The diagnosis of myocardial infarction
The diagnosis of myocardial infarction has for the past two decades been based on WHO criteria which comprise a typical history of chest pain, the presence of diagnostic ECG abnormalities and a rise in biochemical markers. The presence of two or more of these three has defined the diagnosis. In recent years, this long-established definition has been overtaken by the advent of the troponins, which are more sensitive biochemical markers. Many studies have shown that troponins are released in some patients without conventional ECG changes of infarction, in particular elevation of the ST segment and T wave inversion. These patients are found to be at increased risk of subsequent cardiac events. This has given rise to the concept of ‘acute coronary syndromes’, which comprise unstable angina with or without a rise in troponin, non-ST segment elevation myocardial infarction and ST segment elevation myocardial infarction. The use of troponin measurement thus potentially reveals biochemical changes that would not previously have been detected in patients with chest pain. This has major implications in the definition of myocardial infarction, and of course for the patients who may now be given this diagnosis, but who would formerly have been reassured that they had not had an infarct.
A task force drawn from a number of expert bodies has published a consensus ‘Universal Definition of Myocardial Infarction’ (see Further reading, p. 193). This places biomarkers central to the definition, requiring the detection of a rise and/or fall of a biomarker (preferably troponin). At least one troponin value should be above the 99th centile of a ‘normal’ population, and the assay should have an analytical performance at this level defined by a coefficient of variation of less than 10%. This is a challenging analytical standard that not all currently available assays meet. In addition there should be at least one of:
- symptoms of ischaemia
- ECG changes indicative of new ischaemia
- development of pathological Q waves in the ECG
- imaging evidence of new loss of viable myocardium or new regional wall motion abnormality.
This new definition is not yet universally adopted. There are implications for the laboratory in potential increases in testing and the analytical requirements, for cardiology services in the increase in patients receiving a diagnosis of myocardial infarction, and of course for patients (and their families, employers and insurers) receiving this diagnosis who would not previously have done so.
Biochemical tests in myocardial infarction and ischaemia
After myocardial infarction, a number of intracellular proteins are released from the damaged cells. The proteins of major diagnostic interest include:
- Troponin I and troponin T
- Enzymes, such as creatine kinase (CK), CK-MB, aspartate aminotransferase (AST) and lactate dehydrogenase (LDH)
- Myoglobin
- Ischaemia-modified albumin.
The troponins and CK will be considered in detail below, since they are the most widely established biochemical indices of myocardial damage, with the troponins largely taking the place of the enzymes in recent years. CK is, however, still widely used in some countries, and retains a use in the investigation of muscle disorders. Myoglobin is also a sensitive index of myocardial damage, and it rises very rapidly after the event. However, it is non-specific, since it is raised following any form of muscle damage. It is not in wide laboratory use, but has a role in point of care analysers in the emergency setting. A negative result on an appropriately timed sample can be used to rule out myocardial damage and thereby determine early patient management. A positive result requires further investigation. Ischaemia-modified albumin is a new early and sensitive biochemical marker of myocardial ischaemia, but is non-specific and not widely available. Its role in patient management is yet to be determined.
Time-course of changes
After a myocardial infarction, the time-course of plasma biochemical markers always follows the same general pattern (Figure 12.1). After an initial ‘lag’ phase of at least 3 h, during which levels remain normal, they rise rapidly to a peak between 18 and 36 h, and then return to normal at rates that depend on the half-life of each marker in plasma. The biphasic response of troponins with a rapid rise and prolonged elevation, and the rapid rise and fall of CK and CK-MB activity should be particularly noted. In patients treated with thrombolytic agents, the general pattern of plasma marker changes shown in Figure 12.1 is slightly modified. Following successful thrombolytic therapy (e.g. with streptokinase), there is a ‘washout’ of markers from the infarcted area, and levels rise rapidly to reach an early peak, at 10–18 h.
Optimal times for blood sampling
Figure 12.1 Patterns of biochemical markers in the first few days after an uncomplicated myocardial infarction.
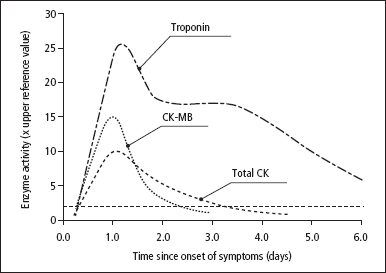
Table 12.1 Time-course of plasma biochemical marker elevation after myocardial infarction
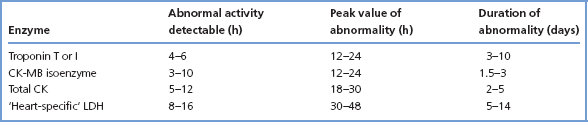
Optimal times for blood sampling
A sample taken on admission, if elevated, will make the required diagnosis, but if not elevated will not rule out the diagnosis if insufficient time has elapsed for a significant CK or troponin rise to have occurred. A sample taken between 6 and 12h after the onset of symptoms will give more reliable results (Table 12.1).
Except for the occasional patient seen for the first time 2 days or more after the episode, in whom troponin measurements might still be useful, it is very rarely of any value to take samples for plasma markers after 48h from the onset of symptoms that suggest a diagnosis of myocardial infarction.
Troponin
The troponin complex is exclusively present in striated muscle fibres and regulates the calcium-mediated interactions of actin and myosin. It comprises equimolar quantities of the structurally unrelated proteins troponin T, troponin I and troponin C. Troponin T binds tropomyosin; troponin I is an inhibitory protein and troponin C is responsible for binding calcium. Three distinct isoforms of troponin T and I exist, one each in slow twitch and fast twitch fibres of skeletal muscle, and one in cardiac muscle. These cardiac-specific forms of troponin can be recognised and measured in the plasma. There are two isoforms of troponin C, one found in slow twitch and cardiac muscle, and one in fast twitch muscle, so there is not a troponin C species unique to myocardium.
In human heart the cardiac-specific troponin T and troponin I are largely insoluble, but 3–5% exists as a soluble cytoplasmic pool. Following cardiac myocyte necrosis, this soluble fraction probably accounts for the early rapid release of troponin into the circulation, and the slower release of the insoluble fraction accounts for the prolonged plateau of troponin release. The existence of the cardiac-specific isoforms of these troponins makes them the most specific of all the biochemical markers for cardiac damage. Under normal circumstances there is no cardiac troponin T or I detectable in the circulation by currently available assays, so any detectable rise is of significance, contributing to the high sensitivity of these tests. However, troponin may also sometimes be elevated in renal failure, severe heart failure and acute pulmonary embolism.
In general, although the initial rise in cardiac troponins after myocardial infarction occurs at about the same time as CK and CK-MB, this rise continues for longer than for most of the enzymes because of continuing release of ‘insoluble’ protein from the infarcted muscle. Cardiac troponin measurements are particularly useful in excluding the diagnosis of myocardial damage, particularly after 12h following chest pain or other symptoms, and in patients who are likely to have concurrent cardiac and skeletal muscle damage.
Creatine kinase
There are three principal CK isoenzymes, each comprising two polypeptide chains, either B or M; these give the dimers BB, MB and MM.
- Skeletal muscle has a very high total CK content; over 98% normally comprises CK-MM and less than 2% CK-MB. CK-MB may rise to 5–15% in some patients with muscle disease, and also in athletes in training.
- Cardiac muscle also has a high CK content. It comprises 70–80% CK-MM and 20–30% CK-MB. As a general rule, cardiac muscle is the only tissue with more than 5% CK-MB. Before troponin analyses were widely available, CK-MB measurement was useful in confiming the cardiac origin of a raised CK.
- Other organs, such as brain, contain less CK, often CK-BB. However, CK-BB rarely appears in plasma and is not of diagnostic importance. Plasma normally contains more than 95% of its CK as CK-MM.
CK is used in the diagnosis of myocardial infarction and some muscle diseases (see below). Increases, sometimes large, may occur after trauma or surgical operations, IM injections, in comatose patients, in diabetic ketoacidosis, acute renal failure and hypothyroidism, and after prolonged muscular exercise, especially in unfit individuals.
Creatine kinase and muscle disease
Plasma CK, AST, LDH and ALT activities may be increased in muscle disease. However, plasma total CK is usually the measurement of choice, irrespective of the aetiology of the disorder, since it is increased in the greatest number of cases and shows the largest changes.
- Muscular dystrophy In Duchenne-type dystrophy, high plasma CK activity is present from birth, before the onset of clinical signs. During the early clinical stages of the disease, very high activities are usually present, but these tend to fall as the terminal stages of the disease are reached. Smaller CK increases are present in other forms of muscular dystrophy. About 75% of female carriers of the Duchenne dystrophy gene have small increases in plasma CK activity.
- Malignant hyperpyrexia This is a rare but serious disorder, characterised by raised body temperature, convulsions and shock following general anaesthesia. Many of the patients show evidence of myopathy. Extremely high plasma CK activities are seen in the acute, post-anaesthetic stage, but smaller increases often persist and can also be detected in the relatives of affected patients. Preoperative screening of plasma CK is not a reliable way of detecting patients liable to develop malignant hyperpyrexia, and should be limited to those patients with a family history of anaesthetic deaths or of malignant hyperpyrexia.
- Miscellaneous muscle diseases CK is variably increased in various myopathies, including that due to a side effect of treatment with β-hydroxy-β-methylglutaryl-coenzyme A (HMG-CoA) reductase inhibitor (‘statin’) cholesterol-lowering drugs. It is also raised in polymyositis.
- Neurogenic muscle disease Plasma CK activity is usually normal in peripheral neuritis, poliomyelitis and motor neuron disease.
CK-MB isoenzyme
CK-MB is a more sensitive and specific test for myocardial damage than total CK. Its use has been largely overtaken by the widespread availability of troponin measurement. If troponin measurement is not readily available, plasma CK-MB measurements (preferably by a mass measurement method) are the best alternative. Measurement of CK alone is no longer recommended because of its lack of specifity.
CK-MB is available on some point of care analysers.
The diagnosis of heart failure
Heart failure is a complex clinical condition in which the heart’s ability to pump is compromised by one or more of a number of underlying conditions, commonly ischaemic heart disease, but also heart valve abnormalities. The prognosis is poor if untreated, with a 2-year survival rate of under 50%.
The diagnosis of heart failure can be difficult, especially since the usual presenting symptoms such as breathlessness or ankle swelling are common and can be due to many different conditions. Physical examination is neither sensitive nor specific for heart failure, even in expert hands, with incorrect diagnoses in up to 50% of patients. The definitive diagnosis is best made by echocardiography, but access to this may be limited or delayed. BNP is a neurohormone secreted by cardiac myocytes in response to volume expansion and pressure overload, and plays a role in circulatory homeostasis. In heart failure the level of BNP increases, enabling differentiation of cardiac and pulmonary causes of breathlessness. It has an evolving role in the diagnosis of heart failure in both primary care and the emergency setting, since it costs considerably less than echocardiography, and the result can be available much more rapidly.
Laboratory and point of care assays for BNP and for the inactive peptide N-terminal-proBNP (NT-proBNP) are available, and provide qualitatively similar information. Their accuracy is greatest in patients with more severe disease and poorest in those already receiving treatment. Levels rise with age, so age-related cut-offs should be used.
A number of other conditions can cause elevated BNP levels but, in a patient who is not on heart failure treatment, if levels are below the cut-off level then heart failure is highly unlikely, and the patient should be investigated for other conditions. If the level is elevated the patient should proceed to further assessment, including echocardiography. The introduction of this strategy has the potential to speed up accurate diagnosis of heart failure, and to save money by restricting the use of echocardiography to those patients most likely to benefit from its use.
The diagnosis of thromboembolic disease
Laboratory investigations have a part to play in the investigation of possible thromboembolic disease. As the fibrinolytic system breaks down clots formed of cross-linked fibrin, degradation products are produced, including D-dimers. Under normal circumstances these are not detectable in the circulation, but are present in thromboembolic disease and in disseminated intravascular coagulation. Measurement of D-dimer levels is used in the diagnosis (or, more accurately, exclusion) of thromboembolic disease such as deep venous thrombosis (DVT) and pulmonary embolism (PE). In carefully selected patients at relatively low risk of DVT or PE, a normal result can effectively rule out these conditions, avoiding the need for more expensive and time-consuming imaging techniques. Careful clinical assessment is needed, since false-positive results are possible in non-thrombotic pathologies such as neoplasia, recent surgery, myocardial infarction and trauma.
Both laboratory and point of care tests are available for D-dimers and can help in the immediate management of patients presenting with symptoms or signs suggestive of DVT or PE, since a negative result offers reassurance. A positive result will require further investigation.
Cardiovascular risk factors
Many factors are associated with or cause increased cardiovascular risk. These can pragmatically be divided into those which cannot be influenced and those which can be influenced and reduction of which has been demonstrated to reduce risk. Those that cannot be influenced include a family history of premature vascular disease, age and pre-existing vascular disease. Those whose modification has an established role include cigarette smoking and hypertension (not considered further here), diabetes (see Chapter 6) and the hyperlipidaemias, especially hypercholesterolaemia. Lipid metabolism and the hyperlipidaemias are covered in some detail below, followed by a description of how cardiovascular risk is assessed and treated. Other novel biochemical markers of vascular risk are briefly described.
Lipids
Lipids act as energy stores (triglycerides) and as important structural components of cells (cholesterol and phospholipids). They also have specialised functions (e.g. as adrenal and sex hormones). The main lipids, being insoluble in water, are transported in plasma as particulate complexes with proteins, the lipoproteins.
From the clinical viewpoint, it is the strong relationship between plasma lipid levels and the incidence of ischaemic vascular disease, particularly of the coronary arteries, that is of major importance. In the following section, we outline:
- The biochemistry of the main body lipids.
- The mechanisms for lipid transport in plasma.
- The importance of lipids, and other factors, in the pathogenesis of arterial disease.
- The role of plasma lipid measurements in the management of hyperlipidaemia and in cardiovascular risk reduction.
Cholesterol
This is a steroid that is present in the diet, but it is mainly synthesised in the liver and small intestine, the rate-limiting step being catalysed by HMG-CoA reductase. Cholesterol is a major component of cell membranes, and acts as the substrate for steroid hormone formation in the adrenals and the gonads. It is present in plasma mainly esterified with fatty acids. The body cannot break down the sterol nucleus, so cholesterol is either excreted unchanged in bile or converted to bile acids and then excreted. Cholesterol and bile acids both undergo an enterohepatic circulation.
Triglycerides
These are fatty acid esters of glycerol, and are the main lipids in the diet. They are broken down in the small intestine to a mixture of monoglycerides, fatty acids and glycerol. These products are absorbed, and triglycerides are resynthesised from them in the mucosal cell. Most of these exogenous triglycerides pass into plasma as chylomicrons (see below).
Endogenous triglyceride synthesis occurs in the liver from fatty acids and glycerol. The triglycerides synthesised in this way are transported as VLDL (see below).
Fatty acids
These are mostly straight-chain monocarboxylic acids. They are mainly derived from dietary or tissue triglyceride, but the body can also synthesise most of them, apart from certain polyunsaturated (essential) fatty acids. Fatty acids act as an alternative or additional energy source to glucose.
Phospholipids
These have a structure similar to triglycerides, but a polar group (e.g. phosphorylcholine) replaces one of the three fatty acid components. The presence of both polar and non-polar (fatty acid) groups gives the phospholipids their characteristic detergent properties. Phospholipids are mainly synthesised in the liver and small intestine; they are important constituents of cells, and are often present in cell membranes.
Lipoproteins
Cholesterol and its esters, triglycerides and phospholipids are all transported in plasma as lipoprotein (Table 12.2) particles. Fatty acids are transported bound to albumin.
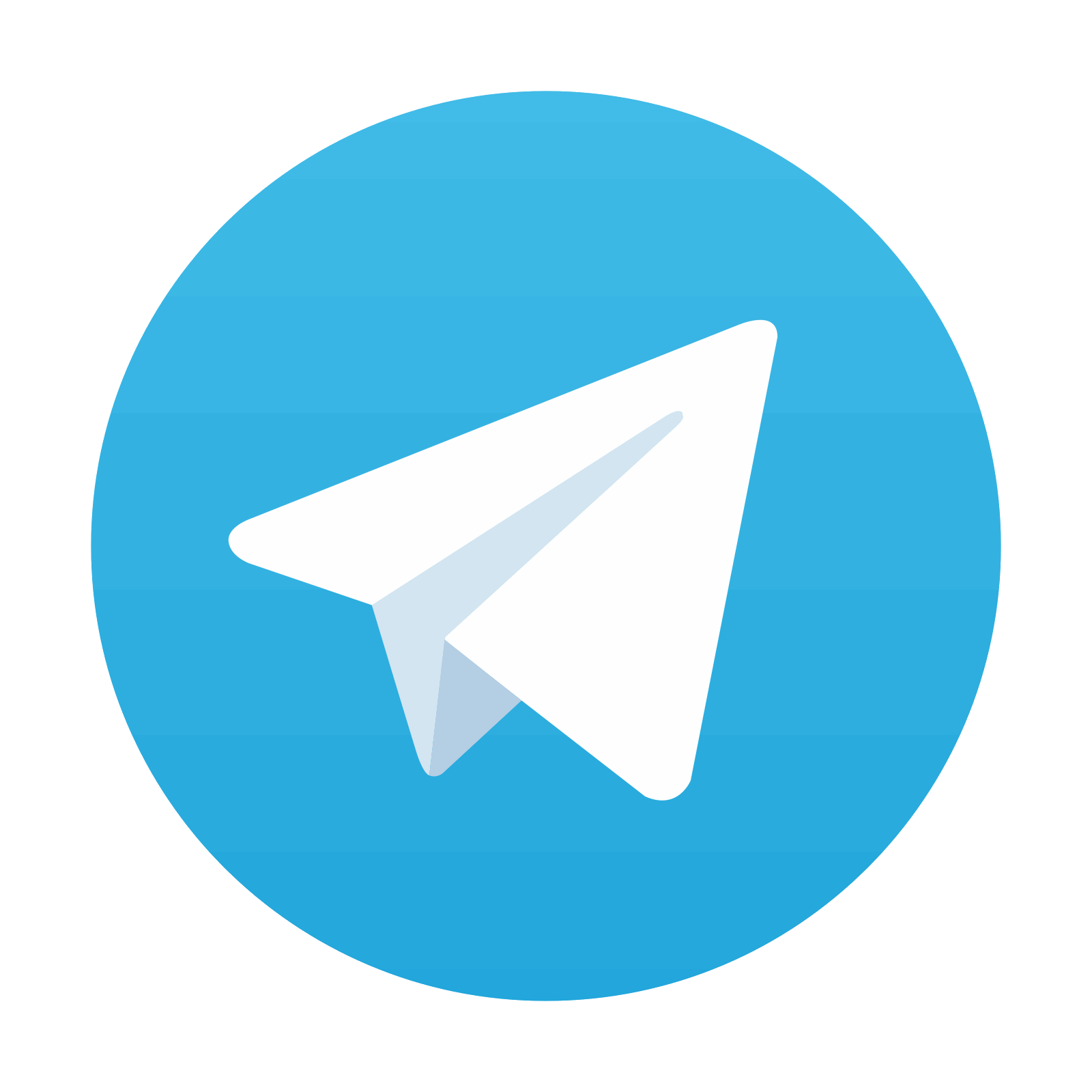
Stay updated, free articles. Join our Telegram channel

Full access? Get Clinical Tree
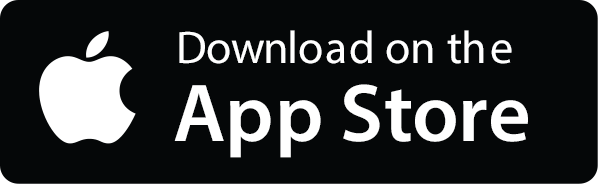
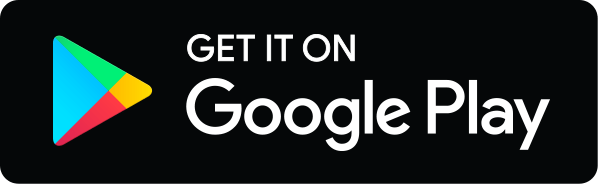