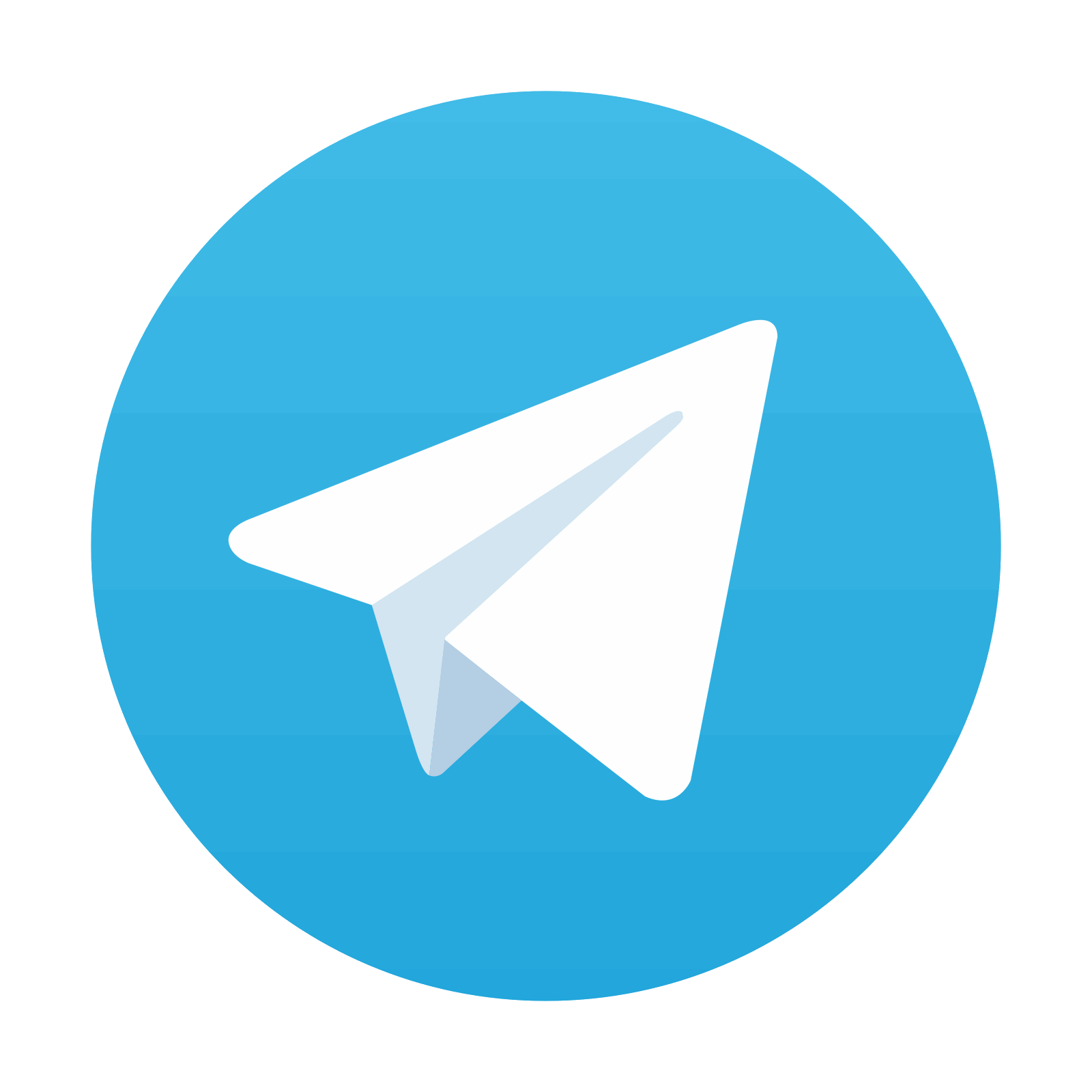
Stay updated, free articles. Join our Telegram channel

Full access? Get Clinical Tree
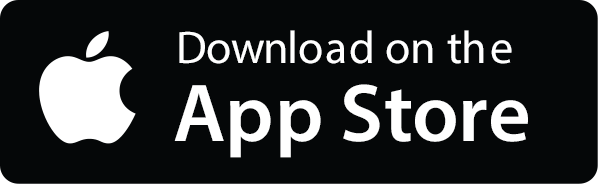
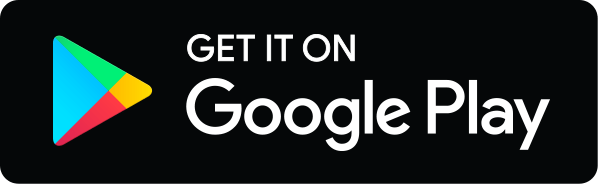
