Abstract
Spermatozoa, one of the two most pivotal cells of biological existence, are responsible for mediating the transfer of genetic information to subsequent generations. Mammalian fertilization and subsequent embryonic development depend in part on the inherent integrity of sperm genome. Different fertility societies around the globe and the World Health Organization (WHO) estimate that infertility is present in between 7 and 15 percent of couples of reproductive age [1].
16.1 Introduction
Spermatozoa, one of the two most pivotal cells of biological existence, are responsible for mediating the transfer of genetic information to subsequent generations. Mammalian fertilization and subsequent embryonic development depend in part on the inherent integrity of sperm genome. Different fertility societies around the globe and the World Health Organization (WHO) estimate that infertility is present in between 7 and 15 percent of couples of reproductive age [1].
The current diagnosis of male infertility is based upon semen analysis, which encompasses the traditional semen parameters, semen volume and sperm concentration, motility and morphology as a gold standard. However, it has become apparent that none of these parameters recommended by the World Health Organization (WHO) is sufficient for the prediction of male fertility capacity. As the WHO parameters only address a few aspects of sperm quality and function, the discriminative power in relation to fertility is quite low [2, 3]. Although there is a direct relationship between semen quality and pregnancy rates, both in spontaneous and assisted conceptions, there is still no definite predictive threshold for success for conventional semen parameters. Conventional semen analysis does not assess all aspects of testicular function and sperm quality. New tests for predicting the chance of pregnancy would be clinically useful. Over the past two decades, sperm DNA testing has been hailed as a more promising method for fertility evaluation [4, 5, 6]. There have been attempts to propose sperm DNA fragmentation as such a new test for male reproductive capability [6, 7].
Sperm cells are remarkably complex and highly specialized cells as compared to somatic cells. Their function is to deliver the paternal genomic blueprint and a highly specialized epigenome along with a pool of proteins and coding and non-coding RNAs to the oocyte. Reproductive success, including optimal embryonic development and healthy offspring, greatly depends on the integrity of the sperm chromatin structure, its genome and epigenome. It is now well documented that DNA damage in sperm is linked to reproductive failures both in natural and assisted conception [8, 9] and is a major cause of defective sperm function and couples opting for assisted conception. Sperm DNA damage is also the underlying etiology of idiopathic recurrent pregnancy losses, idiopathic recurrent congenital malformations and even childhood cancers [10, 11]. The association between DNA damage and diminished reproductive outcomes has led to the introduction of sperm DNA integrity testing into the clinical assessment of male infertility.
There is a growing understanding about the importance of sperm DNA integrity on embryo development and the health of the offspring. Therefore, the purpose of this book chapter is to understand the structure of mammalian sperm chromatin and its impact on the rapidly advancing postgenomic era.
This essay will also evaluate the molecular techniques used for study of sperm DNA fragmentation with a detailed overview of the Aniline Blue staining method.
16.2 Sperm Nuclear Chromatin
The function of sperm is to safely transport the haploid paternal genome to the egg containing the maternal genome. Before the sperm can set out on its adventurous journey, remarkable arrangements need to be made during the post-meiotic stages of spermatogenesis [12]. Spermatogenesis, a continuous and highly conserved process, leads to the formation of haploid sperm cells capable of fertilization. It has been characteristically divided into three critical phases: a) mitotic amplification phase causing proliferation of spermatogonia; b) meiotic phase, where spermatogonia develop into primary and secondary spermatocytes; and c) post-meiotic phase which is also known as spermiogenesis where spermatids differentiate into spermatozoa. The developing germ cells in the post-meiotic phase are further subdivided into early spermatids with round nuclei, intermediate spermatids with elongated nuclei, and spermatids with condensed nuclei [13]. The essential hallmark of spermiogenesis is the replacement of nuclear somatic-cell like histones by small basic proteins known as protamines. This facilitates the condensation/compaction of sperm nucleus and consequently the sperm head. This condensation of the paternal genome in the human spermatozoa is specific to the sperm cell in order to protect the DNA during the transit from the male to the oocyte prior to fertilization [14]. The nuclear proteins found in the sperm form a distinct chromatin structure that is unlike any other cell type and is perfectly suited to support the male gamete [14, 15].
Spermatogenesis is also marked by a shutdown of transcriptional machinery at a defined point of germ cell differentiation [10, 11, 15, 16, 17, 18, 19]. Thus, the resulting translational repression, and storage of long-lived mRNAs, for example those which encode protamines necessary for completion of spermiogenesis and others which are necessary for fertilization and early embryonic development. Mammalian sperm chromatin can be divided into three major structural domains: (1) the vast majority of sperm DNA is coiled into toroids by protamines, (2) a much smaller percent remains bound to histones [20], and (3) the DNA is attached to the sperm nuclear matrix at MARs (matrix attachment regions) at medium intervals of roughly 50 kb throughout the genome [14].
16.2.1 Histones to Protamines: An Overview
Haploid spermatids undergo extensive morphological changes, including a striking reorganization and compaction of their chromatin [12]. Thereby, the nucleosomal histone-based structure is nearly completely substituted by a protamine-based structure. Protamines are the most abundant nuclear proteins found in sperm and are unique to sperm cells. These proteins have a strong positive charge due to their high arginine content, which helps facilitate their function [11, 17, 18, 19]. The high arginine content facilitates strong DNA binding, while the cysteines in protamines promote the formation of inter- and intra-protamine disulphide bonds during the process of compaction [12, 21]. The vast majority of the sperm chromatin is thus compacted into toroids containing roughly 50 kb of DNA [14]. During the process of spermatogenesis, protamines replace 85–95 percent of histones in the sperm, including both canonical histones and testicular histone variants, via a stepwise process. First, transition proteins, comprising both transition proteins 1 and 2 (T1 and T2), replace histone proteins that are DNA bound. Second, T1 and T2 are replaced by protamine proteins; protamine 1 and protamine 2 (P1 and P2). The ratio of P1:P2 is approximately 1:1 in most fertile humans [17].
After the incorporation of protamines into the paternal chromatin, cysteine residues between protamine molecules form intermolecular disulfide bridges as the cell matures. The strong positive charge of P1 and P2 as well as the formation of disulfide bridges produces a tightly condensed chromatin structure [15, 22]. The compaction of the sperm DNA into a nucleoprotamine complex results in approximately 6–20 times denser structure than the nucleosome-bound chromatin seen in somatic cells [15, 17, 20]. This condensation aids in two important roles: a) sperm motility relies chiefly on condensed nuclear structure as a decondensed sperm head may mechanically inhibit or perturb the cell’s potential for motility [23], b) prevents the sperm from impending DNA damage resulting from transit through the male and female reproductive tracts and exposure to oxidative stress due to genital tract inflammation, varicocele, and testicular hyperthermia prior to fertilization, as sperm lacks DNA repair mechanisms [17]. The replacement of histones during spermatogenesis is an incomplete process, as approximately 5–15 percent of the genome remain bound by nucleosomes and is not condensed into a nucleoprotamine complex [22]. This retention of nucleosomes in the peripheral compartment of the sperm genome includes telomeres and genes regulating early embryonic development. The nucleohistone compartment is vulnerable to oxidative stress and also facilitates the maintainance of genomic imprints [11, 20]. The replacement of histones is likely facilitated by incorporation of histone variants, post-translational histone modifications, chromatin-remodeling complexes, as well as transient DNA strand breaks.
16.2.2 Resiliences and Vulnerabilities
Sperm DNA damage has been documented as one of the major factors for defective sperm function. DNA damage includes DNA denaturation and sperm DNA fragmentation (SDF) and may be the common underlying etiology of infertility, recurrent pregnancy loss, pre- and post-implantation losses, accelerated aging, and childhood carcinomas [11, 24]. Oxidative stress is one of the leading causes of DNA damage and this can be determined by quantifying the levels of oxidative DNA adducts like 8-OHdG, a base which induces both mutations and epimutations. Sperm nuclear DNA fragmentation has been positively correlated with lower fertilization rates in IVF, impaired implantation rates, an increased incidence of abortion and disease in offspring, including childhood cancer. Abnormalities in chromatin condensation can cause nuclear damage as DNA denaturation or fragmentation are often associated with male infertility [25].
16.3 Sperm Chromatin Assessment
There has been an increase in the use of sperm DNA and chromatin integrity tests in the evaluation of the infertile man with the hypothesis that these tests may better diagnose infertility and predict reproductive outcomes. The evaluation of sperm chromatin and DNA structure was initially undertaken to improve our understanding of spermatogenesis, sperm physiology, sensitivity to reproductive toxicants and reproductive biology.
It has been suggested that protamine deficiency (with consequent aberrant chromatin remodeling), reactive oxygen species and abortive apoptosis may be responsible for sperm DNA damage [26, 27].
16.4 Various Techniques for Sperm Chromatin Assessment
There are two types of assays that have been developed to measure sperm DNA integrity: a) those that can directly measure the extent of DNA fragmentation through the use of probes and dyes and b) those that measure the susceptibility of DNA to denaturation, which occurs more commonly in fragmented DNA. Various methods to assess sperm DNA fragmentation are briefly described below and summarized in Table 16.1. The most commonly used tests are terminal deoxynucleotidyltransferase UTP nick end labeling (TUNEL), the sperm chromatin dispersion test (SCD), and the sperm chromatin structure assay (SCSA®).
Table 16.1 Sperm chromatin assessment methods
S.NO. | TEST | PRINCIPLE | MEASUREMET/ DETECTION | MERITS | DEMERITS |
---|---|---|---|---|---|
1. | AO Test | Detects metachromatic shift in fluorescence of AO, uses fluorescence microscopy | Single-stranded DNA breaks | Biologically stable measure of sperm quality Technique highly reproducible | Expensive instrumentation Observer subjectivity hinders the result if fluorescent microscopy used |
2. | TB Staining | Increased affinity of TB to sperm DNA phosphate residues, uses optical microscopy | Single- and Double-stranded DNA breaks | Simple and inexpensive Permanent preparations for use under ordinary microscope Morphological assessment of cells done | Limited number of cells can be reasonably scored Limits of repeatabilit |
3. | CMA3 Staining | Competitively binds to DNA, uses fluorescent microscopy | Indirectly assesses protamine deficient DNA | Reliable Sensitivity 73%, specificity 75% Strong correlation with other assays | Inter-observer variability |
4. | TUNEL Assay | Direct assay, done by optical microscopy and fluorescent microscopy | Direct quantification of enzymatic incorporation of dUTP into single- and double-stranded DNA breaks | Sensitive, reliable Intraobserver variability <8%, Interobserver variability <7% | Sophisticated and expensive Requires standarization between different laboratories |
5. | Comet Assay | Direct assay, electrophoretic assessment of DNA fragments. Done by optical or fluorescent microscopy | Double-stranded DNA breaks | Simple to perform, low performance cost. Well standarized assay Low intra assay coefficient of variation | Requires experienced observer to analyse and interpretation of results |
6. | ISNT | Direct assay, template-dependent DNA polymerase I, Requires fluorescent microscope | Single-stranded DNA breaks, Incorporates biotinylated dUTP with template-dependent DNA polymerase I | Reaction based on direct labelling of terminal DNA breaks Defects identifiable at molecular level | Less sensitive |
7. | SCSA | Indirect assay based on flow cytometry, Intercalation of acridine orange dye | Susceptibility of sperm DNA to acid induced conformational transition in situ. | Accurately assess percentage of sperm DNA damage Also gives the percentage of high density staining sperm indicative of immature chromatin | Requires expensive instrumentation and skilled technicians. |
8. | SCD/ Halo test | Indirect assay, based on the characteristic halo produced by the sperm after denaturation (not produced by sperm with fragmented DNA) | Sperm DNA fragmentation (single stranded DNA fragments) | Simple, fast and reproducible Doesn’t require the determination of color or fluorescence | Inter-observer variability |
9. | Nuclear protein composition | Indirect assay, protein extraction, and immunoblotting with specific antibodies | Protamine-to-histone ratio | Specific, sensitive, | Requires experienced person to conduct and interpret the results |
10. | Sperm nuclear maturity test | Indirect assay, slide based quantification | Sperm DNA integrity Protamine composition | Simple and inexpensive | Inter-observer variability |
1) Acridine Orange (AO) Test- Green fluorescence (normal DNA), red fluorescence (denatured DNA), 2) Toluidine Blue (TB) staining- Light blue (normal sperm), violet (sperm with DNA fragmentation), 3) Chromomycin A3 (CMA3)- Bright yellow (protamine deficient sperm), yellowish green (normal protamine content), 4) Terminal deoxynucleotidyl transferase dUTP nick end labeling (TUNEL)- fluorescent activated cell sorting histogram, 5) Comet- migration of fragmented DNA appreciate as tail, intact DNA remains as head, 6) In situ nick translation (ISNT)- measures the number of fluorescent sperm which incorporate dUTP, 7) Sperm chromatin structure assay (SCSA)- AO staining assessed by flow cytometry, measures metachromatic shift of AO fluorescence from green to red, 8) Sperm chromatin dispersion (SCD)- assesses sperm with different DNA dispersion, assesses large, medium or small sized halo.
16.5 Aniline Blue Staining
Aniline blue (AB) staining is a histochemical technique that depends on the use of dyes. AB is an acidic dye that has a great affinity for lysine-rich histones in the nucleus of immature sperm, which stain blue.
16.5.1 Background
The chromatin of mature spermatozoa possesses a varying binding capacity for nuclear dyes and stains. The sperm nuclear condensation or maturation can be assessed by the AB test. AB, an acidic dye, has a greater permeability/affinity for proteins in the loose chromatin of the sperm nucleus. This is due to the presence of the residual histones and increased accessibility of the basic groups of the nucleoprotein. Increased AB staining of sperm indicates loose chromatin packing.
Abundant lysine is present in the histone-rich nuclei of immature spermatozoa, which takes up the AB stain, whereas arginine and cysteine present in protamine-rich spermatozoa of mature spermatozoa remain unstained [6, 25, 28, 29]. Thus, this test differentiates between the lysine-rich histones from the arginine- and cysteine-rich protamines. Detection of extra lysine-rich histones indicates lower amounts of protamines in the sperm nucleus and immature chromatin condensation [21, 30]. Infertile males with lower protamine levels show lower sperm counts, motility and abnormal morphology and a higher predisposition to DNA damage [6, 21, 24]. The procedure for the acidic AB staining has been summarized in Figure 16.1 and is based on the protocol previously explained [31].
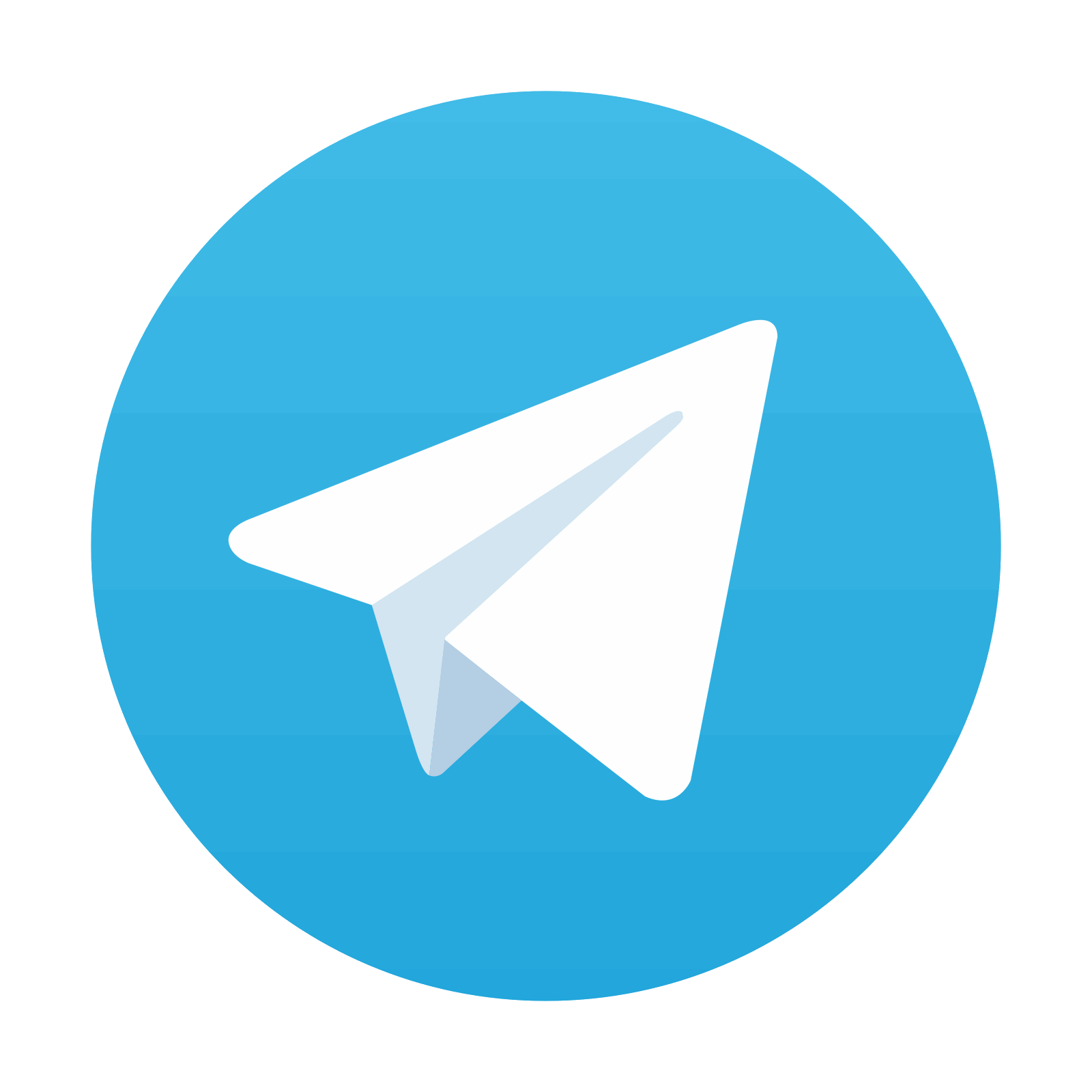
Stay updated, free articles. Join our Telegram channel

Full access? Get Clinical Tree
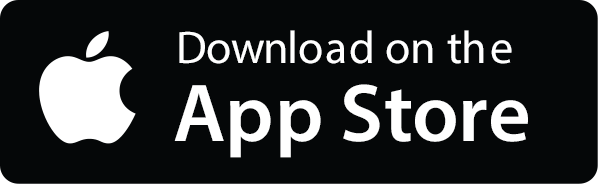
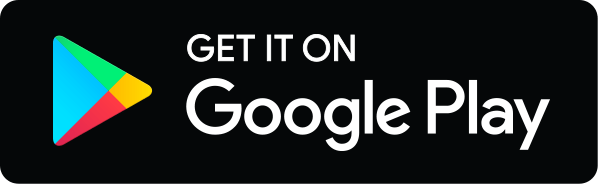
