Fig. 42.1 Regulation of calcium metabolism.
A fall in plasma Ca2+ leads to increased release of parathyroid hormone (PTH) from the parathyroid gland, which increases calcitriol [1,25-(OH2)-D3] formation in the kidney. This in turn increases gut absorption of Ca2+. PTH further increases bone mobilisation of Ca2+ to return plasma Ca2+ to normal. An increase in plasma Ca2+, conversely, decreases PTH secretion. Calcitonin, secreted by the thyroid, decreases Ca2+ reabsorption from the kidney and decreases bone turnover. Drugs used for hypercalcaemia are indicated by green arrows and drugs for hypocalcaemia by red arrows.
PTH is a polypeptide hormone which is the main physiological regulator of Ca2+ in blood. Its secretion from parathyroid chief cells is stimulated by a reduction of ionised Ca2+ in plasma. PTH secretion is inhibited when the plasma Ca2+ concentration rises.
The main actions of PTH relating to calcium homeostasis are:



The effect of PTH on the kidney occurs within minutes of PTH release, whereas that on bone begins after 1–2 h.
Vitamin D (calciferol) is a group of compounds that have secosteroid nuclei (a steroid nucleus with one bond in the steroid ring broken). There are two precursors of active vitamin D. Ergocalciferol (vitamin D2) is derived from food and absorbed from the gut. However, given adequate ultraviolet B sunlight, the major source of vitamin D is conversion of 7-dehydrocholesterol in the skin to cholecalciferol (vitamin D3). Therefore, vitamin D is really a skin-derived hormone rather than a vitamin but this source was discovered after the dietary origins. Vitamins D2 and D3 are further metabolised in the liver to 25-hydroxyvitamin D3 (calcidiol), and then in the kidney to 1,25-dihydroxyvitamin D3 (calcitriol). 1α-Hydroxylation is an essential step for activation of vitamin D, and PTH stimulates 1α-hydroxylase activity in the kidney, increasing the formation of calcitriol. Calcitriol binds to specific vitamin D receptors in the target cell nucleus (Ch. 1) and the vitamin D–receptor complex acts as a transcription factor that increases the synthesis of Ca2+ transport proteins in the gut. The main effect of active forms of vitamin D is to increase the plasma concentration of Ca2+ by:
In the kidney, vitamin D promotes phosphate retention, in contrast to the action of PTH. Therefore, vitamin D maintains the plasma Ca2+ and phosphate concentrations to allow normal osteoblast function. These actions of vitamin D affect Ca2+ turnover in bone over periods of days to weeks.
Calcitonin is a peptide secreted by the parafollicular cells of the thyroid when its calcium-sensing receptors detect a rise in plasma Ca2+. The main target cell for calcitonin is the osteoclast, which it inhibits by stimulation of adenylyl cyclase, thus reducing bone turnover. Calcitonin also decreases Ca2+ and phosphate reabsorption by the kidney, thereby increasing their renal excretion.
Physiology of bone turnover
Bone is constantly undergoing remodelling (bone turnover), which involves resorption and replacement of small areas of bone. Up to 10% of bone undergoes remodelling at any point in time, and trabecular bone (in the ends of long bones and in the vertebrae) undergoes greater turnover than cortical bone. Resorption leaves trenches on the bone surface and osteoclasts recruit osteoblasts to refill the trenches. This process is essential for maintenance of Ca2+ homeostasis, replacement of apoptotic osteoclasts and repair of microfractures. There are many factors that regulate bone turnover, but the final pathway is via the balance between RANKL expressed by osteoblasts and activated T- and B-lymphocytes, and osteoprotegerin expressed by osteoblasts (see above). The balance between osteoblast and osteoclast activity and therefore the extent of bone remodelling is modulated by an interaction between various cells in the immune system (lymphocytes and dendritic cells), cytokines and circulating hormones.
Hypercalcaemia
The main causes of hypercalcaemia are:

Hypercalcaemia occurs when the mobilisation of Ca2+ into the extracellular space exceeds the capacity to remove it. Chronic moderate hypercalcaemia leads to a progressive decline in renal function, formation of renal stones and ectopic calcification (e.g. cornea, blood vessels). Severe hypercalcaemia causes anorexia, nausea, vomiting, constipation, drowsiness and confusion, eventually leading to coma. Hypercalcaemia impairs the ability of the kidney to reabsorb salt and water which in conjunction with vomiting can lead to depletion of plasma volume and pre-renal failure. Urgent treatment is indicated when the plasma Ca2+ concentration rises above 3.5 mmol⋅L−1 (normal <2.6 mmol⋅L−1), since sudden death from cardiac arrest can occur.
Antiresorptive drugs for hypercalcaemia
Mechanisms of action and effects: Bisphosphonates are pyrophosphate analogues that bind to hydroxyapatite crystals in bone matrix. They are preferentially deposited under osteoclasts, and are taken up by these cells and inhibit their resorptive action on bone. There are two different cellular actions of the drugs on osteoclasts, depending on the structure of the bisphosphonate.

The actions of most bisphosphonates are relatively short-lived unless taken regularly, but zoledronic acid can suppress bone resorption for up to a year after a single dose.
Pharmacokinetics: Bisphosphonates are poorly absorbed from the gut, and oral formulations are best taken once weekly with the stomach empty to avoid binding by Ca2+ in food. Alendronic acid and risedronate sodium are only available in oral formulations while disodium pamidronate and zoledronic acid are only formulated for intravenous use. Removal of most bisphosphonates from blood via the kidney is rapid, but their effect is prolonged since a fraction remains tightly bound to Ca2+ in bone.


Calcitonin
Mechanism of action and effects: The actions of calcitonin on bone and the kidney to reduce plasma Ca2+ concentrations have been discussed above. Calcitonin begins to act within a few hours of administration, with a maximum effect within 12–24 h. However, the hypocalcaemic effect produced by repeated administrations only lasts between 2 and 3 days. The loss of clinical response results from downregulation of calcitonin receptors on osteoclasts, leading to a rebound increase in bone resorption.
Pharmacokinetics: Salmon calcitonin (salcatonin) is usually given by intramuscular or subcutaneous injection, although intravenous infusion can be used. The half-life is very short (about 20 min) as it is degraded to inactive fragments in the plasma and the kidney.
Treatment of hypercalcaemia
When possible, the primary cause should be corrected, for example removal of a parathyroid adenoma or treatment of myeloma. Oral Ca2+ supplements, vitamin D and thiazide diuretics should be discontinued. Additional measures may include correction of dehydration, enhancing renal excretion of Ca2+ and inhibiting bone resorption.
Most people with severe hypercalcaemia are fluid-depleted at presentation. Rehydration with intravenous isotonic saline is essential; this also promotes a sodium-linked Ca2+ diuresis in the proximal and distal renal tubules. Loop diuretics such as furosemide (Ch. 14) increase renal Ca2+ elimination but should only be given with high volumes of intravenous isotonic saline and intensive monitoring of fluid balance to avoid dehydration.
A bisphosphonate such as disodium pamidronate or zoledronic acid by intravenous infusion is the drug treatment of first choice for severe hypercalcaemia. Initial intravenous rehydration is essential to avoid precipitation of calcium bisphosphonate in the kidney. Oral bisphosphonate treatment may be sufficient for less severe hypercalcaemia. Following a single intravenous infusion of bisphosphonate the plasma Ca2+ concentration falls gradually after 2–4 days, with a maximum effect after 4–7 days and a response that persists for 1–4 weeks after treatment. Because of the delay in onset of action of the bisphosphonates, calcitonin can be given concurrently for an early effect. Corticosteroids such as prednisolone (Ch. 44) are effective for lowering plasma Ca2+ when vitamin D excess is an important factor, for example in sarcoidosis and for acute treatment of vitamin D overdose, or for hypercalcaemia associated with haematological malignancy such as myeloma or lymphoma. Corticosteroids probably act by reducing the effect of vitamin D on intestinal Ca2+ transport, but can take several days to work.
Hypocalcaemia
There are two major underlying causes of hypocalcaemia:
< div class='tao-gold-member'>
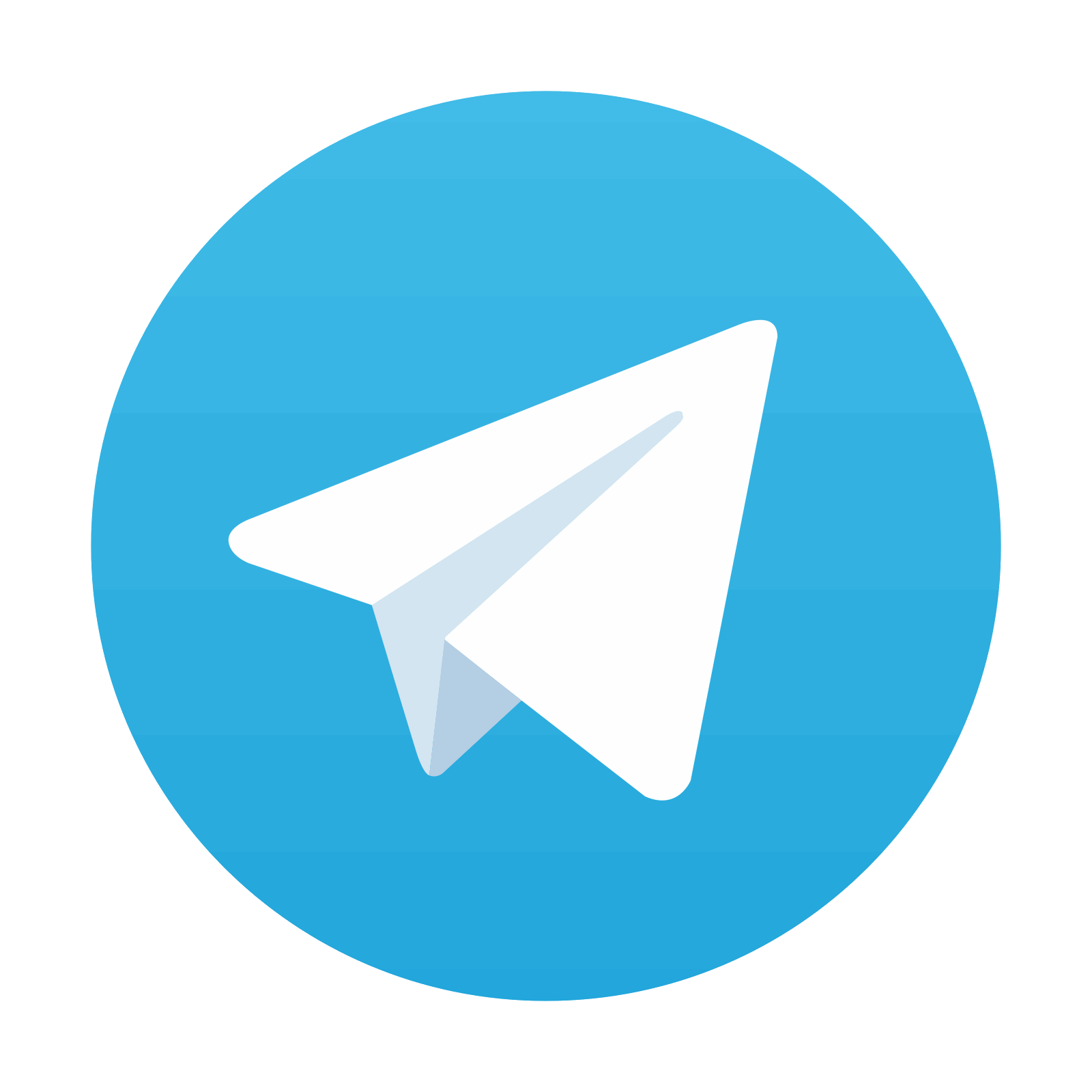
Stay updated, free articles. Join our Telegram channel

Full access? Get Clinical Tree
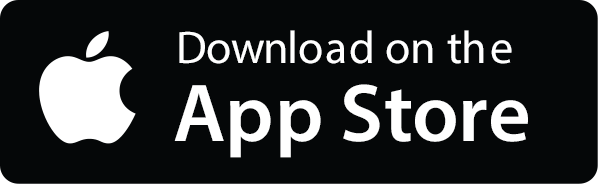
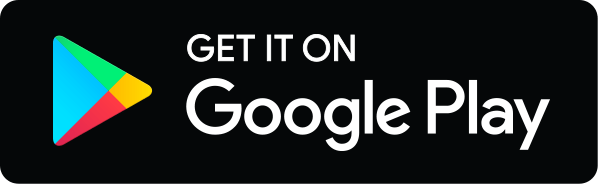