Chapter 2 Blood and related physiology
Blood is circulated around the body within the cardiovascular system, transporting O2, necessary metabolic substrates and hormones to the cells of the body, while removing CO2 and waste products. Plasma, the liquid phase of blood, has many functions, involving colloid osmotic effects, transport, signalling, immunity and clotting. The cellular elements of blood are responsible for gas transport, immunity and some aspects of haemostasis.
2.1 Blood volume and constituents
Blood volume averages about 70 ml kg−1 body weight, giving a total of approximately 5 L in an adult. This consists of a suspension of red cells, white cells and platelets (the formed elements) in plasma. Centrifuging a sample of blood separates the formed elements from the plasma and the ratio of the volume of the packed red cells to the total blood volume is referred to as the haematocrit or packed cell volume (Fig. 22), which is normally about 0.45, or 45%. A thin layer of white cells and platelets can also be identified at the interface between the red cells and the plasma (the buffy coat). Plasma comprises 55% of total blood volume, i.e. about 3 litres in an adult.
2.2 Plasma constituents
Plasma has the same ionic composition as the rest of the extracellular fluid (Section 5.1) but also contains plasma proteins (total concentration of about 60 g L−1), with a range of important functions.
2.3 Erythrocytes
At the end of this section you should be able to:
Erythrocyte development
Stages in erythrocyte development
Pluripotential, or uncommitted, stem cells, which have the potential to produce any type of blood cell, divide and develop into erythroid stem cells committed to form erythrocytes (Fig. 23). These divide further and mature, synthesizing haemoglobin and eventually forming normoblasts. Nuclear material is extruded and the endoplasmic reticulum resorbed, producing first a reticulocyte, containing a few remnants of endoplasmic reticulum, and then an erythrocyte. Normally only these last two cell types are found in the circulation, with reticulocytes making up less than 2% of the total. This percentage rises during periods of rapid erythrocyte synthesis, when more immature cells enter the circulation. Mature red cells take the form of biconcave discs which deform easily within the narrow capillaries. The normal red cell count in blood is 4 × 1012 to 6 × 1012 L−1 (4–6 × 106 mm−3).
Erythrocyte destruction
Ageing erythrocytes are destroyed, often in the spleen, after an average life span of 120 days. The phagocytic cells of the reticuloendothelial system degrade the haemoglobin released, with iron from the haem and amino acids from the globin molecules being recycled. The porphyrin ring is converted to bilirubin, which is further metabolized by the liver and then excreted in bile (Section 6.3).
Control of erythrocyte production
Erythropoiesis is controlled by the kidney, which releases a hormone known as erythropoietin if the delivery of O2 to renal cells falls below normal. This will occur if the concentration of circulating haemoglobin is reduced, i.e., during anaemia. The bone marrow responds by increasing red cell production, thus increasing the haemoglobin content back to normal. Since this control loop is sensitive to tissue O2 levels rather than the actual haemoglobin concentration, other conditions which reduce the O2 content of blood will also stimulate erythropoiesis, even if the haemoglobin concentration is normal. This is seen at high altitudes, where the partial pressures of O2 in the lungs and blood are reduced (Section 4.6). Over a period of weeks at high altitudes, erythropoietin stimulates an increase in the haemoglobin concentration, with a rise in haematocrit and red cell count (compensatory polycythaemia). It is for this reason that athletes wishing to increase the O2-carrying capacity of their blood often train at altitude.
Nutritional requirements for red cell production
Erythropoiesis and haemoglobin synthesis require adequate supplies of the vitamins B12 (cyanocobalamin) and folic acid, as well as the mineral iron. Deficiencies of these may cause anaemia.
Vitamin B12 and folate
Deficiency of B12 or folate can arise in two ways. The diet itself may include inadequate amounts of the normal source foods for these vitamins, e.g., animal products (for both B12 and folate) and green vegetables (rich in folate). Vitamin B12 deficiency can also occur with a normal diet as a result of reduced B12 absorption. Parietal cells in the stomach normally secrete intrinsic factor, which binds to B12, and it is the resulting complex that is absorbed from the ileum (Section 6.4). The B12 is then transported to the liver, which normally stores 1–2 years’ supply (liver is an excellent dietary source of B12). In pernicious anaemia, there is reduced secretion of intrinsic factor. This leads to malabsorption of B12 and a megalo-blastic, macrocytic anaemia results. These patients often also appear mildly jaundiced because of increased bilirubin production following haemo-lysis of the abnormally fragile red cells. Treatment involves regular intramuscular injections with B12, thereby bypassing the normal intestinal absorption mechanisms.
Iron
Iron is mainly absorbed in the ferrous form by means of intestinal transferrin and an iron–transferrin receptor (Section 6.4). The rate of absorption increases when iron demand is increased within the body. Plasma transferrin transports iron within the circulation and excess iron is stored as ferritin or haemosiderin, particularly in the liver, spleen and bone marrow. Mean iron stores are 2–3 times larger in men than women, presumably as a consequence of menstrual losses.
Blood groups and transfusion
ABO system
There are two main antigens in this system, A and B, and these give rise to four different blood groups as shown in Table 2. Of these, blood groups O and A are almost equally common and together account for over 85% of the population in Western Europe. It should be noted that plasma always contains preformed antibodies (IgM class) against A or B antigens which are not already present on our own erythrocytes, whether we have been sensitized by exposure to foreign red cells or not. This breaks the general rule in immune responses, since antibodies against all other foreign antigens are only secreted in appreciable amounts after exposure to that antigen (Section 2.5). It may be that we are all exposed to A and B antigens from another source, e.g., intestinal bacteria, and only become tolerant to the antigens also present on our erythrocytes. Whatever the mechanism, the consequence is that a major immune reaction can be expected on the first exposure to blood of the wrong ABO group.
2.4 Leucocytes
At the end of this section you should be able to:
Leucocyte types
Based on their histological appearances, five main types of leucocyte may be identified. These fall into two morphological groups. Polymorphonuclear granulocytes have irregular, multilobed nuclei and a high density of cytoplasmic granules (Fig. 23). Neutrophils, eosinophils and basophils all belong in this group. Lymphocytes and monocytes, by comparison, lack granules and have large, regular nuclei, and so they are classified as mononuclear agranulocytes. The basic characteristics of individual leucocyte types are listed below.
Neutrophils comprise 60–70% of circulating leucocytes. They are highly mobile and can engulf debris or foreign organisms through the process of phagocytosis, trapping the target in a vesicle which then fuses with a lysosome (Fig. 24). Organic material is digested by lysosomal enzymes, although inorganic material may remain within the cytoplasm indefinitely.
Lymphocytes are the only nonphagocytic white cells and represent 25–30% of blood leucocytes. They are central to specific immune defences within the body (Section 2.5) and can be subdivided into B and T lymphocytes.
Leucocyte production
Leucocytes originate from the pluripotential stem cells in the bone marrow, which divide and mature giving two separate leucocyte stem cell lines (Fig. 23).
The lymphoid line. From this stem cell line the lymphocytes are produced (Fig. 23). B cells mature in the marrow before being distributed to the lymphoid tissues of the body, i.e. the lymph nodes, spleen, thymus and Peyer’s patches in the intestinal submucosa. T lymphocyte precursor cells are believed to migrate initially to the thymus, where they mature fully before being redistributed to other lymphoid sites. Lymphocytes can replicate and develop further within the lymphoid tissues of the body and there is a continuous recirculation of lymphocytes from blood to lymph and back again.
2.5 Immune responses
At the end of this section you should be able to:
Nonspecific or innate immunity
The inflammatory response and phagocytosis
Phagocytic responses in inflammation Tissue macrophages adjacent to a site of bacterial invasion, for example, become mobile and phagocytically active. Chemicals released from injured and infected cells (chemotaxins) act as attractants for these cells, directing their movements towards the damaged area in a process called chemotaxis. Local macrophages are soon reinforced by the migration of blood neutrophils and monocytes into the region (Fig. 25). These stick to the endothelium of capillaries in the affected area (leucocyte margination) and then invaginate themselves through the clefts between the endothelial cells using active amoeboid movements (diapedesis). The blood-borne phagocytes escape into the tissues where they are chemotactically attracted to assist in removal of infectious or toxic agents and tissue debris. The resulting congregation of large numbers of neutrophils and macrophages within a tissue is the histological hallmark of acute inflammation.
Body temperature.This may rise as a result of resetting of the hypothalamic set point (Section 1.1). Fever (pyrexia) seems to increase the activity of phagocytic cells and is promoted both by exogenous pyrogens, including bacterial products known as endotoxins, and by endogenous pyrogens released by phagocytes. Endogenous pyrogens stimulate prostaglandin synthesis and this is blocked by drugs such as aspirin. This probably explains their ability to reduce fever (their antipyretic action).
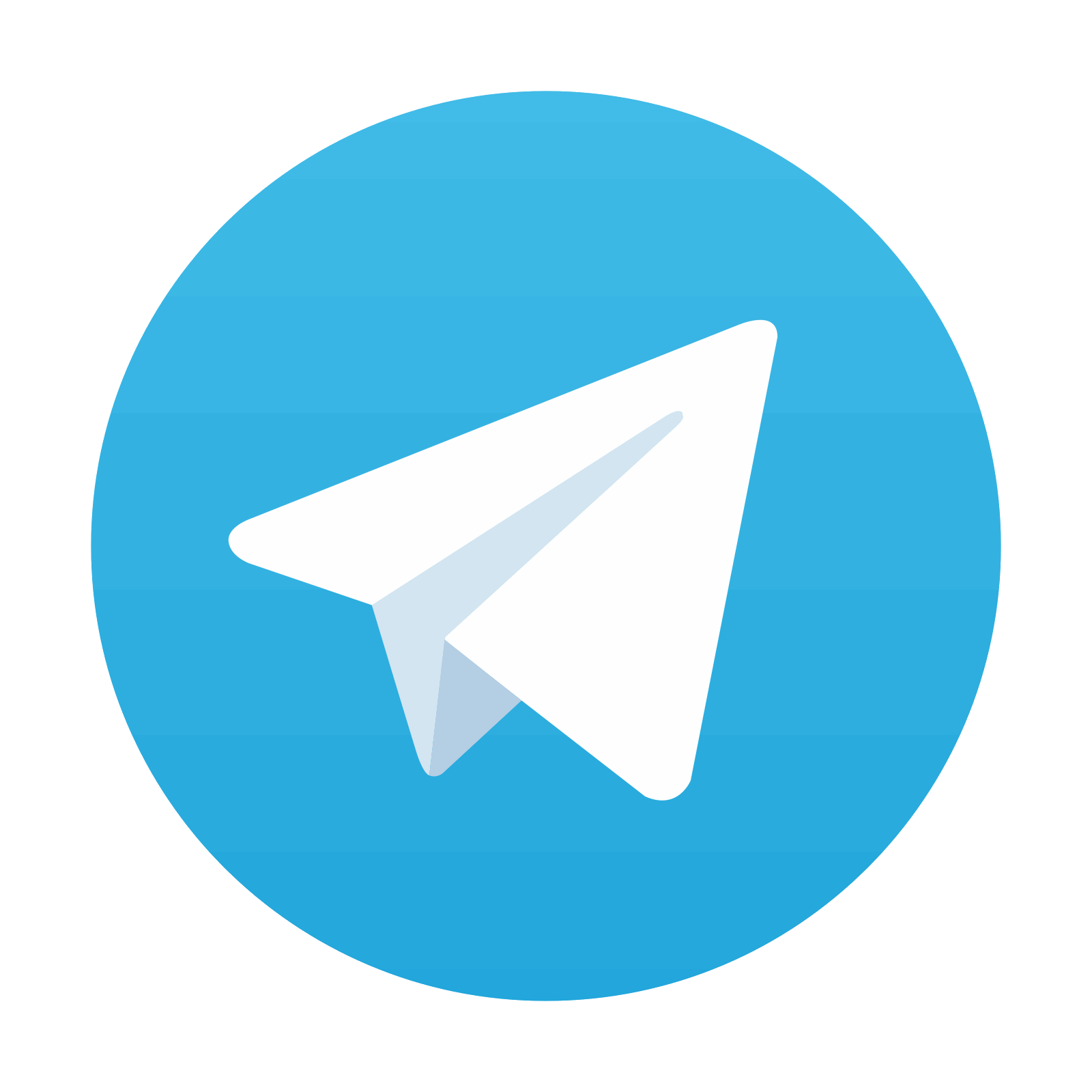
Stay updated, free articles. Join our Telegram channel

Full access? Get Clinical Tree
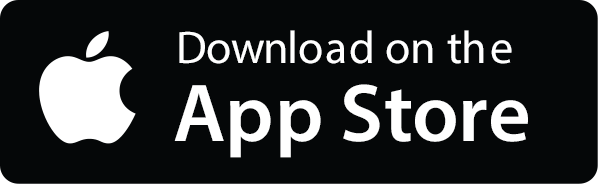
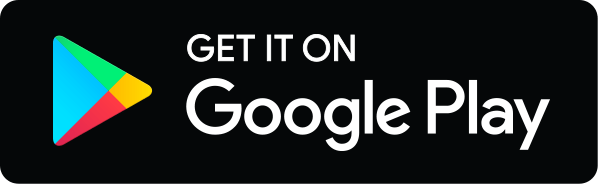