Fig. 1.1
Chemical structure of some exogenous and endogenous agonists of the transient receptor potential vanilloid type-1 (TRPV1) capsaicin receptor
The first paper on studying the pharmacological effects of capsaicin was published by Endre Hőgyes in 1878. His main findings include (1) In humans capsicol as counterirritant does not induce vesiculation in the skin in contrast to cantharidine commonly used at that time; (2) Oral intake of capsicol in gelatine capsules enhances gastrointestinal motility without gustatory effect; (3) In dogs capsaicin induces fall in body temperature. Owing to the lack of effects in various preparations innervated by efferent nerves his main conclusion was that “capsicol acts mainly on sensory nerves” (Hőgyes 1878). Although these observations were published in a well-recognized pharmacology journal they remained unnoticed for more than 60 years and did not form a starting point for the pharmacology of sensory nerve endings.
In striking contrast, at that time similar approaches on the selective actions of natural alkaloids such as curare, ergot alkaloids, nicotine, and atropine paved the way to the mechanism of efferent neurohumoral transmissions which led to the identification of their neurotransmitters of acetylcholine and noradrenaline. Thus the pharmacology of the efferent nervous system started with investigation the effects of these rather toxic herbal compounds. The pharmacology of nociceptors started decades after the discovery that nociceptors are the sites of capsaicin desensitization as described in Ref. (Szolcsányi 2005) (Fig. 1.2).
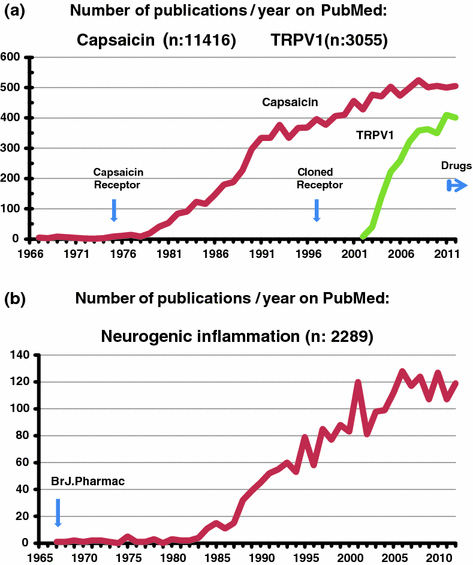
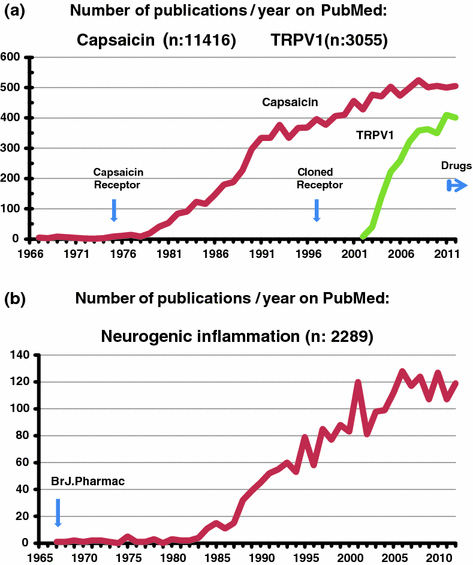
Fig. 1.2
Number of publications at keywords of capsaicin and TRPV1 (a) or neurogenic inflammation (b) indicated in database of PubMed (2013 March); capsaicin receptor: first publication of a hypothetical receptor in 1975 (Szolcsányi and Jancsó-Gábor 1975a); cloned receptor: cloning the capsaicin receptor in 1997 (Caterina et al. 1997). Drugs: first FDA approval for a capsaicin containing drug (Qutenza). Br. J. Pharmac.: first direct evidence for the existence of neurogenic inflammation (Jancsó et al. 1967). For more details see text
1.2 Capsaicin Desensitization
The phenomenon of “capsaicin desensitization” was discovered by Nicholas Jancsó in the 1940s of the last century (Szállási and Blumberg 1999; Szolcsányi 2005, 1984). It was a serendipitious observation in the course of experiments in which he studied the pivotal mediator role of histamine in mediation of inflammation and storage of macromolecules in endothelial and macrophage cells. During these years, antihistaminic drugs were inaccessible and therefore he used in rodents high histamine doses for desensitization of these receptors. He used by chance capsaicin instead of histamine for desensitization because he thought that capsaicin acts as a potent histamine releasing agent. It turned out, however, that the “capsaicin desensitization” is a new phenomenon and it differs from the actions of histamine in its broad-spectrum antinociceptive effect. The perspectives of these early data, however, were not recognized by the editor of Experientia and the manuscript written by N. Jancsó with his wife Aurelia Jancsó-Gábor in 1949 on the discovery of capsaicin desensitization was rejected. Afterward the Jancsó couple focused on their main field of interest on storage of macromolecules in inflammatory cells and in the kidney which were published in the Nature and other journals. However, they never sent another manuscript on capsaicin to international journals. In 1955 Nicholas Jancsó wrote an excellent monograph in German on storage of macromolecules in the reticulonoendothelial system and in the kidney (Jancsó 1955). He took this opportunity to summarize all of his results and concept about “capsaicin desensitization.” Since his views on actions of capsaicin have been often misinterpreted in recent reviews, I quote in English two sentences from the book: (1) “The resistance of eyes desensitized by capsaicin also against acidic, alkalic and even hypertonic salt solutions”… indicates “that the sensory nerve endings become unresponsive to chemical stimuli although their physical excitability remains”; (2) “Those receptors (denselben Receptoren) are apparently desensitization which remain responsive to physically evoked corneal or sneezing reflexes.” In contrast to the desensitization of the nicotinic receptors “synaptotropen Verbindungen” he never considered the existence of a capsaicin receptor on sensory nerve endings (Jancsó 1955).
About 10 years later Jancsó 1964 briefly outlined his views for the last time in English in an abstract of an invited lecture: “Capsaicin induces in rats and guinea pigs a peculiar sensory disturbance lasting for weeks or even years. The animals become insensitive to pain by chemical substances while the perception of pain caused by physical means remains unimpaired. Capsaicin probably interferes with the synthesis of the mediator substance (of neurogenic inflammation JS) in the pain receptor or in the whole neurone. The mediator substance may be a bradykinin-like polypeptide, or the enzyme producing it” (Jancsó 1964).
The mechanism of this “peculiar” phenomenon remained enigmatic and without quantitative published data even his statements were questioned and challenged (Makara et al. 1967). After 4 years of experimental physiological background I joined the Jancsó’s couple to work on capsaicin in 1962. We worked together until his demise in 1966. This period was very fruitful but the results were not summarized, completed and particularly were not prepared for publication. The first paper on capsaicin desensitization was sent to the Br. J. Pharmacol. Jancsó et al. (1967) 1 year later (Fig. 1.2) providing also the first direct evidence for the existence of neurogenic inflammation. Since my view about the role of bradykinin differed from Jancsó’s interpretation, this part of experiments and his quoted conclusion on this aspect were omitted although the data with enhanced bradykinin-like activity obtained from the skin after antidromic nerve stimulation were convincing. I myself made the titration under his supervision on the isolated rat uterus preparation for several months. My impression was that this bradykinin-like activity is increasing in time at room temperature and therefore I attributed it to an enhanced bradykininogen extravasation and not to a release from the nerve endings. Few years later by using also the rat-isolated duodenal preparation, we obtained the first hint of evidence that substance P might be the mediator which is released from the stimulated sensory nerve endings: “The contraction of the rat duodenum could be attributed to the presence of another mediator: e.g. substance P” (Jancsó-Gábor and Szolcsányi 1972).
These results remained unnoticed for 10 years (Fig. 1.2). Neither the selective blockade of chemonociception in capsaicin-pretreated rats nor its blocking effect on neurogenic inflammation initiated further research although the sensory receptor-selective action of capsaicin was documented also by action potential recordings from the saphenous nerve of the capsaicin-desensitized rats (Jancsó et al. 1967). It is worthy to mention that until the mid-1960s there was no unequivocal evidence for the existence of nociceptors (Melzack and Wall 1965) although Sherrington predicted their existence already in 1906 (Sherrington 1906). Thus, it remained elusive whether the long-lasting capsaicin desensitization is a neurotoxic effect which renders sensory nerve terminals in general unresponsive to chemical agents, or the effect is related to a loss of function of a subgroup of sensory receptors, notably nociceptors, which mediate chemonociception but not those which mediate mechano-nociception.
1.3 Selective Effect of Capsaicin on Cutaneous C-Polymodal Nociceptors
C-polymodal nociceptors in the skin, the major subgroup of unmyelinated afferent fibers were discovered by Bessou and Perl in 1969 (Bessou and Perl 1969). The coined name polymodal refers to responsiveness of these sensory end organs to three different modalities: noxious heat, moderate-high mechanical, and chemical (acids) stimuli. First evidence of a selective action of capsaicin on C-polymodal nociceptors was obtained by the capsaicin-evoked selective collision of the C2 compound action potentials of the cat saphenous nerve which could be activated also by noxious heat (Szolcsányi 1977). In addition both electrophysiological and psychophysical evidence for the thermodependence of its sensory effects on animals and humans, respectively provided evidence that capsaicin selectively acts on C-polymodal nociceptors (Szolcsányi 1977). Psychophysical assessments on human tongue and skin also supported this conclusion. Immersion of the tongue into 1 % solution of capsaicin resulted in selective loss of chemonociception evoked by capsaicin, mustard oil or zingerone without altering the recognition threshold concentrations of menthol and taste stimuli evoked by NaCl, quinine, ascorbic acid, and glucose. These results provided the first evidence that capsaicin is not a general chemosensory blocking agent but its effect is restricted to physiologically well-defined groups of sensory receptors identified as the C-polymodal nociceptors. Temperature discrimination limens were inhibited in the warm and hot (44–45 °C) range, but sensation to tactile and cold stimuli remained intact. On the blister base on volar skin, the pain producing effect of capsaicin, bradykinin, or acetylcholine, but not that of potassium chloride solution, was inhibited after topical application of a high, desensitizing concentration of 1 % capsaicin solution (Szolcsányi 1977; Szolcsányi and Pintér 2013). The results provided the first set of evidence for a selective action of capsaicin on nociceptors and for the thermo- and chemoanalgesic effect of capsaicin pretreatment on human skin and tongue (Fig. 1.3).
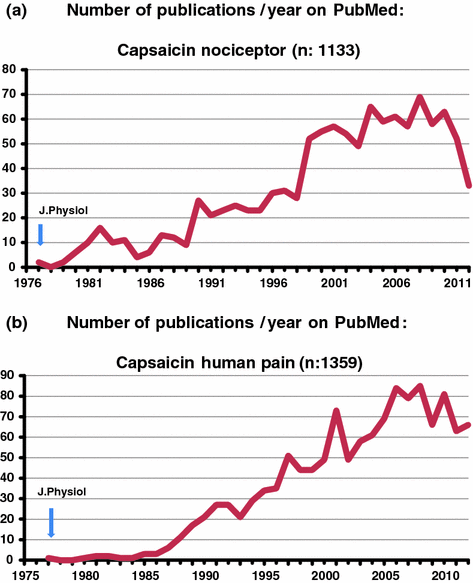
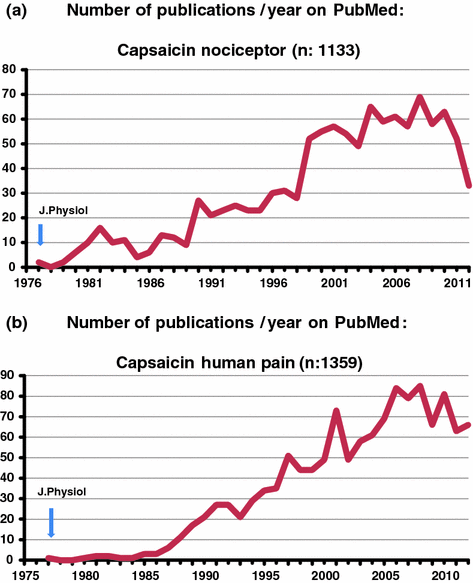
Fig. 1.3
Number of publications at keywords capsaicin nociceptor (a) and capsaicin human pain (b) Arrows first publication on the lists (Szolcsányi 1977)
Excitation and desensitization of cutaneous primary afferent units in the rabbit ear by close arterial injection of capsaicin fully supported its highly selective action on this group of nociceptors (Fig. 1.4). Capsaicin did not evoke over a 100-fold dose range action potentials of C-mechanoreceptors, A-delta (Aδ) mechanical nociceptors, and all types of Aδ and Aβ mechanoreceptors or C-afferent cooling receptors (Szolcsányi 1987, 1980). These sensory receptors were neither desensitized to their natural stimuli after high doses of capsaicin. It was interesting, however, that action potentials of C-polymodal nociceptors to all three modalities of mechanical, chemical (bradykinin, xylene, capsaicin), or noxious heat stimuli were suppressed or blocked. Desensitization of a single C-polymodal unit to one kind of stimulation often was not paralleled by similar changes in responsiveness to other modalities of stimulation and most units still responded to one type of stimulation. Thus, it seems that the transducer processes and not the conducting axons were impaired (Szolcsányi 1993, 1987). Notably, in contrast to earlier findings close arterial injection of bradykinin in low but not in high doses evoked action potentials exclusively on C-polymodal nociceptors but not on other types of afferent fibers (Szolcsányi 1987). Topical application of capsaicin in 50 % DMSO on the skin of rat (Kenins 1982), or on the burn-induced blister base in the cat (Foster and Ramage 1981), and on intact skin of humans (Konietzny and Hensel 1983) or monkeys (Bauman et al. 1991) as well single unit studies after intradermal injection in the rat (Martin et al. 1987) or monkey (Bauman et al. 1991) supported that capsaicin evoked action potentials only on C-polymodal nociceptors, on Aδ mechanoheat (polymodal) nociceptors, and also on mechano-insensitive (MiH) or silent C-nociceptors (Szolcsányi 1993, 1996). Interoceptors with axons conducting in C- and Aδ range and excited by bradykinin and in some cases also by mechanical stimuli were shown to be excited and desensitized by capsaicin. These results have recently been summarized elsewhere (Szolcsányi and Pintér 2013).
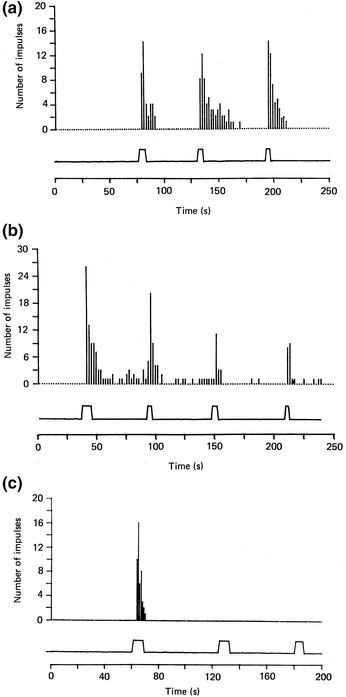
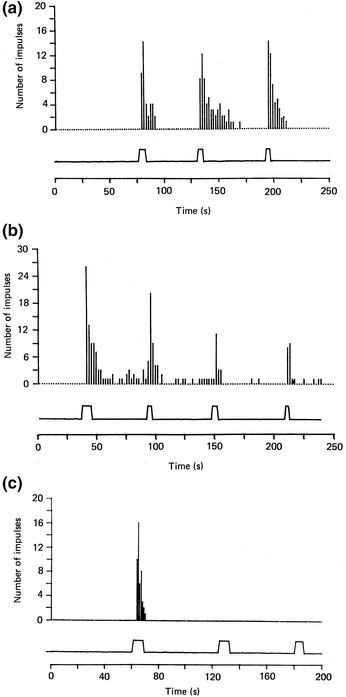
Fig. 1.4
Response of a C-polymodal nociceptor of the rabbit ear to repeated intra-arterial injections of capsaicin. Number of discharges in each 2 s period (a and b) and in 1 s (c). The marks below the graphs indicate the duration of capsaicin injection. Doses: 20 μg (a), 200 μg (b) and 600 μg (c). Note the reproducibility with the small dose and the desensitization after higher doses. (Reproduced from Szolcsányi 1987a with the kind permission of the editors of J. Physiology London)
Beyond the mechano-heat sensitive C-polymodal nociceptors (CMH) in the skin of healthy human subjects, a smaller portion of C-nociceptors were mechanoinsensitive; most of them still responded to noxious heat (CH) but 6/67 units were insensitive to physical stimuli (CMiHi) (Weidner et al. 1999). Nevertheless, both C-polymodal nociceptor (CMH) (LaMotte et al. 1992; Schmelz et al. 2000a) and CH and CMiHi nociceptors were activated by intracutaneous injection of capsaicin (Schmelz et al. 2000a). Furthermore, topical application of mustard oil or capsaicin sensitized the CMi nociceptors and several of them afterward responded to mechanical stimuli (Schmelz et al. 1994). In another study, tonic pressure on the human skin sensitized most of the C-mechano-insensitive units and responded with action potentials after 20 s (Schmidt et al. 2000). It is important to note that there is clear evidence that capsaicin-sensitive CMH and CMi nociceptors mediate itch (Johanek et al. 2008; Han et al. 2013) and are mainly responsible to mediate the axon reflex flare in human skin (Schmelz et al. 2000b). Topical desensitization of the skin with high concentration of capsaicin abolished the itch sensation (Tóth-Kása et al. 1986). It is interesting that axon reflex flare could be evoked also in pigs (Pierau and Szolcsányi 1989), but not in rodents and it is also mediated by CMi fibers (Lynn et al. 1996). After UV-B irradiation these silent nociceptors are sensitized (Rukwied et al. 2008) as C-polymodal nociceptors (Szolcsányi 1987).
Taking together all these findings on the excitatory and blocking effects of capsaicin by recording activity of single sensory fibers the following conclusions can be drawn:
(1)
Physiological well-defined types of sensory units with unmyelinated (C) or thin myelinated (Aδ) fibers are excited by capsaicin. The largest subgroup of these capsaicin-responsive units are the C-polymodal nociceptors but in monkeys, pigs, and humans considerable group of afferents are mechanically insensitive albeit they could be sensitized sometimes under inflammatory conditions to become mechanical responsive (Szolcsányi and Pintér 2013).
(2)
Mechanoreceptors with unmyelinated or myelinated axons cannot be activated and there is no evidence for their long-term blockade after capsaicin application. Cold receptors are neither sensitive to capsaicin.
(3)
After high capsaicin doses responsiveness of C-polymodal nociceptors is inhibited to mechanical, thermal, and chemical stimuli. Complete damage of these nociceptors, however, also could be elicited by higher doses of capsaicin (Szolcsányi and Pintér 2013). Nevertheless, there is functional evidence that after capsaicin pretreatment loss of responsiveness of capsaicin-sensitive nerve terminals is not necessarily due to loss of nerve fibers. Electrophysiological data support different stages of desensitization of the nerve endings to natural stimuli (sensory desensitization) (Szolcsányi 1993; Szolcsányi and Pintér 2013).
(4)
Large group of capsaicin-sensitive sensory receptors release neuropeptides and induce neurogenic inflammation and other efferent tissue responses in internal organs. Thus, they have dual sensory-efferent function (see later).
1.4 Experimental Models for Capsaicin-Induced Blockade of Nociceptors
1.4.1 Terminology
The traditional descriptive term of “capsaicin desensitization” simply refers to the refractoriness of animals, human beings, or in vitro tissue preparations to capsaicin-type agents which is accompanied in rodents by chemoanalgesia and impaired thermoregulation in a warm environment (Szolcsányi 1993; Szolcsányi and Pintér 2013). Owing to the present state of knowledge on operations and modulation of TRPV1 channels the term is now obsolete. It might have been different meanings and therefore should be avoided. Here the long-term functional unresponsiveness of capsaicin-sensitive, TRPV1-expressing neurons and their sensory nerve endings are summarized which can be achieved by high doses of capsaicin or its congeners. Precise mechanisms at the molecular level of unresponsiveness, shift of threshold or thermal enthalpy, potency, or efficacy of agonists, modulators of the gating function of the integrative TRPV1 cation channel to various stimuli are discussed in another chapter of this book and see also recent references (Szolcsányi and Pintér 2013; Szolcsányi and Sándor 2012; Xia et al. 2011; Touska et al. 2011; Planells-Cases et al. 2011). In the pharmacology “desensitization” means a decreasing responsiveness or refractoriness to the action of a chemical agent, as declining gating of the TRPV1 or nicotinic receptors to the respective agonists by recording at cellular level of currents, ion fluxes, or other responses. For decreasing responsiveness to repeated application of an agonist compound in isolated organs or isolated cells, the term tachyphylaxis is used although in some publications they are also called desensitization. The term “desensitization” is an accepted term also in sensory physiology and pharmacology referring to the change of responsiveness of the sense organ to various stimuli (Szolcsányi 1993; Szolcsányi and Pintér 2013). In order to make it clear in this chapter for this functional blockade or diminished responsiveness of the capsaicin-sensitive primary afferent neurons, the term “sensory desensitization” is used. The importance of this differentiation is underlined by the fact that capsaicin-induced sensory desensitization involves all functions of the nociceptive nerve terminals, while pharmacological desensitization of the TRPV1 channel inhibits only those effects which are mediated by this cation channel.
1.4.2 Sensory Desensitization Induced by Capsaicin
Repeated application of capsaicin congeners in near threshold concentrations or low doses in vivo or in vitro induces reproducible effects without desensitization (Szolcsányi 1993; Szolcsányi and Pintér 2013; Szolcsányi and Jancsó-Gábor 1976). At higher concentration ranges, however, a dose-dependent sensory desensitization develops within minutes even under in vivo condition. Figure 1.4 (Szolcsányi 1987) shows that close arterial injection of capsaicin into the rabbit ear in doses of 20 μg elicited similar burst of discharges on a single unit of C-polymodal nociceptor, while a 10 times higher dose induced desensitization and further increment in dose resulted in unresponsiveness to capsaicin, although it still fired to an enhanced level of temperature or mechanical stimuli but not to bradykinin. Close arterial injection of 0.2 μg capsaicin into the hindleg of the rat also evoked reproducible number of spikes on C- or Aδ single unit fibers of polymodal nociceptors (Szolcsányi et al. 1988). Instillation of capsaicin congeners into the eye of rats produced similar reproducible nocifensive wiping responses at low threshold dose range of the compounds and induced sensory desensitization at higher doses. The sensory desensitization evoked by the compound was related to the chemical structure and not to magnitude of the nocifensive reaction. Thus, not excessive stimulation but strong binding of the agonist and lasting opening the cation channel is responsible for the sensory desensitizing effect (Szolcsányi and Pintér 2013; Szolcsányi and Sándor 2012). It is interesting that under these conditions no sensitization was observed although after subcutaneous (s.c.) injection of capsaicin under the skin of rat’s hind paw (Szolcsányi 1977) or after topical capsaicin application on the skin of monkey (Bauman et al. 1991) or in an in vitro skin preparation (Guenther et al. 1999) sensitization of C-polymodal nociceptors to innocuous heat stimuli was observed.
For studying the sensory desensitizing effect of capsaicin there is a large scale of methods used in animal experiment:
(1)
Topical application to the skin or instillation into the eye or applying to other mucosal areas of airways, gastric mucosa, or urinary bladder induces local desensitization. These latter scopes will be discussed in other chapters of the book owing to their potential therapeutic relevance.
(2)
Close arterial injection. It has the advantage of studying dose-related effects with fast onset and short lasting exposure. Results of these treatments have been discussed and an example is shown in Fig. 1.4. Subcutaneous injection for regional sensory desensitization or neurotoxic damage of the cutaneous sensory nerve terminals can be achieved.
(3)
Subcutaneous or intraperitoneal injections of capsaicin in large doses particularly in rats and mice is a common method for inducing long-term systemic sensory desensitization. The lasting effects observed after pretreatment of adult or neonatal animals—as has been compared mainly on rats—are qualitatively different. Therefore, they are discussed separately.
(4)
Perineural application around various nerve trunks in rats, mice, guinea pigs, and other species.
(5)
Intrathecal application.
(6)
In vitro application to preparations of isolated organs, sensory nerve-peripheral tissues, sensory nerve-spinal cord preparation, tissue slices, cultured dorsal or trigeminal neurons and TRPV1 transfected cell lines are commonly used.
(7)
TRPV1 gene-deleted mice and TRPV1 siRNA knockdown mice and recently gene modified animals using Cre-recombinant knock in mice have provided new scopes of evidence for the role of TRPV1 in various functions. Notably, TRPV1 transfected cell lines has become indispensable means in high throughput screening in drug development.
1.4.3 Systemic Application in Adult Animals
In rats the antinociceptive effect of systemic capsaicin pretreatment lasts for several days, weeks or months (Szolcsányi 1993; Buck and Burks 1986; Holzer 1991; Szolcsányi 1987b, 1990). In all reported studies the blockade of chemonociception to large scale of agents was clearly shown, but antinociceptive effect against noxious heat or mechanical stimuli resulted in controversial results. It could be due partly to methodical differences. It was particularly striking that although after topical application on the human skin there was a marked enhancement in noxious heat threshold (Szolcsányi 1990) in animal experiments when testings with conventional models of hot plate, tail flick, radiant heat or plantar tests which detect reflex latencies to suprathreshold stimuli the results were contradictory. Furthermore, often the eye-wiping test to capsaicin (Szolcsányi and Jancsó-Gábor 1976) was taken as a level of “desensitization” to noxious chemical stimuli, while physical stimuli were applied on other parts of the body. The first study (Holzer 1991) to measure the threshold for different nociceptive stimuli on the same skin area of the hind paw led to the following results. After a total s.c. dose of 400 mg/kg given in five successive days, the blockade of chemonociception to xylene was complete for a week, and it remained inhibited for 25 days while the enhanced noxious heat threshold returned slightly earlier. After pretreatment with 150 mg/kg nociceptive thermal threshold increased by 2.5 °C for one day, but returned to the control level on the fourth day. Slight enhancement in the threshold of cutaneous mechanical stimuli lasted only for 1 week (Szolcsányi 1987). Mechanical hyperalgesia due to chronic inflammation, however, was markedly reduced in capsaicin pretreated rats (Barthó et al. 1990). In contrast to all these findings antinociceptive effect of capsaicin pretreatment on the capsaicin-evoked eye-wiping test lasts after a dose of 50 mg/kg over 2 months (Szolcsányi 1993; Szolcsányi and Pintér 2013). Long-lasting antinociceptive effect of systemic capsaicin pretreatment of adult guinea pigs (Buck et al. 1981) and mice (Gamse 1982) were also reported. It is worthy to mention, that birds are not sensitive to the irritant effect of capsaicin and after high dose of close arterial capsaicin injection desensitization to nociceptive chemical stimuli was neither evoked (Szolcsányi et al. 1986).
The long-lasting antinociceptive effect of capsaicin pretreatment is accompanied by severe mitochondrial swelling of the small B-type neurons of the trigeminal or dorsal root ganglia (Joó et al. 1969; Szolcsányi et al. 1975; Chiba et al. 1986). This ultrastructural damage was described also in some corneal nerve endings after topical application of capsaicin (Szolcsányi and Pintér 2013; Szolcsányi et al. 1975) and could explain the functional loss of the affected nociceptors without frank degeneration. Peripheral and central nerve terminals of capsaicin-sensitive primary afferent neurons are more vulnerable to systemic or intra-arterial capsaicin doses. Thus in contrast to the cell body axon terminals but not the dorsal root fibers degenerate after capsaicin pretreatment (Chung et al. 1990, 1985; Pethő and Szolcsányi 1996; Palermo et al. 1981). Furthermore, impaired mitochondrial function with diminished ATP production could explain the characteristic fatigue of responsiveness of the affected sensory receptors (Szolcsányi and Pintér 2013; Szolcsányi and Jancsó-Gábor 1976; Szolcsányi 1990; Szolcsányi et al. 1975). The decreasing level of nocifensiveness to repeated application of a nondesensitizing vanilloid e.g. zingerone should be taken into account to avoid overlooking a partial desensitized state.
1.4.4 Systemic Treatment of Neonatal Rats
It was reported in 1977 by the group of Gábor Jancsó that rats treated s.c. on the second day of life with 50 mg/kg capsaicin produces within an hour massive acute necrotic cell death of small B-type of primary afferent neurons. These animals tested in the adult age failed to respond to capsaicin and in these animals neurogenic inflammation could not be elicited (Jancsó et al. 1977, 1987). One year later Thomas Jessell, Claudo Cuello and Leslie Iversen made the remarkable discovery (Jessell et al. 1978) that capsaicin pretreatment of adult rats selectively depleted the sensory neuropeptide substance P from the sensory ganglia but not from other tissues. Usage of capsaicin in neuropeptide research as a sensory neurotoxin was one of the main reason why interest to capsaicin research increased in the eighties as indicated on Fig. 1.2. For this purpose a model suggesting complete and selective loss of sensory neurons seemed to be more tempting than usage of rats treated in the adult age. Several quantitative morphological studies confirmed the original observation of Gábor Jancsó (1987) in respect of substantial loss of B-type neurons and sensory unmyelinated C-fibers in adult rats after neonatal pretreatment. It turned out, however, that loss of neurons is not restricted to the capsaicin-sensitive, i.e., C-polymodal nociceptive neuronal population and several secondary changes in the peripheral tissues and in the pain pathway was revealed which resulted in contradictory conclusions (Holzer 1991; Cervero and McRitchie 1981). Most importantly single unit studies showed an indiscriminate loss of sensory C-fibers (Welk et al. 1984) and the spectrum of destructed primary afferent neurons depended on the dose (Nagy et al. 1983). Selective degeneration of C-afferents has been described in a lower dose range of 20–30 mg/kg dose and the commonly used 50 mg/kg induced 18 % loss of the myelinated fibers. Furthermore, 34 % loss of large light RT96 labelled A-type neurons was also described (Lawson and Harper 1984). Further secondary changes are reviewed elsewhere (Szolcsányi 2005; Holzer 1991; Szőke et al. 2002a).
Acute necrotic cell death was not observed after neonatal treatment of rats with other TRPV1 agonists as resiniferatoxin (Szállási and Blumberg 1999; Szolcsányi et al. 1990) or anandamide (Szőke et al. 2002b) and in both cases again the selective, pronounced mitochondrial swelling in B-type sensory neurons was striking as in rats treated with capsaicin in adult age. Furthermore, quantitative morphometry provided strong evidence that there is no significant loss of neurons in trigeminal ganglia for 5 days after neonatal capsaicin treatment (Szőke et al. 2002a). The loss of neurons in trigeminal ganglia ensued on the next two weeks, but this loss was completely prevented by daily administration of 100 μg/kg s.c. NGF. The first dose was given one day after the capsaicin injection, to avoid the interference with the acute capsaicin effect.
It has been concluded that after neonatal capsaicin treatment few cells with necrotic or apoptotic signs of degeneration observed shortly after the treatment could be attributed to the asphyxia induced by capsaicin which evokes in this dose range pronounced reflex apnoea, fall in blood pressure and heart rate (Szolcsányi et al. 1990) leading dose dependently to mortality even after adult treatments (Szikszay et al. 1982). After treatment with other TRPV1 agonists as resiniferatoxin (Szolcsányi et al. 1990) or anandamide which do not evoke the Bezold Jarish reflex mitochondrial swelling in small type of neurons of dorsal root or trigeminal ganglia but not cell death was observed. In all cases of TRPV1 agonists these ultrastructural changes lasted for several weeks or months (Szállási and Blumberg 1999; Szolcsányi 1993; Szolcsányi and Pintér 2013; Szőke et al. 2002a; Szolcsányi et al. 1990; Szőke et al. 2002b). Except for these mitochondrial changes there are qualitative differences between the endpoints of adult or neonatal capsaicin treatments. An important difference should be underlined. Biological markers and sensory neuropeptides missing in rats pretreated in the neonatal age summarized in different reviews in several cases are not expressed in capsaicin-sensitive neurons (Buck and Burks 1986; Holzer 1991). Thus, in contrast to the listed data there is no evidence for expressing cholecystokinin, (CCK), vasoactive intestinal polypeptide (VIP), arginine vasopressin, bombesin, or galanin in TRPV1-expressing capsaicin-sensitive neurons (Szolcsányi et al. 1994). Another important point is the effect of capsaicin treatment on wound healing. Trophic lesions in the skin and cornea in rats pretreated at the neonatal but not in adult age were described by Carlo Maggi group (Maggi et al. 1987; Abelli et al. 1993). Wound healing was not affected after perineural capsaicin treatment (Wallengren et al. 1999) although epidermal immunoreactive CGRP and substance P fibers were markedly lost for at least 42 days (Dux et al. 1999).
1.4.5 Perineural Application
After perineural application of 1 % capsaicin the first evidence for a long-lasting functional impairment of capsaicin-sensitive afferent responses proposed to be due to substance P depletion was reported by Jancsó et al. (1980). Subsequent single unit studies revealed that after a non-selective axonal blockade of this high concentration of capsaicin lasting for 1–3 days, selective loss of C-polymodal nociceptors and an increased noxious heat threshold of the remaining units are together being responsible for the analgesic effect of this type of capsaicin treatment (Petsche et al. 1983; Pini et al. 1990; Szolcsányi 1993; Szolcsányi and Pintér 2013). The advantage of localized degeneration of capsaicin-sensitive afferents and the long-lasting effect due to degeneration of the fibers made this technique popular and was tested on sciatic, saphenous, or vagal nerve trunks mainly on rats but occasionally also on the guinea-pig, ferret, rabbit, cat, and monkey (Szolcsányi 1993; Szolcsányi and Pintér 2013). After 1 % capsaicin application significant antinociception against noxious heat or mechanonociceptive stimuli lasted over 10 days (Szolcsányi 1987). In the concentration range of 0.1–1.5 % long-lasting depletion of substance P was reported (Wall 1987). Furthermore, for a potential therapeutical usage of the highly potent resiniferatoxin (RTX), perineural application was proposed in a concentration of 0.001 % which produced noxious heat analgesia for 2 weeks (Kissin 2008). In a recent publication (Browning et al. 2013), however, after perivagal capsaicin application degeneration of vagal efferent motoneurons was also demonstrated suggesting a “critical re-evaluation” in this field. Mechanism of neurotoxicity evoked by high concentration of capsaicin or RTX applied on nerve trunks remained enigmatic and could not be explained on the basis of cytotoxicity described in vitro when high concentration of capsaicin was applied to overexpressed cell lines or to cultured neurons of sensory ganglia (Kissin 2008).
1.4.6 Local Desensitization by Subcutaneous Pretreatment
Topical application of capsaicin is already in the analgesic therapy for some neuropathic and osteoarthritic pain states (Szolcsányi and Pintér 2013). Therefore this scope is discussed in other chapters of this book including the long-term loss of epidermal sensory fibers after subcutaneous injection of capsaicin in humans (Szolcsányi 1993; Szolcsányi and Pintér 2013). Under experimental condition in the rat, sc. injection of capsaicin (5 μg/50 μl) induced enhancement of noxious heat and mechanical nociception for 2 weeks (Szolcsányi 1987). In respect of noxious heat and noxious cold threshold changes induced, intraplantar injection of capsaicin, resiniferatoxin and N-oleoyldopamine (OLDA) were tested for several days. It has been found that injection of TRPV1 agonists of capsaicin and RTX induced an enhanced noxious heat threshold and shifted down the noxious cold threshold providing a clear desensitizing effect in a dose dependent manner. The recovery from the cold antinociception was, however, faster than that of the hot one indicating probably that the sensory desensitizing/damaging effect of the nerve endings lasted not as long as the vanilloid-induced diminished function of the noxious heat responsive TRPV1 thermotransducer. OLDA failed to elevate noxious heat threshold indicating its low sensory desensitizing potency (Bölcskei et al. 2010). Plantar incision-induced heat hyperalgesia was reduced by infiltration the plantar region by 0.025 and 0.1 % capsaicin similarly as after perineural capsaicin pretreatment while mechanical hyperalgesia was only slightly influenced (Hamalainen et al. 2009).
1.4.7 Intrathecal Application
The prolonged potent antinociceptive action of intrathecal capsaicin combined with depletion of substance P in the dorsal horn reported by the group of Tony Yaksh provided in 1979 the first evidence for the blockade of central terminals of capsaicin-sensitive nociceptors (Yaks et al. 1979). Damaged glomerular C-type terminals of the dorsal horn localized with ultrastructural technique proved the selective site of action (Palermo et al. 1981). Piperine and nonanoyl vanillyamide together with capsaicin enhanced the tail flick latency in parallel with the depletion of substance P and somatostatin, while the less pungent capsaicin congeners were less effective or ineffective after central application (Micevych et al. 1983) similarly as at the peripheral terminals for inhibition the eye-wiping responses (Szolcsányi and Jancsó-Gábor 1975). Structure–activity relationship between the potency of immediate neuropeptide release and long-term antinociceptive effect was observed (Jhamandas et al. 1984). The highly potent analgesic effect of intrathecal RTX application has been revealed in our laboratories in 1993 (Szolcsányi et al. 1993). More recent detailed analysis and therapeutical perspectives of intrathecal RTX application is discussed in a separate chapter of this book.
1.4.8 Effect on In Vitro Preparation
Until the mid sixties of the last century few papers were published on the in vitro effects of capsaicin on isolated preparations from mammals or on microorganisms (Fig. 1.5) (Molnár 1965; Szolcsányi 1982). Smooth muscle responses already reported seemed to me interesting to reveal some new type of neurogenic mechanisms mediated by some capsaicin-sensitive interoceptors. The first in vitro evidence reported in 1978 fully supported this hypothesis. Capsaicin selectively stimulated and subsequently abolished for hours the function of nerve endings of extrinsic neurons which elicited a new type of neural efferent mechanism (Szolcsányi and Barthó 1978; Barthó and Szolcsányi 1978). This unorthodox dual “sensory-efferent” function of “capsaicin-sensitive” nerve endings (Fig. 1.5) turned out to revise the classical axon reflex theory (Fig. 1.6) and will be discussed under a separate subheading. Subsequently several in vitro studies were reported on the role of substance P and other neuropeptides and about their possible mediator role in the spinal dorsal horn of the nociceptive pathway. Wide range of reports are summarized in several reviews (Buck and Burks 1986; Holzer 1991; Maggi 1995). The enhanced interest on capsaicin in the eighties (Fig. 1.2) could be attributed mainly to the high interest in neuropeptide-related research.
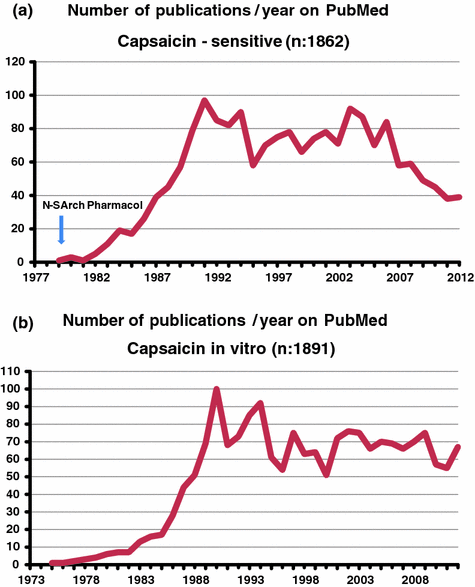
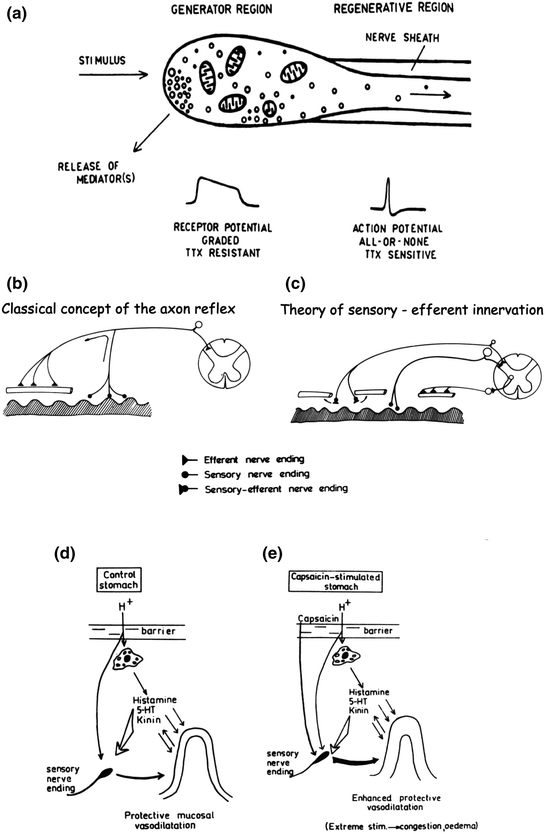
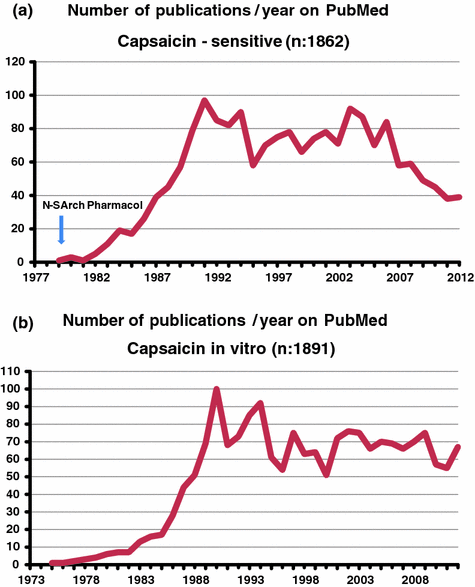
Fig. 1.5
Number of publications at keywords capsaicin–sensitive (a) and capsaicin in vitro (b). Arrow first publication on the list (Szolcsányi and Barthó 1979)
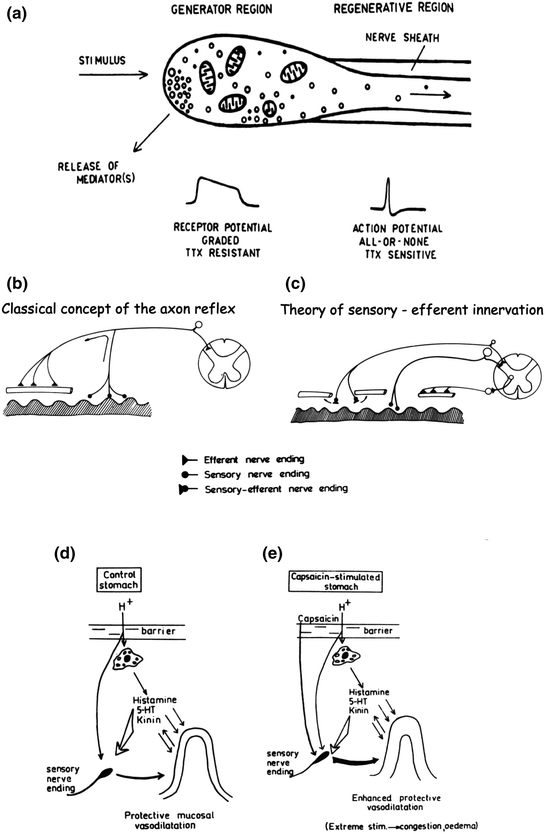
Fig. 1.6
a Theory of dual sensory-efferent function of capsaicin-sensitive nociceptors (Reproduced from Szolcsányi 1988 with the kind permission of Birkhäuser Publishing House). b Original concept of axon reflex theory. c Revised axon reflex theory (Reproduced from Szolcsányi 1984 with the kind permission of Akadémiai Kiadó, Budapest). d Role of capsaicin-sensitive nerve endings in gastroprotection. e Enhanced protection of gastric mucosa by intake of low concentration of capsaicin (Reproduced from Szolcsányi and Barthó 1981 with the kind permission of Akadémiai Kiadó, Budapest)
The first evidence that capsaicin (1–10 μM) selectively depolarizes and evokes spikes on dorsal root ganglion cells supplied by C fibers was reported by Heyman and Rang in (1985). A study on cell culture of dorsal root ganglia (DRG) cells revealed earlier a major subgroup of neurons which were selectively activated by bradykinin and as tested in some cases also to capsaicin (Baccaglini and Hogan 1983). The selective depolarization of vagal sensory C-fibers and cell bodies due to enhanced conductance to sodium and calcium ions was subsequently described together with the calcium-induced in vitro neurotoxic effect documented with ultrastructural pictures (Marsh et al. 1987). Furthermore, several seminal papers published by the Sandoz group from London analysed in detail the ion fluxes and their biochemical consequences (Wood et al. 1988; Winter et al. 1990) and electrophysiological effects of capsaicin (Bevan and Szolcsányi 1990; Bevan and Docherty 1993). In a rat saphenous nerve–skin preparation in vitro the selective excitatory effect of capsaicin on polymodal nociceptors was observed (Seno and Dray 1993) in accordance with the in vivo data discussed earlier. Threshold concentration of capsaicin was around 100 nM on C-MH and Aδ-MH (polymodal) fibers and up to 1–3 μM no other types of sensory receptors were excited.
Another seminal observation in studies on phorbol esters made by Peter Blumberg around the turn of the 1990s was the discovery that resiniferatoxin (RTX) a tricyclic diterpene isolated from the fresh latex of Euphorbia resinifera differs in actions from other phorbol esters and evoked responses on nociception and thermoregulation which resembled that induced by capsaicin. Capsaicin and RTX molecule share a common vanilloid moiety linked to apolar regions (Fig. 1.1). After the first in vivo studies were completed, a common spectrum of effects of these vanilloids revealed that RTX has several orders higher potency than capsaicin, but showed also some special features (Szolcsányi et al. 1990; De Vries and Blumberg 1989; Szállási and Blumberg 1989. Thus, RTX has become a promising tool and Árpád Szállási with Peter Blumberg introduced a H3RTX binding technique and initiated a series of extensive investigations on the “vanilloid receptor” (Szállási and Blumberg 1990). Full account of these achievements (Szállási and Blumberg 1999) are discussed in two other chapters of this book. Nevertheless, it is worthy to mention that as early as in 1990 single patch recording from neurons of dorsal root ganglia showed evidence that capsaicin and RTX apparently gated the same ion channel (Bevan and Szolcsányi 1990). Furthermore, sympathetic neurons, neurofilament-containing (A-type) neurons and non-neural cells were not sensitive to RTX or capsaicin and the two compounds acted on the same cells (Winter et al. 1990) as an evidence also on a spinal cord-tail preparation (Dickenson et al. 1990).
Intrathecal application of capsaicin revealed the important scope of action of capsaicin on the central terminals of the capsaicin-sensitive afferents in the spinal cord (Dickenson et al. 1990). Two in vitro electrophysiological studies first supported these observations. The excitatory effect of capsaicin (10–20 μM) in a spinal cord slice preparation was followed by a loss of slow excitatory postsynaptic potentials (EPSP) (Urbán et al. 1985). In the isolated spinal cord of the neonatal rat dorsal root stimulation evokes a ventral root reflex on the conralateral side. The slow component of the ventral root reflex but not the fast one was abolished after local (1 μM for 30 min) or systemic (50 mg/kg s.c. 2 days before) capsaicin pretreatment (Akagi et al. 1985). More recently it has been described that capsaicin (1 μM) increased the glutaminergic miniature EPSP postsynaptic currents in lamina II dorsal horn second order nociceptive neurons while the amplitudes of the “inhibitory postsynaptic potentials” (IPSP-s) were inhibited (Pan and Pan 2004) or according to another study the GABAergic miniature IPSP-were not changed (Kim et al. 2009).
1.4.9 Action of Capsaicin on the Brain
The first functional and morphological evidence that capsaicin has a site of action in the brain was obtain more than 40 years ago within the series of analysing the thermoregulatory effects of capsaicinoids (Jancsó-Gábor et al. 1970a; Szolcsányi et al. 1971. This aspect will be discussed under a separate subheading since it forms the major obstacles for developing TRPV1 antagonist due to the common hyperthermic side effect of some otherwise promising drug candidates. Morphological evidence using radioimmunoassay, H3RTX binding and in situ hybridization detections of TRPV1 mRNA resulted in positive effects in various brain regions although quantitative estimations revealed about a 30 times lower level of expression in brain areas than in the sensory ganglia (Caterina 2007). Using the TRPV1 reporter mice a highly sensitive technique TRPV1 was detected only in the posterolateral hypothalamus with strong presence in primary sensory ganglia (Cavanaugh et al. 2011). From the functional aspect particular attention was paid to sites of the descending inhibitory pain pathway and on the hippocampus. These unsettled issues on the actions of capsaicin in the brain are discussed in another chapter and in several recent reviews (Kauer and Gibson 2009; Steenland et al. 2006; Palazzo et al. 2008).
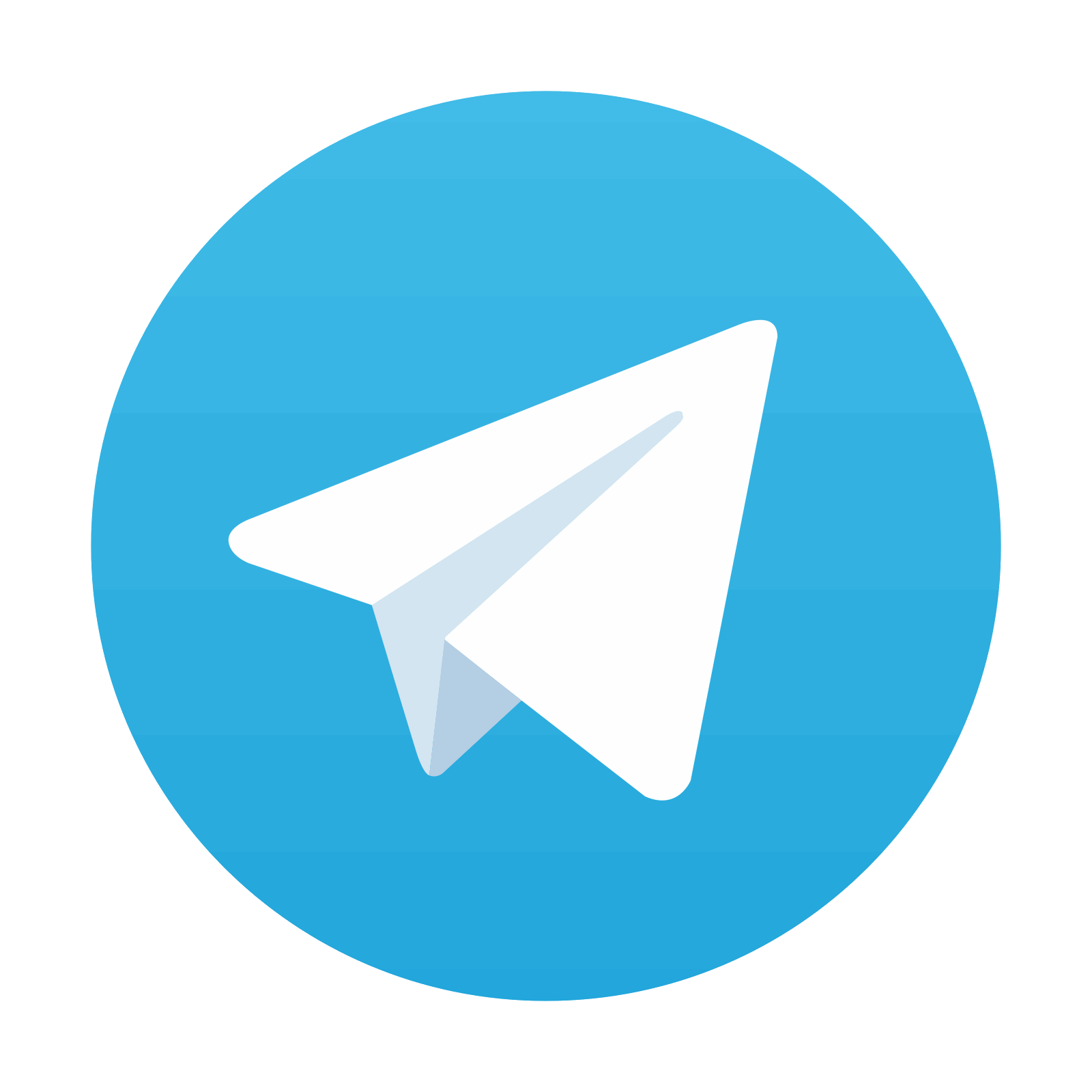
Stay updated, free articles. Join our Telegram channel

Full access? Get Clinical Tree
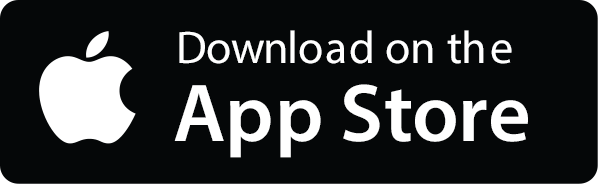
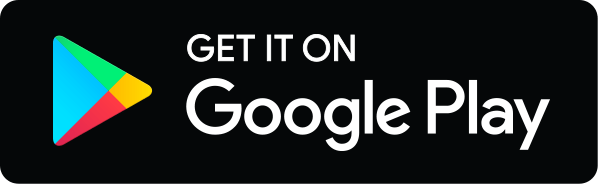