Abstract
Fertilization is an exceptionally specific cell recognition event that represents the culmination of a complex sequence of morphological and functional maturational events. In the case of the male gamete, this process is initiated by the commitment of spermatogonial stem cells to differentiate, sequentially forming spermatogonia, spermatocytes and eventually spermatozoa that are released into the lumen of the seminiferous tubules [1]. In addition to meiotic divisions, this process encompasses extensive cytoplasmic, organelle and nuclear remodeling events, thus establishing the unique and highly polarized architecture of the mature spermatozoon. A key aspect of this phase of development is the modification and repositioning of the Golgi apparatus to form a highly specialized secretory organelle, known as the acrosome, overlying the anterior aspect of the sperm head. Upon release from the testes the functionally immature spermatozoa enter the epididymis where they are progressively remodeled and acquire both motility and the potential to fertilize an oocyte [2]. This potential is eventually realized after passage through the female reproductive tract whereupon the ejaculated cells complete a suite of biochemical and biophysical changes known as capacitation [3]. These successive phases of functional maturation culminate in the acquired ability to release the acrosomal contents, during an event known as the acrosome reaction. This unique exocytotic event facilitates sperm passage through the outer vestments of the oocyte and is essential for successful in vivo fertilization in all mammalian species, including the human [4]. Consequently, failure of acrosomal exocytosis represents a common etiology in defective spermatozoa of male infertility patients that have failed in vitro fertilization (IVF) in a clinical setting; accounting for as much as 29 percent of unexplained male infertility cases [5, 6]. Much of our current mechanistic understanding of the acrosome reaction is grounded in the ability to stimulate this process in vitro using simple chemically defined media and the application of pharmacological interventions, and/or transgenic mouse models. Here, we discuss the biological significance of the acrosome reaction and the application of histochemical techniques that have been developed to study the progression and completion of this critical physiological event.
10.1 Introduction
Fertilization is an exceptionally specific cell recognition event that represents the culmination of a complex sequence of morphological and functional maturational events. In the case of the male gamete, this process is initiated by the commitment of spermatogonial stem cells to differentiate, sequentially forming spermatogonia, spermatocytes and eventually spermatozoa that are released into the lumen of the seminiferous tubules [1]. In addition to meiotic divisions, this process encompasses extensive cytoplasmic, organelle and nuclear remodeling events, thus establishing the unique and highly polarized architecture of the mature spermatozoon. A key aspect of this phase of development is the modification and repositioning of the Golgi apparatus to form a highly specialized secretory organelle, known as the acrosome, overlying the anterior aspect of the sperm head. Upon release from the testes the functionally immature spermatozoa enter the epididymis where they are progressively remodeled and acquire both motility and the potential to fertilize an oocyte [2]. This potential is eventually realized after passage through the female reproductive tract whereupon the ejaculated cells complete a suite of biochemical and biophysical changes known as capacitation [3]. These successive phases of functional maturation culminate in the acquired ability to release the acrosomal contents, during an event known as the acrosome reaction. This unique exocytotic event facilitates sperm passage through the outer vestments of the oocyte and is essential for successful in vivo fertilization in all mammalian species, including the human [4]. Consequently, failure of acrosomal exocytosis represents a common etiology in defective spermatozoa of male infertility patients that have failed in vitro fertilization (IVF) in a clinical setting; accounting for as much as 29 percent of unexplained male infertility cases [5, 6]. Much of our current mechanistic understanding of the acrosome reaction is grounded in the ability to stimulate this process in vitro using simple chemically defined media and the application of pharmacological interventions, and/or transgenic mouse models. Here, we discuss the biological significance of the acrosome reaction and the application of histochemical techniques that have been developed to study the progression and completion of this critical physiological event.
10.1.1 The Sperm Acrosome
10.1.1.1 Biogenesis and Structure of the Acrosomal Vesicle
The term “acrosome” was introduced by Lenhossek in 1898 and literally translates as “tip body” or “apical body”. Over time, we have come to appreciate that the acrosome is a discrete lysosome-like membranous organelle that is indeed positioned overlying the nucleus within the anterior region of the sperm head. The acrosome is unique to the male gamete and, notwithstanding considerable variation in size and shape between species, this organelle originates from the Golgi apparatus during the remodeling of early spermatids that accompanies spermiogenesis (Figure 10.1) [1]. Acrosomal biogenesis is initiated by the formation of pro-acrosomic vesicles that are generated within, and actively trafficked by, the Golgi apparatus. These entities eventually fuse giving rise to a single, dense, acrosomal vesicle that extends to cover approximately two-thirds of the nuclear surface [1]. This structure is delimited by both inner and outer acrosomal membranes, and, through its association with the overlying acroplaxone (consisting of actin and keratin fibers) and manchette complex, the acrosomal vesicle becomes anchored to the nuclear envelope and participates in the sculpting of the sperm head during spermiogenesis [1].
Figure 10.1 Biogenesis of the human sperm acrosome. Schematic diagram depicting the cytodifferentiation of the developing germ cell during spermiogenesis. Formation of the acrosomal vesicle is initiated in early stage spermatids as pro-acrosomic vesicles generated by the Golgi apparatus fuse giving rise to a single, highly specialized secretory organelle that extends to cover approximately two-thirds of the nuclear surface.
In the mature spermatozoon, the acrosomal vesicle incorporates a myriad of structural and enzymatic components that are compartmentalized into either soluble or insoluble fractions. The latter of these consists of particulate material termed the acrosomal matrix; a structure that resists detergent solubilization and has properties consistent with those of functional amyloids [7]. Indeed, the core of the acrosomal matrix is known to comprise several amyloidogenic proteins, as well as other proteins predicted to self-assemble into amyloid [7]. Accordingly, the acrosomal matrix has been postulated to function as a stable scaffold for interactions between the sperm and oocyte and to allow the controlled and sequential release of matrix associated proteins necessary for fertilization during the acrosome reaction. Thereafter, proteases activated by the increased intra-acrosomal pH that results from the induction of the acrosome reaction have been postulated to promote the disassembly of the acrosomal matrix, culminating in the complete loss of the acrosomal cap [7].
Owing to its essential role during fertilization, considerable effort has been devoted to the characterization of the acrosomal contents. Among the dominant proteins identified by biochemical means residing in the acrosomal matrix are acrosin, acrosin binding protein, zona pellucida binding protein, zona pellucida-3 receptor, and zonadhesin. However, the recent application of advanced mass spectrometry analyses has expanded the curated inventory of acrosomal matrix associated proteins to excess of 1,000 candidates [8]. Notably, approximately half of the proteins represented in the acrosomal matrix had not been previously identified in proteomic analyses of spermatozoa, suggesting that the composition of this organelle differs substantially from that of other sperm domains [8]. Moreover, the acrosomal matrix is enriched in proteins classified as having cytoskeletal, oxidoreductase, and hydrolase functions, as well as ancillary proteins categorized as enzyme modulators, proteases, transporters, transferases, nucleic acid binding proteins, chaperones, transfer/carrier proteins, structural proteins, and kinases. Of interest, the proteomic composition of the acrosomal matrix differs between the populations of sperm entering (i.e. caput spermatozoa) and leaving the epididymis (i.e. cauda spermatozoa) raising the prospect that this specialized vesicle may undergo maturational changes during epididymal transit in preparation for downstream functions during fertilization [8]. These findings confirm that the acrosome is a dynamic functional structure comprising an abundance of fertilization-associated proteins; many of which have the potential to be exploited as biomarkers capable of discriminating the acrosomal status and/or fertilization competence of human spermatozoa [9].
10.1.1.2 Physiology of the Acrosome Reaction
In all mammalian species including the human, the completion of an acrosome reaction is a prerequisite for successful fertilization in vivo [10]. Indeed, the importance of the acrosome for human fertilization is evidenced by a rare congenital condition in which spermatozoa are produced with a small spherical head lacking an acrosome [11]. These acrosome-less spermatozoa are unable to bind to or penetrate the zona pellucida (ZP) and accordingly, men with this condition are universally sterile and unable to produce pregnancies via natural conception or standard IVF procedures [4]. This condition can be treated by intracytoplasmic sperm injection (ICSI) [11], albeit with variable success in terms of fertilization rates and pregnancies; depending on whether or not the sperm heads are capable of decondensing normally within the ooplasm [12]. Similarly, the inability to complete an acrosome reaction represents a relatively common etiology in the defective spermatozoa of idiopathic male infertility patients that have failed standard IVF [6], accounting for as much as 29 percent of unexplained male infertility cases [5]. Such defects often manifest in a failure of sperm-zona pellucida penetration, occurring downstream of zona pellucida binding; a condition referred to as disordered zona pellucida-induced acrosome reaction (DZPIAR), and one that has been associated with severe chronic infertility [4]. It follows that the proportion of sperm with intact acrosomes in the insemination medium is positively correlated with fertilization rates in vitro, thus emphasizing the utility of diagnostic assays that enable determination of sperm competence to complete an acrosome reaction before commencing assisted reproductive technologies (ART).
The acrosome reaction is a distinctive exocytotic process that is initiated by multiple fusion events and subsequent vesiculation occurring between the outer acrosomal membrane and the overlying plasma membrane (Figure 10.2). As this wave of vesiculation radiates across the anterior region of the sperm head, it leads to the destabilization of the acrosomal structure, formation of hybrid membrane vesicles and the concomitant release of the soluble acrosomal contents [10]. The dispersion of the acrosomal contents, which includes a number of powerful hydrolytic enzymes, enables sperm penetration of the tenacious investments surrounding the ovulated oocyte. This, in turn, provides the fertilizing spermatozoon with access to the oocyte plasma membrane beneath, and ultimately secures cytoplasmic continuity between the gametes. Recent work has drawn into question the long-held view that the acrosome reaction proceeds in an “all or none” manner, with accumulating evidence suggesting that the process may instead comprise several intermediate phases, each with their own set of functional consequences [13]. In keeping with this model, several features of acrosomal exocytosis, including the relatively slow kinetics at which it proceeds, underscore the highly specialized nature of this secretory process and the prospect that is governed by complex molecular mechanisms [13].
Figure 10.2 Human sperm acrosome reaction. Schematic diagram depicting the progression of the acrosome reaction within the head of a mature human spermatozoon. After initiation of the acrosome reaction, multiple fusion foci form between the outer acrosomal membrane and overlying plasma membrane. As the acrosome reaction proceeds a wave of vesiculation propagates across the anterior region of the sperm head, facilitating the loss of the acrosomal contents and exposure of the inner acrosomal membrane; except in the region of the equatorial segment where the outer acrosomal membrane and the plasma membrane retain their integrity.
The ability to undergo a physiological acrosome reaction necessitates that spermatozoa have first completed a process of functional maturation, known as capacitation, during their transit of the female reproductive tract [13]. Among the many biochemical and biophysical changes that accompany capacitation, the sperm membrane becomes increasingly fusogenic, owing to alterations in its lipid architecture and the release of decapacitation factors [14]. These combined events serve to modulate intracellular ion concentrations leading to hyperpolarization of the sperm plasma membrane and triggering complex signaling cascades that prime the cell for completion of an acrosome reaction. The precise physiological stimulus that acts downstream of capacitation to induce acrosomal exocytosis remains a matter of some controversy [15]. Indeed, notwithstanding a wealth of evidence that adhesion to zona pellucida ligands act as the key stimulus for induction of acrosomal exocytosis, particularly in the human, alternative models based on studies of transgenic mice now suggest that the timing of the acrosomal exocytosis may actually precede that of zona adhesion [15]. Consistent with this notion, it has been shown that a significant proportion of mouse spermatozoa initiate acrosomal exocytosis in the upper segments of the oviductal isthmus [16], prior to engaging zona pellucida ligands. Moreover, mouse spermatozoa that have undergone an acrosome reaction retain the ability to bind and penetrate the zona pellucida [17].
Such experimental models appear at odds with human studies in which the induction of an acrosome reaction in the in vitro insemination medium actually reduces or prevents sperm from subsequently binding to the zona pellucida [4, 18]. Nevertheless, these data could help account for the ability of agonists, such as follicular fluid and progesterone that sperm encounter within the oviduct prior to zona adhesion, to induce acrosomal exocytosis in the spermatozoa of several species, including the human [9]. The steroidal hormone progesterone reportedly acts by modulating an increase in cytosolic Ca2+ levels, an event that can be recapitulated using non-physiological pharmacological reagents, such as the divalent cation ionophore, A23187. Indeed, by directly facilitating Ca2+ influx, the A23187 ionophore obfuscates the need for the intracellular signaling mechanisms and effector pathways that otherwise regulate the progression of the acrosome reaction. It is therefore not surprising that the processes of acrosomal exocytosis driven by calcium ionophore proceed differently from those occurring in response to physiological stimuli such as solubilized zonae pellucidae. Thus, the spatial profile of an ionophore-induced acrosomal reaction is random, with no obvious preference for initiation in the anterior, middle or posterior acrosomal regions [19]. By contrast, solubilized zonae pellucidae exclusively stimulate a slower and more orderly loss of acrosomal components; beginning at the posterior zone of the acrosome and progressing in an anterograde direction [19]. Whilst these data lend support to the notion of receptor-mediated events controlling membrane fusion and release of acrosomal components, the limited availability of human zonae pellucidae prevents this resource being widely used as physiological stimuli in assisted reproductive settings. Thus, despite its limitations, monitoring of the ability of spermatozoa to undergo an ionophore-induced acrosome reaction has become an important diagnostic criterion of human sperm function testing in clinical ART laboratories.
10.1.2 Histochemical Methods for Detection of Acrosome Reaction
The frequency with which spermatozoa undergo an acrosome reaction represents an important parameter in the evaluation of sperm function as it provides a reliable indication of the likely success of standard IVF and artificial insemination [20]. The impetus to analyze acrosomal exocytosis in human spermatozoa is further emphasized by the fact that a failure to complete this event represents a relatively common etiology associated with the defective spermatozoa of male infertility patients. In a majority of these cases, males present with isolated lesions in sperm penetration of the zona pellucida and either no, or low, rates of fertilization, without any attendant reduction in other semen parameters or levels of sperm–zona pellucida binding [20]. Thus, the diagnostic criterion of DZPIAR is that very low proportions of sperm undergo the acrosome reaction (<16 percent) after binding to the zona pellucida; with estimates suggesting that >25 percent of men seeking recourse to assisted reproductive programs due to idiopathic infertility might be afflicted by this condition [5]. Although these patients commonly have a long duration of unexplained infertility, it is encouraging that they routinely achieve high fertilization and pregnancy rates with ICSI [20]. It is therefore important to diagnose patients with defects in acrosomal responsiveness before commencing ART treatment in order to avoid failure of fertilization with standard IVF. Such diagnoses rely on the application of accurate methodology for monitoring acrosomal status and the establishment of clinical reference ranges for acrosome reaction rates achieved using different stimuli (i.e. ionophore versus progesterone versus zona pellucidae) [21].
As discussed previously, the completion of an acrosome reaction leads to the loss of the acrosomal cap overlying the anterior domain of the sperm head. In species that possess large acrosomes (e.g. the guinea pig and hamster), this phenomenon can be observed directly by either light or phase-contrast microscopy. However, by comparison, the acrosome of human spermatozoa is relatively small and thus very difficult to visualize at the level of resolution afforded by standard light microscopy. As a consequence, alternative protocols have been developed for monitoring the progression of an acrosome reaction and/or evaluating the acrosomal status within a given population of human spermatozoa, with these protocols being broadly divided into four categories and each having its own inherent advantages and disadvantages (Table 10.1).
Table 10.1 Methods Utilized for Detection of the Acrosome Reaction in Human Spermatozoa
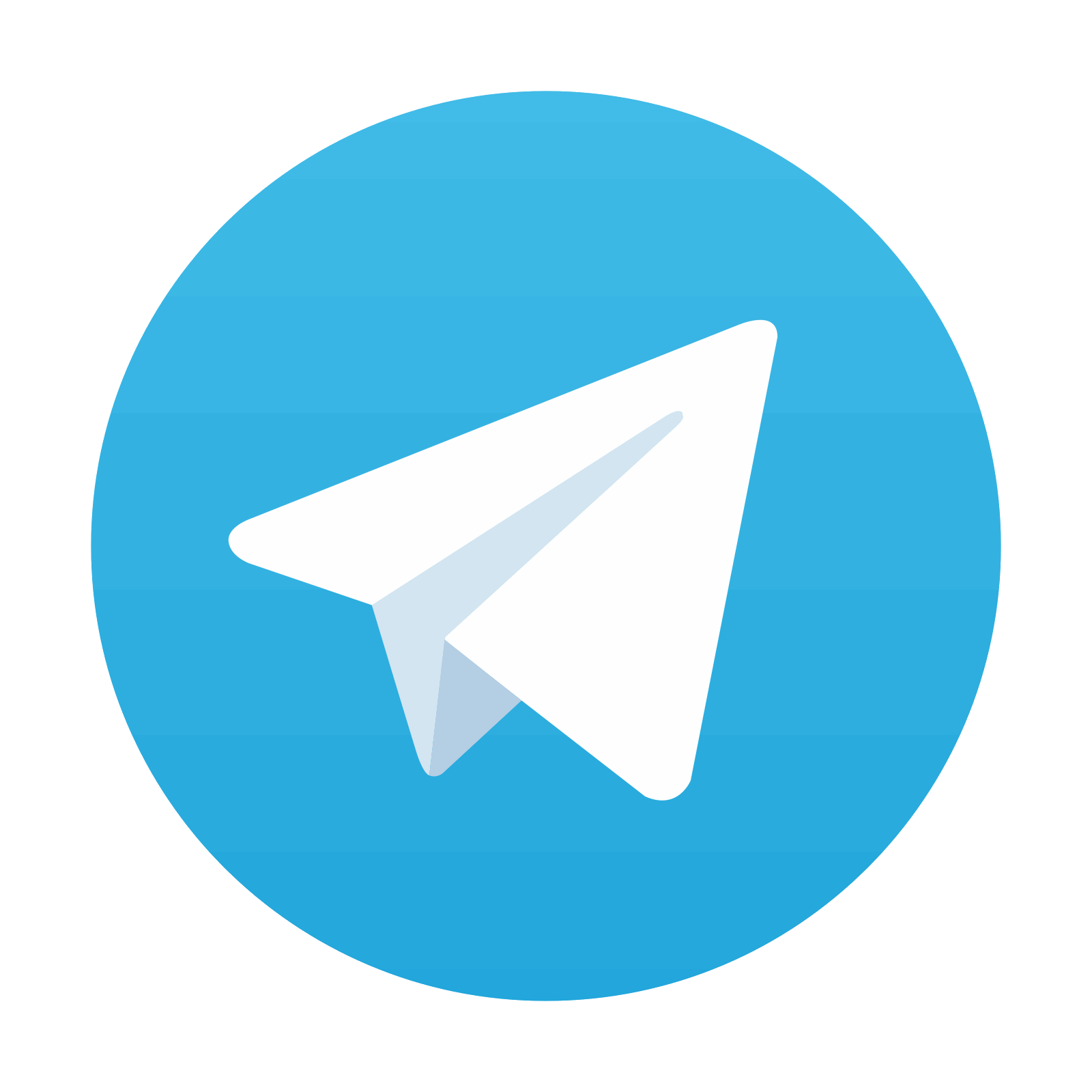
Stay updated, free articles. Join our Telegram channel

Full access? Get Clinical Tree
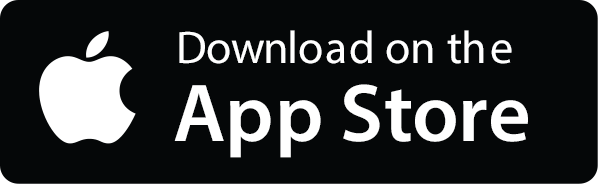
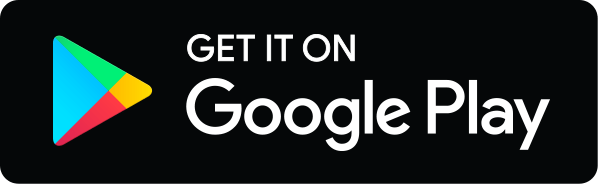
