1 Bones, Ligaments, and Joints
1.1 The Skeleton of the Trunk

A Skeleton of the trunk, anterior view
The skeleton of the trunk is made up of the vertebral column, ribs, and sternum. Details on the ribs and sternum are given on p. 134.

C The spinous processes as anatomical landmarks
Posterior view. The spinous processes of the vertebrae appear as variable prominences beneath the skin and provide important landmarks during the physical examination. With few exceptions, they are easily palpated.
• The spinous process of the seventh cervical vertebra, located at the junction of the cervical and thoracic spine. Usually it is the most prominent of the spinous processes, causing the seventh cervical vertebra to be known also as the vertebra prominens.
• The spinous process of the third thoracic vertebra, located on a horizontal line connecting the scapular spines
• The spinous process of the seventh thoracic vertebra, located at the level of the inferior angles of the scapulae
• The spinous process of the twelfth thoracic vertebra, located slightly below the attachment of the last rib
• The spinous process of the fourth lumbar vertebra, located on a horizontal line connecting the highest points of the iliac crests
Note: The spinous processes of the thoracic vertebrae are angled downward (see p. 112), so the spinous process of the fifth thoracic vertebra, for example, is at the level of the sixth thoracic vertebral body.
1.2 The Bony Spinal Column

A The bony spinal column
a Anterior view, b posterior view, c left lateral view.
Note: Phylogenetically, the transverse processes of the lumbar vertebrae are rudimentary ribs. They therefore are known as costal processes (see also p. 108).
B Regions and curvatures of the spinal column
Left lateral view. The spinal column of an adult is divided into four regions and presents four characteristic curvatures in the sagittal plane. These curves are the result of human adaptation to upright bipedal locomotion, acting as springs to cushion axial loads. The following regions and curvatures are distinguished in the craniocaudal direction:
• Cervical spine – cervical lordosis
• Thoracic spine – thoracic kyphosis
• Lumbar spine – lumbar lordosis
• Sacral spine – sacral kyphosis
The cervical, thoracic, and lumbar regions of the spinal column are also known collectively as the presacral spine. The transitional areas between the different regions are of clinical importance because they are potential sites for spinal disorders (e.g., herniated disks). Occasionally, the vertebrae in these transitional areas have an atypical morphology that identifies them as transitional vertebrae. This is particularly common at the lumbosacral junction, where sacralization or lumbarization may be seen, depending on the appearance of the atypical vertebra. With lumbarization, the first sacral vertebra is not fused to the sacrum and constitutes an extra lumbar vertebra. With sacralization, there are only four lumbar vertebrae, the fifth being “sacralized” by fusion to the sacrum. These assimilation disorders are often unilateral (hemilumbarization, hemisacralization).

C Integration of the spinal column into the pelvic girdle
Skeleton of the trunk with the skull and pelvic girdle, left lateral view. Normally, the spinal column is curved and integrated into the pelvic girdle in such a way that characteristic angles are formed between certain imaginary lines and axes. These angles and lines are useful in the radiographic evaluation of positional abnormalities and deformities of the spine and trunk.
Lumbosacral angle: angle formed by the axes of the L 5 and S 1 vertebrae, averaging 143°. It results from the fact that the sacrum is a fixed component of the pelvic ring (see p. 140) and thus contributes little to straightening the vertebral column. The result is a characteristic sharp angle at the junction of the presacral part of the spinal column with the sacrum.
Sacral angle: angle between the horizontal plane and the superior surface of the sacrum, averaging approximately 30°
Pelvic inclination angle: angle formed by the pelvic inlet plane (connecting the sacral promontory to the upper border of the symphysis) with the horizontal. It measures approximately 60° in upright stance. The pelvic inclination angle increases or decreases as the pelvis is tilted forward or backward (see p. 159). With an ideal pelvic position in upright stance, the anterior and posterior superior iliac spines are at the same horizontal level, and the anterior superior iliac spine is directly above the pubic symphysis. By knowing this, the examiner can easily evaluate the position of the pelvis by using palpable bony landmarks.
Line of gravity: the line of gravity passes through landmarks that include the external auditory canal, the dens of the axis (C 2), the functional-anatomical transition points in the spinal column (between lordosis and kyphosis), and the whole-body center of gravity just anterior to the sacral promontory
1.3 Development of the Spinal Column


A Development of the spinal column (weeks 4–10)
a Schematic cross section, b–e schematic coronal sections (the plane of section in b–e is indicated in a).
a, b The former somites have differentiated into the myotome, dermatome, and sclerotome. The sclerotome cells separate from the other cells at 4 weeks, migrate toward the notochord, and form a cluster of mesenchymal cells around the notochord (anlage of the future spinal column).
c Adjacent cranial and caudal sclerotome segments above and below the intersegmental vessels join together and begin to chondrify in the sixth week, displacing the notochord material superiorly and inferiorly (notochord segments).
d The intervertebral disks with their nucleus pulposus and anulus fibrosus develop between the rudimentary vertebral bodies. Ossification begins at the center of the vertebral bodies in the eighth week of development.
e By fusion of the caudal and cranial sclerotome segments, the segmentally arranged myotomes interconnect the processes of two adjacent vertebral anlages, bridging the gap across the intervertebral disks. This is how the motion segments are formed (see p. 126). The segmental spinal nerve courses at the level of the future intervertebral foramen, and the intersegmental vessels become the nutrient vessels of the vertebral bodies (week 10).
Clinical aspects: If the neural tube or the dorsal portions of the vertebral arches fail to close normally during embryonic development, the result is spina bifida—a cleft spine. In this anomaly, the spinal column is open posteriorly, and the spinous processes are absent (the various forms and manifestations are described in textbooks of embryology). Usually there is a bilateral defect in the vertebral arches (generally affecting the L 4 and L 5 region), known as spondylolysis. This defect may be congenital or acquired (e.g., due to trauma). Acquired cases are common in sports that pose a risk of vertebral arch fractures (javelin throwing, gymnastics, high jumping). If the associated intervertebral disk is also damaged, the vertebral body will begin to slip forward (spondylolisthesis). In cases of congenital spondylolysis (which are associated with varying degrees of spondylolisthesis), the slippage progresses slowly during growth, and the condition tends to stabilize after 20 years of age.
B Neonatal kyphosis
Midsagittal section through a newborn, left lateral view. Owing to the curved intrauterine position of the fetus, the newborn has a “kyphotic” spinal curvature with no lordotic straightening of the cervical and lumbar spine (after Rohen, Yokochi, Lütjen-Drecoll).

C Straightening of the spine during normal development (after Debrunner)
The characteristic curvatures of the adult spine are only partially present in the newborn (compare with B) and appear only during the course of postnatal development. First cervical lordosis develops to balance the head in response to the growing strength of the posterior neck muscles. Lumbar lordosis develops later as the child learns to sit, stand, and walk. The degree of lordosis increases until the legs can be fully extended at the hips, and it finally becomes stable during puberty. A similar transformation of the spinal column is observed in the phylogenetic transition from quadrupedal to bipedal locomotion.

D Physiological curvatures of the adult spinal column
Midsagittal section through an adult male, left lateral view.
1.4 The Structure of a Vertebra

A Structural elements of a vertebra
Left posterosuperior view. All vertebrae except the atlas and axis (see p. 111) consist of the same basic structural elements:
• A vertebral body
• A vertebral arch
• A spinous process
• Two transverse processes (called costal processes in the lumbar vertebrae)
• Four articular processes
The processes give attachment to muscles and ligaments, and the bodies of the thoracic vertebrae have costovertebral joints. The vertebral bodies and arches enclose the vertebral foramen, and all of the vertebral foramina together constitute the vertebral (spinal) canal.

B Accessory ribs
Superior view. a Cervical rib, b lumbar rib.
The presence of anomalous cervical ribs can narrow the scalene interval, causing compression of the brachial plexus and subclavian artery (scalenus syndrome or cervical rib syndrome, see also p. 364). An accessory lumbar rib, on the other hand, has no adverse clinical effects.

C Costal elements in different regions of the spinal column
Superior view. The shape and configuration of the vertebrae are closely related to the development of the ribs and their rudiments (indicated here by color shading).
a Cervical vertebrae: Here the rudimentary rib forms a process called the anterior tubercle. It unites with the posterior tubercle to form the transverse foramen.
b Thoracic vertebrae: Because these vertebrae give attachment to the ribs, their bodies and transverse processes bear corresponding cartilage-covered articular surfaces (costal facets on the transverse processes, also superior and inferior costal facets).
c Lumbar vertebrae: The costal elements in the lumbar spine take the form of “transverse processes,” which are much larger than in the cervical spine. Because of their size, they are also known as costal processes.
d Sacrum: Here the rudimentary rib forms the anterior portion of the lateral part of the sacral vertebra. It is fused to the transverse processes.
D Typical vertebrae from different regions of the spinal column
Superior and left lateral view.
a, b First cervical vertebra (atlas).
c, d Second cervical vertebra (axis).
e, f Fourth cervical vertebra.
g, h Sixth thoracic vertebra.
i, j Fourth lumbar vertebra.
k, l Sacrum.
The vertebrae in different regions of the spinal column differ not only in their size but also in their special features. While the vertebral bodies gradually become larger from superior to inferior to accommodate the increasing stresses imposed by the gravity and body weight, the vertebral foramina gradually become smaller to match the decreasing diameter of the spinal cord. The arrangement of the vertebral arches and adjacent processes also varies at different levels in the spine (for details see pp. 111, 113, and 115).
1.5 The Cervical Spine

A Cervical spine, left lateral view
Of the cervical vertebrae, which number seven in all, the first and second cervical vertebrae (C1 and C2, atlas and axis) differ most conspicuously from the common vertebral morphology. They are specialized for bearing the weight of the head and allowing the head to move in all directions, similar to a ball-and-socket joint. Each of the remaining five cervical vertebrae (C3–C7) has a relatively small body, which presents a more or less square shape when viewed from above, and a large, triangular vertebral foramen (see Cc). The superior and inferior surfaces of the vertebral bodies are saddle-shaped, the superior surfaces bearing lateral uncinate processes that do not appear until about the tenth year of life (see p. 126). The transverse process consists of an anterior and a posterior bar, which terminate laterally in two small tubercles (anterior and posterior tubercles). These bars enclose the transverse foramen, through which the vertebral artery ascends from the C6 to the C1 level. The superior surface of the transverse process of the first three cervical vertebrae bears a broad, deep notch (spinal nerve sulcus), in which lies the emerging spinal nerve at that level. The superior and inferior articular processes are broad and flat. Their articular surfaces are flat and are inclined approximately 45° from the horizontal plane. The spinous processes of the third through sixth cervical vertebrae are short and bifid. The spinous process of the seventh cervical vertebra is longer and thicker than the others and is the first of the spinous processes that is distinctly palpable through the skin (vertebra prominens).

B Cervical vertebrae, left lateral view

D Cervical vertebrae, anterior view
1.6 The Thoracic Spine

A Thoracic spine, left lateral view
The thoracic vertebral bodies gradually become taller and broader from superior to inferior, the lower vertebral bodies assuming a transverse oval shape like that of the lumbar vertebrae. The vertebral foramen is roughly circular and is smaller than in the cervical and lumbar vertebrae. The end plates are rounded and triangular. The spinous processes are long and angled sharply inferiorly, creating an overlapping arrangement that interlinks the thoracic vertebrae. The facets of the inferior articular processes are directed anteriorly, while the facets of the superior articular processes face posterior so that they can articulate with the inferior facets to form the zygapophyseal or facet joints (p. 124). Another feature of the thoracic vertebrae is that their transverse processes are angled backward to allow for articulations with the ribs.

B Thoracic vertebrae, left lateral view
The costal facets are cartilage-covered surfaces that articulate with the corresponding ribs (see p. 139). The bodies of the second through ninth thoracic vertebrae (T1–T9) bear two articular facets on each side — a superior costal facet and an inferior costal facet — such that two adjacent vertebrae combine to articulate with the head of a rib. Thus, a given numbered rib articulates with its own numbered vertebra and the vertebra above. Exceptions to this scheme are ribs 1 and 10 to 12, which articulate only with the vertebral body of the same number. The body of the tenth thoracic vertebra has only superior articular facets, and the tenth rib usually articulates only with the tenth thoracic vertebrae. As mentioned, the transverse processes of the thoracic vertebrae (except for T11 and T12) also bear articular facets for the ribs.

D Thoracic vertebrae, anterior view
1.7 The Lumbar Spine

A Lumbar spine, left lateral view
The bodies of the lumbar vertebrae are large and have a transverse oval shape when viewed from above (see C). The massive vertebral arches enclose an almost triangular vertebral foramen, and they unite posteriorly to form a thick spinous process that is flattened on each side. The “transverse processes” of the lumbar vertebrae correspond phylogenetically to rudimentary ribs (see p. 108). Thus, they are more accurately termed costal processes and are not homologous with the transverse processes of the other vertebrae. The thick costal process is fused with the actual transverse process, which is a small, pointed eminence located at the root of each costal process (accessory process, see Cb). The relatively massive superior and inferior articular processes bear slightly inclined articular facets that have a vertical, almost sagittal orientation. The articular facets of the superior articular processes are slightly concave and face medially, while those of the inferior articular processes are slightly convex and face laterally. The mammillary processes on the lateral surfaces of the superior articular processes serve as origins and insertions for the intrinsic back muscles (see Bb and Ca).

B Lumbar vertebrae, left lateral view

D Lumbar vertebrae, anterior view
1.8 The Sacrum and Coccyx

A Sacrum and coccyx, anterior (pelvic) view
The sacrum at birth is composed of five separate sacral vertebrae. Postnatally, they fuse to form a single bone that is flattened anteroposteriorly and has a triangular shape when viewed from the front. The base of the sacrum, located at the superior end of the bone, articulates with the body of the fifth lumbar vertebra by a wedge-shaped intervertebral disk. The apex of the sacrum is at the inferior end of the bone and articulates with the coccyx. The anterior or pelvic surface of the sacrum is concave in both the sagittal and transverse planes (see C). Between the anterior sacral foramina are four transverse ridges (transverse lines), which mark the sites of fusion between the five sacral vertebrae. The coccyx consists of three or four rudimentary vertebrae. Only the first coccygeal vertebra exhibits some of the typical structural elements of a fully formed vertebra. A cartilaginous disk usually connects the base of the coccyx to the apex of the sacrum (sacrococcygeal joint). This joint allows passive forward and backward motion of the coccyx, increasing the anteroposterior diameter of the pelvic outlet during childbirth (see p. 141).

B Sacrum and coccyx, posterior view
The fused spinous processes form a jagged bony ridge on the convex dorsal surface of the sacrum, the median sacral crest. This ridge is flanked on each side by the paired medial sacral crests, formed by fusion of the articular processes. The medial crests are continuous below with the rudimentary inferior articular processes of the fifth sacral vertebra (the sacral cornua); they are continuous above with the two superior articular processes that face posteriorly. Between the sacral cornua is an aperture, the sacral hiatus, which is formed by the incomplete vertebral arch of the fifth sacral vertebrae and provides access to the sacral canal (e.g., for anesthesia). Lateral to the posterior sacral foramina are another pair of longitudinal ridges, the lateral sacral crests, formed by the fused transverse processes. The bony union of the transverse processes with the rudimentary ribs forms the thick lateral parts of the sacral wing that flank the body of the sacrum. Each lateral part bears an ear-shaped (“auricular”) surface that articulates with the ilium (see C). In this view, the two small coccygeal cornua representing the superior articular processes and the two rudimentary transverse processes of the coccyx are visible.

D Sacrum, superior view

E Transverse section through the sacrum
Superior view (the level of the section is shown in A). Fusion of the upper four sacral vertebrae creates four T-shaped bony canals at the level of the intervertebral foramina on each side, providing sites of emergence for the first through fourth sacral nerves. The corresponding ventral and dorsal rami of the spinal nerves exit the bony canals through the anterior and posterior sacral foramina (see p. 543).
1.9 The Intervertebral Disk: Structure and Function

A Structure of an intervertebral disk
Isolated lumbar intervertebral disk, anterosuperior view.
The intervertebral disk consists of an external fibrous ring, the anulus fibrosus, and a gelatinous core, the nucleus pulposus. The anulus fibrosus consists of an outer zone and an inner zone. The outer zone is a fibrous sheath that possesses high tensile strength and is made up of concentric laminae of type I collagen fibers. Its fiber systems crisscross due to their varying obliquity and interconnect the marginal ridges of two adjacent vertebrae (see B), to which they are attached. At the junction with the inner zone of the anulus fibrosus, the tough fibrous tissue of the outer zone blends with a fibrocartilaginous tissue whose type II collagen fibers are attached to the hyaline cartilage end plates of the vertebral bodies (see Da and Ea).

B Outer zone of the anulus fibrosus
Intervertebral disk between the third and fourth lumbar vertebrae, anterior view.
The connective-tissue fiber bundles in the outer zone of the anulus fibrosus cross one another at various angles, interconnecting the bony marginal ridges of two adjacent vertebral bodies.

C Main structural components of an intervertebral disk
Fourth lumbar vertebra with its associated upper disk, superior view.
a Intervertebral disk with the anulus fibrosus and nucleus pulposus.
b Anulus fibrosus (with the nucleus pulposus removed).
c Outer zone of the anulus fibrosus (with the inner zone removed).
d Hyaline cartilage end plate within the bony marginal ridge (entire intervertebral disk removed).
D Position of the intervertebral disk in the motion segment
a Hyaline cartilage end plate, anterosuperior view (the anterior half of the disk and right half of the end plate have been removed).
b Sagittal section through a motion segment (see p. 126), left lateral view.
c Detail from b.
Except for the outer zone of the anulus fibrosus, the entire disk is in contact superiorly and inferiorly with the hyaline cartilage layer of the adjacent vertebral endplates. The subchondral, bony portion of the end plate consists of compact bone (intervertebral surface) permeated by myriad pores (see c) through which the vessels in the bone marrow spaces of the vertebral bodies can supply nutrients to the disk tissue.

E Load-dependent fluid shifts in the intervertebral disk
a The nucleus pulposus functions as a “water cushion” to absorb transient axial loads on the intervertebral disk. Mechanically, the disk represents a hydrostatic system that is resilient under pressure. It is composed of a tension-resistant sheath (the anulus fibrosus) and a watery, incompressible core, the nucleus pulposus. This core consists of 80 to 85% water, which it can reversibly bind in its paucicellular, gelatinous, mucoviscous tissue (owing to its high content of glycosaminoglycans). The nucleus pulposus is under a very high hydrostatic pressure, particularly when acted upon by gravity and other forces. This pressure can be absorbed by the adjacent cartilaginous end plates and also by the anulus fibrosus (which transforms compressive forces into tensile forces). In this way the nucleus pulposus functions as a “water cushion” or hydraulic press between two adjacent vertebral bodies. Combined with the anulus fibrosus, it acts as an effective shock absorber that can distribute pressures uniformly over the adjacent vertebral end plates.
b Fluid outflow from the intervertebral disk (green arrows) in response to a sustained pressure load (thick red arrows). While transient loads are cushioned by the shock-absorber function of the nucleus pulposus and anulus fibrosus (see a), sustained loads cause a gradual but permanent outflow of fluid from the disk. The turgor and height of the disk are reduced, while the end plates and eventually the bony vertebral elements move closer together (see p. 133 for further details on disk degeneration).
c Fluid uptake by the intervertebral disk (green arrows) when pressure is released (thin red arrows). The process described in b is reversed when the pressure on the disk is released, and the height of the disk increases. This increase is caused by fluid uptake from the subchondral blood vessels in the bone marrow spaces, which are instrumental in disk nutrition (see Dc). As a result of pressure-dependent fluid shifts (convection) in the intervertebral disks, the overall body height decreases temporarily by approximately 1% (1.5–2.0 cm) relative to the initial body height during the course of the day.
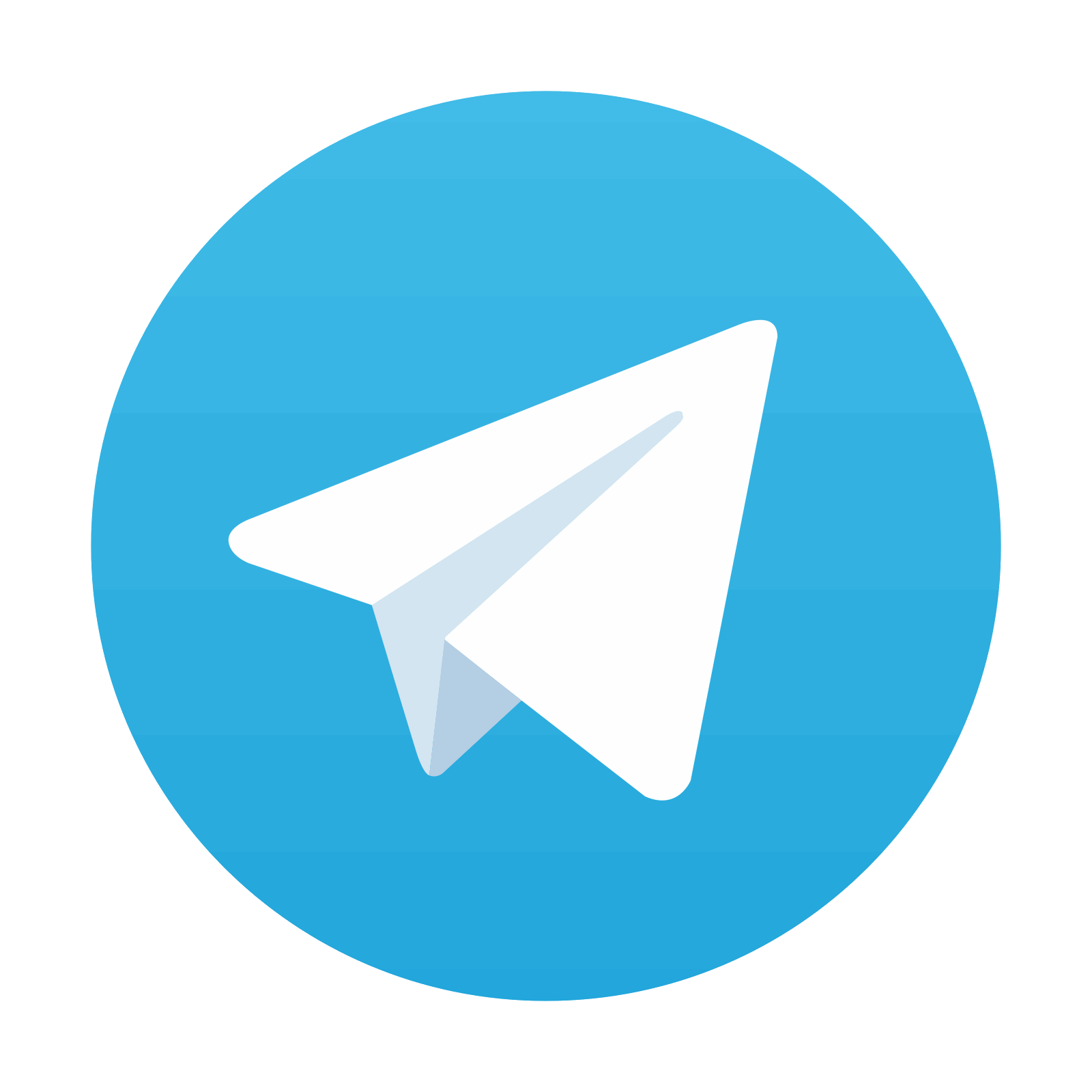
Stay updated, free articles. Join our Telegram channel

Full access? Get Clinical Tree
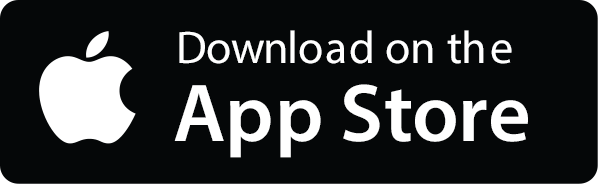
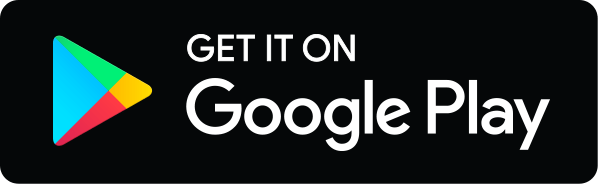