Chapter 13 Plant cell and tissue culture; biochemical conversions; clonal propagation
INDUSTRIAL SIGNIFICANCE
Shikonin, a dye and antibacterial, is commercially produced by the cultivation of Lithospermum plant cells and the production of the ginsenosides and antitumour alkaloids of Catharanthus roseus are currently being developed; Japanese patents exist concerning the manufacture of many secondary metabolites including the purple pigment from Melissa officinalis, the production of catharanthine and ajmalicine by cell cultures derived from C. roseus anthers, the manufacture of an analogue of taxol (q.v.) by callus cultures of Taxus spp., and tropane alkaloids from Duboisia tissue cultures. A vast amount of work has been reported during the last decade and the majority of common medicinal plants, and many less common ones, have been subjected to cell culture investigation. Nevertheless, in the majority of cases, yields of metabolites have been commercially disappointing. Staba, a pioneer in the investigation of medicinal plant cell culture, stated (J. Nat. Prod., 1985, 48, 203) ‘there are arguably as many gravestones as milestones along the way in developing plant tissue culture systems for the production of secondary metabolites’. Ultimately, of course, as Fowler pointed out over 25 years ago (Chem. Ind., 1981, 229), ‘no matter how elegant the science, the fundamental criterion has to be price comparability coupled with profitability’, a fact clearly obviated by subsequent events.
CULTIVATION OF PLANT CELLS
Cultures of single cells growing under controlled conditions in a liquid medium, or callus cultures consisting of undifferentiated masses of cells developing on a semi-solid medium, can be initiated from parenchymatous tissues of shoots, roots and other plant structures (see Fig. 13.1). The maintenance of such cultures depends on an adequate supply of nutrients, including growth factors, and a controlled sterile environment. The cells, although undifferentiated, contain all the genetic information present in the normal plant. By suitable manipulation of the hormone content of the medium, it is possible to initiate the development of roots, shoots, and complete plants from the callus cell culture and to encourage the division of cells in a suspension culture.
Several forms of suspension culture are commonly utilized, as follows.
To maximize on the cell mass produced, the cell suspension culture eventually becomes very dense and this presents problems of even aeration.
PRODUCTION OF SECONDARY METABOLITES
Low production of desired metabolites
Variations in the relative hormonal contents of the growth medium can also affect metabolism. It has been reported that reduced concentrations of 2,4-D increased alkaloid formation in C. roseus cultures and that abscisic acid and antigibberellin compounds have similar effects. With Thalictrum minus, ethylene has been shown to activate the production of berberine in cell cultures from the key intermediate (S)-reticuline and the ethylene-producing reagent 2-chloroethylphosphoric acid stimulates anthraquinone production in callus cultures of Rheum palmatum. Conversely, cardenolide accumulation in Digitalis lanata tissue cultures is decreased by ethylene. Cytokinins have been found to enhance secondary metabolite accumulation in a number of tissue culture studies—indole alkaloids (C. roseus), condensed tannin (Onobrychis sp.), coumarins (Nicotiana sp.), rhodozanthin (Ricinus sp.), berberine (Thalictrum minus). Rhodes et al. found a five-fold increase in alkaloid content of a culture of Cinchona ledgeriana occurs when cells are transferred from a 2,4-D, benzyladenine medium to one containing IAA and zeatin riboside. For information on plant hormones, see Chapter 12.
Although alkaloids from a wide range of medicinal plants have been produced satisfactorily by cell culture in the laboratory, a singular lack of success has been experienced in obtaining quinine and quinidine from Cinchona cultures and morphine and codeine from those of Papaver somniferum although, in both cases, other alkaloids are formed. To some extent the problems with the former are being overcome by the use of transformed roots (see below) but the growth rate is very slow. With morphine biosynthesis it appears that lack of developed laticiferous tissue in the unorganized cell culture may be responsible because cytodifferentiation leading to latificer-type cells leads to morphinan alkaloid production. One problem with Catharanthus cell cultures has been their inability to dimerize the requisite indole monomers to form the medicinally important anticancer alkaloids vinblastine and vincristine. In a similar way the accumulation of monoterpenes in cell cultures of some volatile oil-producing plants is severely limited, probably because of the absence of such storage structures as glands, ducts and trichomes. Thus, in Rosa damascena callus and suspension cultures, negligible amounts of monoterpenes are accumulated, although enzymes with high activity for the conversion of mevalonate and IPP into geraniol and nerol (see Chapter 18) are extractable from the apparently inactive callus. In this case, non-compartmentalization of the metabolites probably leads to their further metabolism. This is supported by the finding that, when added to cultures of Lavandula angustifolia, the monoterpenoid aldehydes geranial, neral and citronellal are reduced to their corresponding alcohols, geraniol, nerol and cirtronellol which, once formed, disappear from the cultures over about 15 h.
Improved metabolite production may sometimes be achieved by the addition of precursors to the culture medium. Thus, addition of coniferin (a phenylpropane) to cell suspension cultures of Podophyllum hexandrum improved podophyllotoxin production 12.8-fold and an increase in quinoline alkaloids was obtained with Cinchona ledgeriana cultures fed with L-tryptophan. With transformed root cultures of Catharanthus roseus, the addition of the precursor loganin to the culture medium has been shown to increase the production of both ajmalicine and serpentine at the early stationary phase of growth, although it produced no increases during the early and late exponential growth phases. Catharanthine production was unaffected but was increased, together with the other alkaloids, by multiple feedings of loganin (see E. N. Gaviraj and C. Veeresham, Pharm. Biol., 2006, 44, 371 and references cited therein).
< div class='tao-gold-member'>
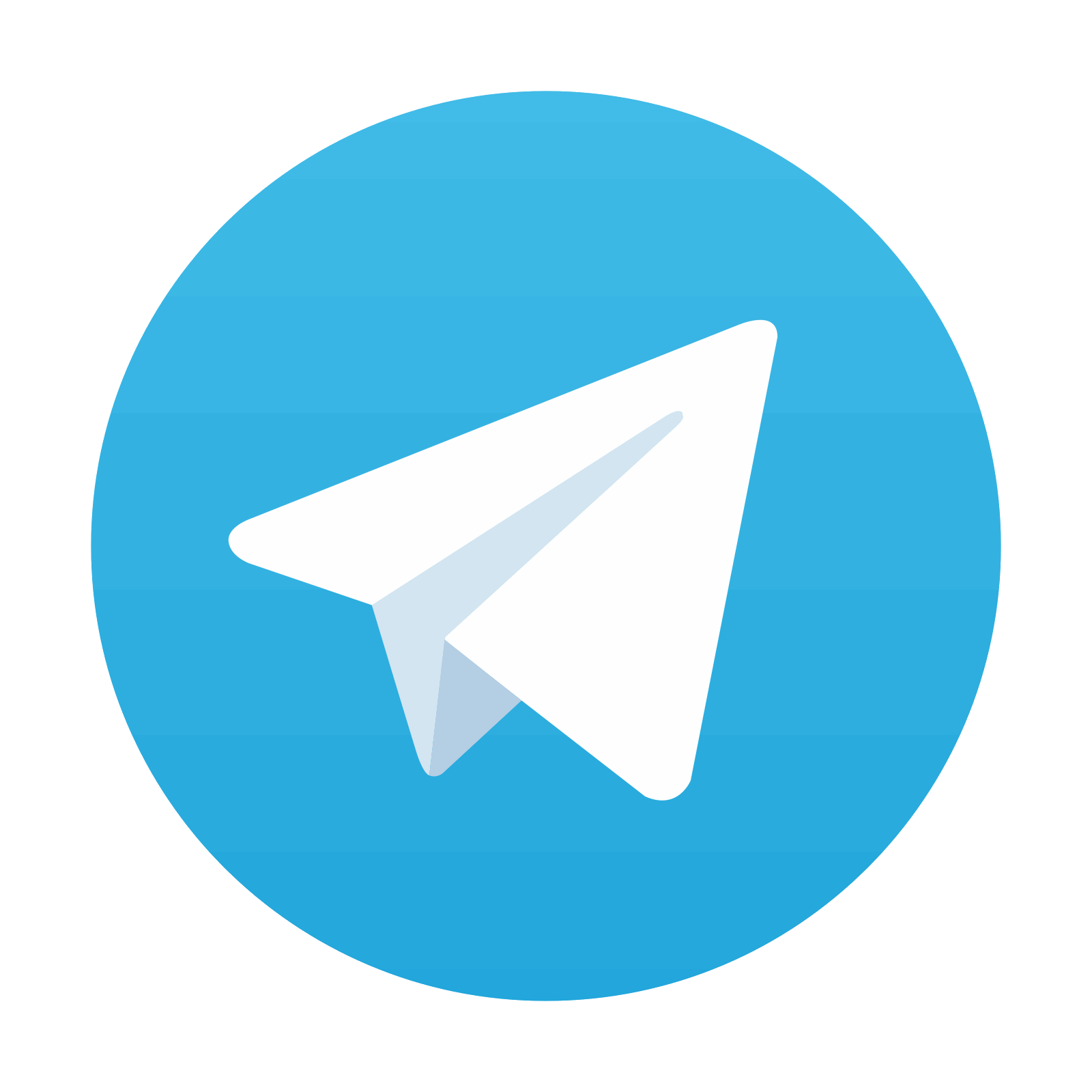
Stay updated, free articles. Join our Telegram channel

Full access? Get Clinical Tree
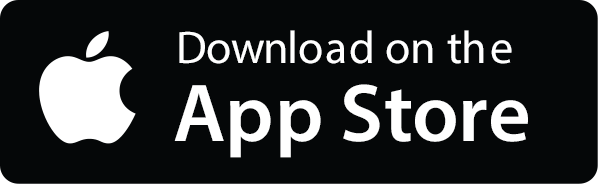
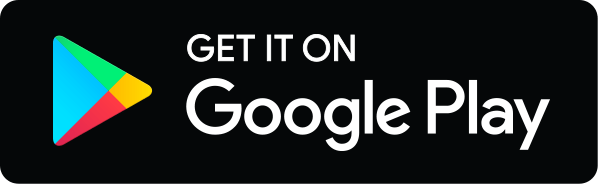