Amount and source of MSCs
Recipient
Dosage of radiation
Infusion approach
Target
Article
5 × 105 bone marrow-derived mesenchymal stem cells from C57BL/6 mice
C3H/HeN mice
C57BL/6-Tg mice
35 Gy right hind leg
Intravenous injection six weeks post-ionizing radiation
Cutaneo
[47]
1 × 106/50 μl, from C57Bl/6-Tg mice
C3Hf/Kam female and C57Bl/6 male mice
4, 6, 8, 15, 18, 21 Gy WBI/skin-only irradiation
Fibrin microbead implantation less than a 15-min interval
Cutaneo
[52]
50 × 106 MSCs × 3 autologous MSCs
Female minipigs
≥50 Gy local irradiation (at the right lumbar)
Intradermal day 27, 48, 69, 81, 96 after irradiation
Cutaneo
[53]
6 × 106 BMSC from rat
Rat
140 Gy locally to skin
Subcutaneous injection early, day 8 after exposure, during the early desquamation
Cutaneo
[51]
1 × 107, bone marrow-derived mesenchymal stem cells
SD rats
6 Gy whole body
subcutaneously around the wound time not mentioned
Wound
[54]
1.2 × 105 cm2 (area 12.56 cm2 × 2) BMSC from porcine
Minipig
20 Gy local irradiation dorsal regions beside the spine
Construct cultured cutaneous substitutes/human amniotic membrane cover
Wound
[55]
1 × 106/100 μl murine BMSC line
Female C57/Bl6 mice
7 Gy
Intravenous from 16 to 24 h after radiation
Hematopoiesis
[48]
5 × 106 UCB-MSCs from human
Female BALB/c
7 Gy TBI
Intravenous 4 h after irradiation
Hematopoiesis
[35]
6 × 106 Trx-1-overexpressing ucMSC from human
NOD/SCID Mice
4.5 Gy
Intravenous
Hematopoiesis
[56]
1 × 106, adipose-derived mesenchymal stem cells from male human
SD rats
15 Gy whole-abdominal irradiation (from the xiphoid process to the pubic symphysis)
Intraperitoneally injection within 2 h
Intestine
[49]
1 × 106/200 μl umbilical cord-derived mesenchymal stem cells-medium
BALB/C male mice
10 Gy local abdominal irradiation
Intravenous injection immediately
Intestine
[57]
5 × 106 BMSC from human
NOD/SCID mice
3.5 Gy TBI 5.7 Gy abdomen
Intravenous 24 h after irradiation
Small intestinal
[58]
BMSC from rat
SD rats
14 Gy whole abdominal irradiation
Peritoneal cavity conditioned medium intravenous concentrated
Intestine
[59]
5 × 106/100 μl BMSC from human
NOD-SCID mice
3.2 Gy TBI
Intravenous 24 h after TBI
Liver
[46]
5 × 105/15 μl, BMSC from C57/BL6 mice
C57BL/6 mice
15 Gy local irradiation (the head and the neck fields)
Intraglandular 24 h after irradiation
Salivary gland
[44]
1 × 106/100 μl, Ad-HGF-modified MSCs from adult human
Female B6 mice
20 Gy of γ rays locally to the lung
Intravenously 6 h after radiation
Lung
[45]
5 × 106/0.5 ml Ad-MSC from female rat
Rat
15 Gy local irradiation to the right thorax
Intravenous 2 h after irradiation
Lung
[60]
0.1, 1, 5, or 10 × 106, autologous MSCs
Male SD rats
27 Gy local irradiation (the colorectal region)
Intravenous injection immediately after radiation
Colon
[61]
2 × 106 per kilogram autologous MSCs
Male pigs
Between 21 and 29 Gy delivered to the rectum
Ear vein on days 27, 34, and 41 postirradiation
Rectum
[62]
6 × 106 BMSCs from mice
Mdx mice
Lethal radiation TBI
Intravenous
Central nervous system
[63]
Furthermore, MSCs were confirmed to play an important role in tissue regeneration after IR. Experimental data demonstrated that human mesenchymal stem cells (hMSCs) migrated into various tissues including bone marrow, liver, lung et al. following transplanting into adult unconditioned mice. They suggested that the property of hMSC to home to various organs in response to tissue injuries might be a strategy to repair the IR induced damages [64].
4.2 Application of MSCs to Cutaneous Wound and Inflammation
Skin is the largest organ in the body, and dermis has been proved to contain various stem cells populations [65]. Researchers have established the importance of skin dermis as an easily accessible source of stem cell populations and their promising significance in wound repair and other diseases [66, 67]. Cutaneous reactions are major actors in radiation accidents and a limitation for long term radiotherapy, and despit the radiation insensitivity of dermis derived stem cell, wound healing time would prolong following combined radiation injury.
Results of experimental applications of MSCs on the radiation induced wound were given to show that MSCs can significantly improve the rate of cell growth and wound recovery [67]. Lymphocytes accumulated locally at the dermis/subcutis border and vascularization was improved [53]. Moreover, cytokine significantly architected dermal reconstitution, improved angiogenesis, and faster epidermal recovery [68].
Cutaneous fibrosis has long been considered as a significant adverse issue after IR exposure. Previous studies have shown that systemic BMSC administration can inhibit drug induced pulmonary fibrosis [69, 70] and that local application can improve cutaneous wound healing [71, 72]. In addition, systemic infusion of MSCs was also discovered to reduce radiation-induced fibrosis by altering macrophage phenotype and suppressing local inflammation [47]. Furthermore, with the application of autologous MSCs around and in the lesion in clinic case, the healing of the lesion proceeded smoothly without side effect [73].
4.3 Application of MSCs to Haematopoietic System
As we already known, bone marrow is sensitive to radiation damage, and MSCs were wildly used in reconstruction of haematopoiesis and anti-GVHD after HSCs transplantation. Much work has been reported recently in these filed. Experimental data demonstrated that a lower apoptotic ratio, a lower ratio of G0/G1 cell cycle phases and a higher ratio of G2/M and S phases were observed in MSCs treated bone marrow [74]. Moreover, another report documented that the hUCB-MSCs treatment had regenerative effects on monocytes, lymphocytes, and white blood cells, and could significantly downregulate plasma levels of Flt-3L and upregulate transforming growth factor (TGF-β1) [35].
4.4 Application of MSCs to Intestine
The rapid regeneration and short growth period of the gastrointestinal epithelial cells give gastrointestinal tract, especially intestine, the characteristic of high sensitivity to radiation . Radiation-induced gastrointestinal tract (GIT) damage often occurs after radiotherapy for digestive neoplasms, and a considerable amount of research has been done about the stem cell based therapy for radiation-induced intestinal injury (RIII) during the last decade.
Experimental data indicated that the microvascular endothelial apoptosis was the primary radiation damage lesion leading to stem cell dysfunction [75]. Furthermore, MSCs were reported to improve intestinal integrity through regulation of endogenous epithelial cell homeostasis by both increasing proliferation and inhibiting apoptosis of intestinal epithelial cells [58, 76]. After MSCs administration, the moderated (RIII)-healing effects, including neovascularisation, anti-inflammation, and maintenance of epithelium homeostasis were observed [49]. And the efficient anti-inflammatory effects of MSCs based therapy were again confirmed in clinical case [77]. The injection of MSCs could also significantly accelerated recovery of the gut by stimulating proliferation of the crypt cell pool [78]. With different animal models, many studies proved similar positive effect of MSC to RIII [76, 79, 80]. The components of MSCs conditioned medium could also increase the survival rate, and improved the small intestinal structural integrity of irradiated mice, which supported the paracrine effect of MSCs [57].
4.5 Application of MSCs to Other Organs
To evaluate the potential therapeutic effect of MSCs, a total body radiation exposure was performed on NOD/SCID mice. The results indicated that the plasmatic transaminases (AST and ALT) levels, oxidative stress and apoptosis process in the liver vascular system were all significantly decreased, and cell proliferation in the liver increased that might improve liver dysfunction [46]. In fact, MSCs can exert their therapeutic functions without a grafting on damaged region. There were reports that the infused MSCs were not found in liver, and they corrected liver dysfunction in an indirect manner [81].
Besides, the bone marrow-derived clonal MSCs could ameliorate salivary damage following irradiation by decreasing the apoptotic cells and increasing microvessel density in salivary glands [44, 82]. Earlier studies found that MSCs resident in lung mostly at early stages. A different retention peak with the model of engrafting MSCs to SD rats of 15 Gy local irradiation were reported, which was probably due to the number of engrafted cells or the dose of radiation, and affect chemokine release [60]. Researchers found that MSC-based therapy could effectively reduce secretion and expression of pro-inflammatory cytokines, decrease expression levels of profibrosis factors, and protect lung epithelial cells, which might also inhibit lung fibrosis [45].
4.6 Application of MSCs to Radiation Combined Injury
Combined injuries are defined as the combination of radiation exposure and tissue injuries from blast and thermal energy, which is much more complicated and difficult to heal than single injuries. Adult stem cell-based therapy has aroused great hopes for the treatments of diseases and injuries, including combined radiation and wound injury.
Experimental data demonstrated that BMSCs over-expressing hPDGF-A and hBD2 could significantly accelerate wound healing process of combined radiation wound injury [83]. Furthermore, the therapeutic effects of MSCs were reported to exist in various aspects of wound healing, including collagen deposition, re-epithelialisation, and granulation formation [55]. Very recently, the granulation tissue derived cells (GTCs) were further characterized as an abundant cell source for their important therapeutic efficacy in wound healing and tissue repair [84]. For skin is relatively insensitive to radiation, in our previous study, we demonstrated that the GTCs from combined radiation and wound injury may represent an alternative source of autologous adult stem cells for transplantation (not published).
5 Therapeutic Implications of Dermal Multipotent Cells for Wound Repair
It has been increasingly established that stem cells play an important role in wound healing. Several studies in recent years also suggest that adult stem cell-based therapy has aroused great hopes for regenerative medicine and the treatments of diseases and injuries [85]. Ideal stem cell sources are thought to be easily accessible, capable of rapid expansion in culture, immunologically compatible, and amenable to stable differentiation or transdifferentiation. Skin, the largest organ of the body, is considered as a natural resource of adult stem cells [65]. However, it is surprising that the dermis, which represents a larger adult stem cell reservoir than the hair follicle and epidermis together, has largely escaped the focus of the majority of the stem cell community.
Until in 2001, our and other groups first described the existence of dermal multipotent cell populations that were unidentified previously in the skin of adult mammals, such as mice, rat, and human, and these cells were considered similar to bone marrow mesenchymal stem cells [86]. By transplanting dermal cells from green fluorescent protein (GFP) transgenic mice intravenously, we provided evidence that these dermal cell populations could differentiated into different cells in many organs and tissues, such as bone marrow, lung, liver and kidney. Moreover, we established a unique clonal population of dermal multipotent cells (DMCs) from neonatal rat skin [87]. Based on the adhesion to tissue-culture plastic and multilineage differentiation potential in vitro, DMCs were easily isolated and incubated on standard culture condition. The surface antigenic profile of DMCs has been shown to be positive for CD44 and CD90 expression, but negative for CD34, similar to marrow mesenchymal stem cells . However, the former showed a much higher proliferation potential. The number of stem cells in the adult tissue is very low, and seeking method to expand the supply of undifferentiated cells remains a challenging issue. We have established that beta-microglobulin, a MHC class I subunit, could act as a novel growth factor to simulate the ex vivo expansion of undifferentiated multipotent stem cells (such as dermal multipotent cells) to reduce the use of fetal bovine serum, which can elicit possible contamination or immunological reaction for clinical application [88].
To investigate the therapeutic effects of the multipotent cell populations within dermal tissues for the repair of wounded tissues, we applied exogenous DMCs to skin wounds and both topical and systemic transplantation of DMCs accelerated the healing of simple wounds in rats. We also noted that topical transplantation exhibited an earlier healing effect than systemic transplantation [89]. The engrafted DMCs were observed to differentiate into multiple cell types that would promote tissue repair, which indicated that they may be a potential source of wound-healing fibroblasts [87]. DMCs also produce many growth factors—such as hepatocyte growth factor (HGF) to improve wound healing [89]. HGF not only induces mitogenic and antiapoptotic activity, but also has an immunomodulatory action to create an appropriate inflammatory response for DMCs at the wound. Furthermore, we also observed that DMCs have the capacity to induce the formation of hair follicle-like structure at the subcutaneous sites in nude mice when implanted along with follicle epithelial cells, suggesting that these multipotent dermal cells are also involved in skin regeneration [90].
In addition to skin wound healing, DMCs are also capable of promoting the hematopoietic recovery in sublethally irradiated rats. These cells can represent an alternative source of adult stem cells for transplantation, restore microenvironment and promote hematopoietic recovery [91]. The implanted DMCs were detected to recruit preferentially to wounded tissues, which was mediated by an elevated expression of stromal-derived factor (SDF-1) after an injury. SDF-1 is a ligand for the CXC chemokine receptor 4 (CXCR-4) on DMCs and SDF-1/CXCR4 signaling is critical for the recruitment of stem cells to the site of injury. DMCs were further applied in rats that received combined radiation and wound injury through tail vein. And we have reported for the first time that systemic transplantation of neonatal dermal multipotent cells significantly promoted survival and accelerated both hematopoietic recovery and wound healing in rats with combined radiation and wound injury, suggesting that stem cell therapy can achieve multiple therapeutic effects and provides a potential new strategy for the treatment of severe traumatic injuries with multiple tissue/organ damage, such as radiation combined injuries [92].
Given that DMCs play an important role in wound repair and are now emerging as potential therapeutic agents [90], we propose that the dermis may represent one of the best autologous sources of stem/progenitor cells for therapeutic applications in skin replacement and repair of other organs. However, the lack of specific markers for histological localization of stem/progenitor cells within the dermis still remains a problem. Moreover, although stem cell based biotherapy has aroused great hopes for the treatments of diseases and injuries, we and others highlighted that there is a risk that cell therapies will produce malignancies [93]. A spontaneous transformation were observed in the dermal multipotent cells after long-term in vitro culture and that Ras/Raf/MEK signaling pathway, protein phosphatase 2A (PP2A), and transcriptional coactivator PC4 may play a role in the malignant transformation of these cells [93, 94]. Furthermore, we have identified a class of lipophilic heptamethine cyanine cells via an energy-dependent pathway. These dyes have superior optical, biocompatible, and pharmacokinetic properties for tumor targeting and for imaging with a superb contrast index value that make them attractive for detecting tumorigenic cells [95]. Considering this safety issue, the long-term risk for tumor occurrences that results from the use of adult multipotent stem/progenitor cell in different pathological conditions must be further investigated. Moreover, there is an urgent need to develop more sensitive methods to detect tumorigenic cells at early stages.
It has long been a goal in biomedical science to harness endogenous repair mechanisms to promote tissue repair and regeneration. However the recent focus is mainly upon stem cell-based transplantation to treatment of various diseases. It would be another interesting approach to recruit resident dermal stem cells for further chronic wound treatment and skin regeneration. As the evolving body of work shows that stem cell populations resided in many adult tissues, another therapeutic approach would be promising. Recently, the skin granulation tissue has been proposed as a promising source of dermal stem cells, and the newly formed granulation tissue is enriched in cells that expressed stem cell surface markers after wounding [96]. However, the origin and biological characteristics of these cells have not been well investigated. Our team explored that, in normal conditions, dermal stem/progenitor cells keep quiescent, and could be activated to proliferate upon injury and enriched in granulation tissues (Fig. 1). The activated dermal stem/progenitor cells [here we termed granulation tissue derived cells (GTCs)] exhibited with enhanced proliferation, self-renewing, and multi-differentiation abilities. And topical transplantation of GTCs into the combined radiation and wound injury mice accelerated wound healing and reduced tissue fibrosis. We also proved that miR-21 mediated ROS generation negatively regulates the stemness-related properties of granulation tissue derived cells [97]. Considering the complex factors involving in the wound microenvironment, we further study the response of dermal cells to a single mechanical injury. The wound scratch model in vitro is administrated to mimic the wound trauma. Following the mechanical injury, dermal cells could be completely activated to proliferation at about 36 h, which is consistent with the in vivo data. Furthermore, dermal cells were changed into a stemness phenotype with increase expression stem cells marker Sox2 and vimentin by mechanical injury, which also supplies evidence for in vivo data that GTCs showed enhanced stemness related properties (Fig. 2).



Fig. 1
Activation of dermal stem cell proliferation after wounding. a BrdU in normal skin or wound sites was measured by immunohistochemistry. C, control group, BrdU was injected consecutively three days in normal mice; L, long term BrdU labeling, BrdU was injected consecutively three days after wounding; S, short term BrdU labeling, BrdU was injected a single time at the 60 h after wounding; d, days after wounding. Scale bar = 100 µm. b BrdU in cultured clones of newly isolated in vivo labeled GTCs was measured by immunofluorescence. Scale bar = 500 µm

Fig. 2
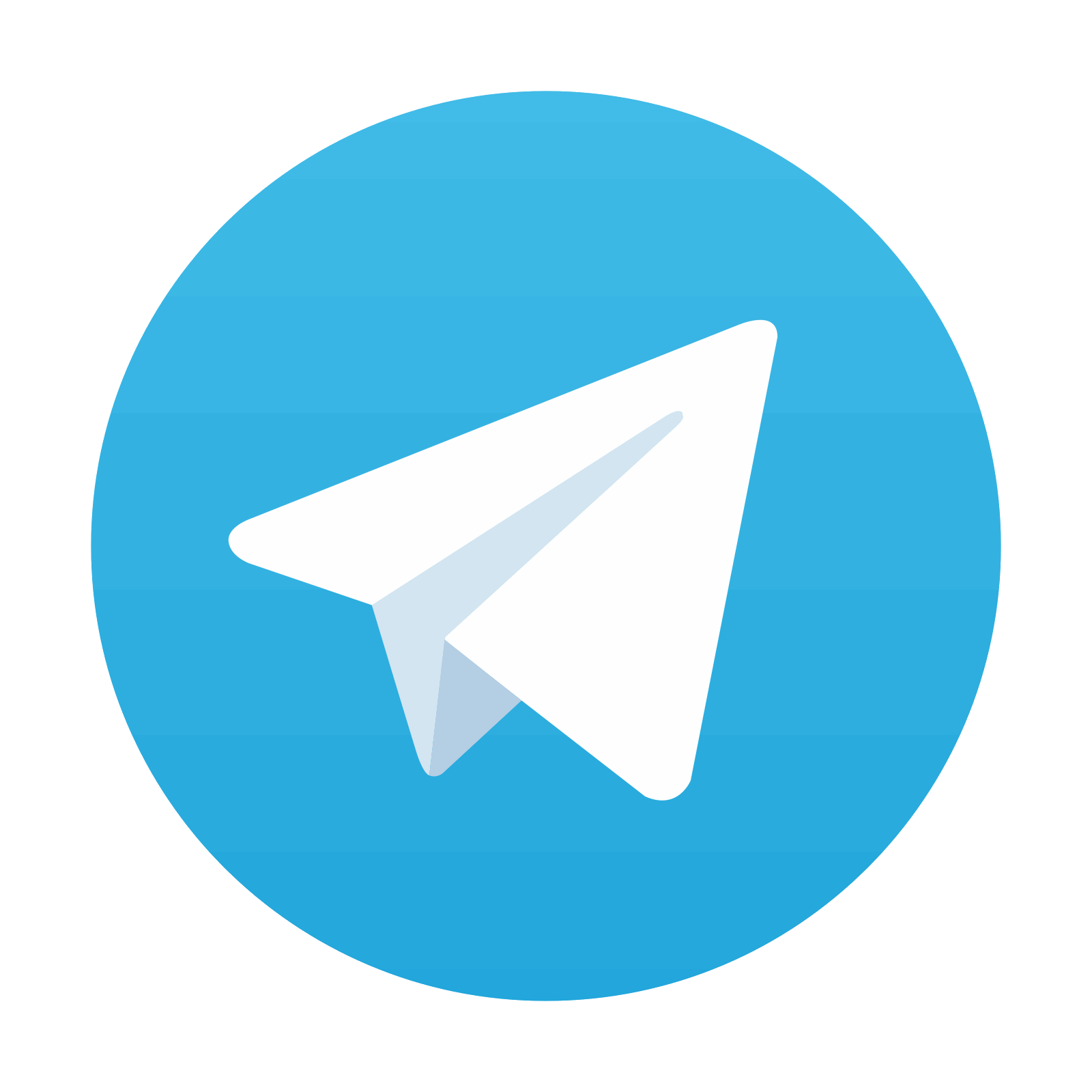
Characterization of changes in dermal cells following mechanical injury. a Representative pictures of dermal cells in wound margins following mechanical scratch. Scales are 500 µm. b Immunofluorescence staining for Ki67 demonstrating activation of dermal cells following mechanical scratch. Cell nuclei are counterstained with DAPI. Scales bars are 500 µm. c Immunofluorescence staining for Sox2 and Vimentin in dermal cells of scratch edge 72 h following mechanical scratch. Cell nuclei are counterstained with DAPI. Scales bars are 200 µm. d Western blot analysis of Sox2 and Vimentin expression at indicated time points in dermal cells following mechanical scratch. e Real time PCR analysis of Sox2 expression in dermal cells 72 h following mechanical scratch. f Colony formation assay of dermal cells 72 h following mechanical scratch. g Quantification of colonies in f. **, p < 0.01. p values were calculated using the independent-samples t test in e and g
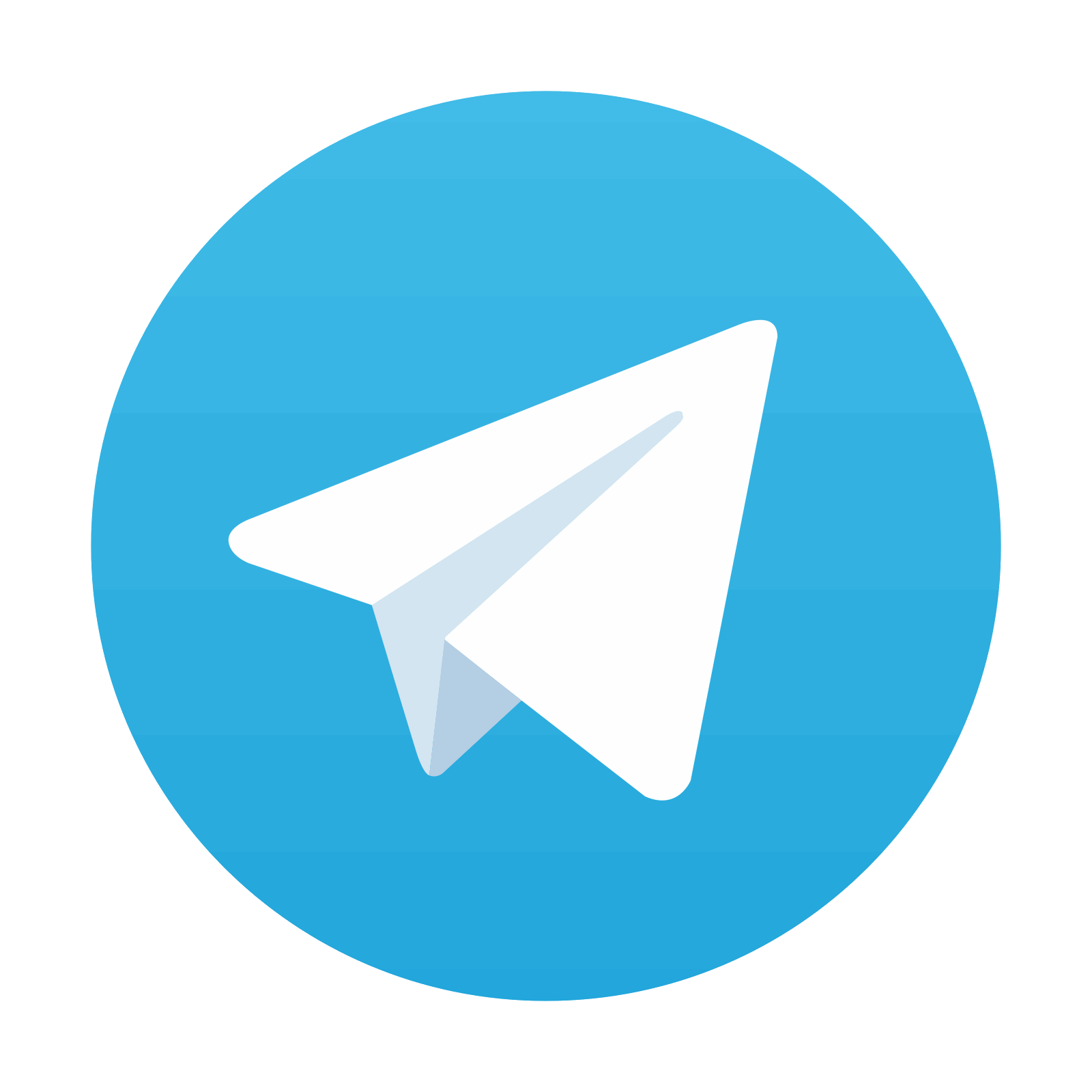
Stay updated, free articles. Join our Telegram channel

Full access? Get Clinical Tree
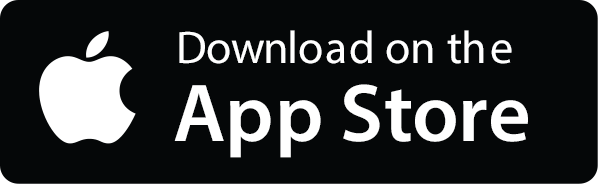
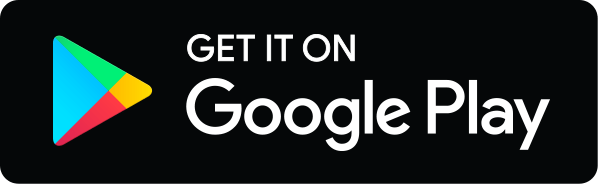
