(1)
Laboratory of Biophysical Chemistry and Groningen Biomolecular Science and Biotechnology Institute, University of Groningen, Groningen, The Netherlands
Abstract
Biosynthetic incorporation of Trp analogs in a protein can help in its characterization using fluorescence spectroscopy and other methodologies like NMR and phosphorescence. Here a protocol is presented resulting in the efficient incorporation of Trp analogs in a recombinant protein, using an Escherichia coli Trp auxotroph. An overview of recent developments in the Trp analog incorporation field is also presented.
Key words
Trp auxotrophFluorescenceAlloprotein1 Introduction
Replacing Trp in a protein by a Trp analog can be advantageous in its characterization using various spectroscopy techniques including fluorescence spectroscopy. Trp is a popular fluorescence probe in protein science because it is an intrinsic probe and its quantum yield (Q) and emission energy are very sensitive for changes in its microenvironment [1]. Under certain experimental conditions, one cannot exploit these advantages of Trp photophysics, for example, when there are other Trp-containing proteins in the sample or when the absorbance of sample components, like DNA at high concentration, overlaps with Trp absorbance. This can result in a strong inner filter effect, limiting efficient excitation of Trp. Replacing Trp by a Trp analog absorbing at higher wavelengths may help in overcoming this. The absorbance and fluorescence characteristics of Trp can be engineered via a subtle modification in the indole moiety, and many Trp analogs can be efficiently biosynthetically incorporated. Escherichia coli is the most popular expression host for the incorporation of Trp analogs in recombinant proteins, and the following Trp analogs with an indole moiety are translated: 4-, 5-, and 6-fluoroTrp [2–4]; 2-azaTrp [5], 4-azaTrp [6], 5-azaTrp [6], 6-azaTrp [7], and 7-azaTrp [5]; 4- and 5-hydroxyTrp [8, 9]; and 4- and 5-aminoTrp [10] (Fig. 1). The absorbance spectra of Trp and three popular Trp analogs, 7-azaTrp, 5-hydroxyTrp, and 5-fluoroTrp, are shown in Fig. 2.
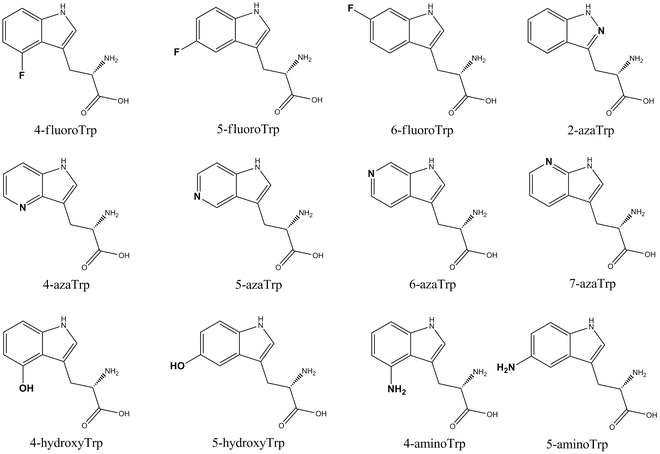
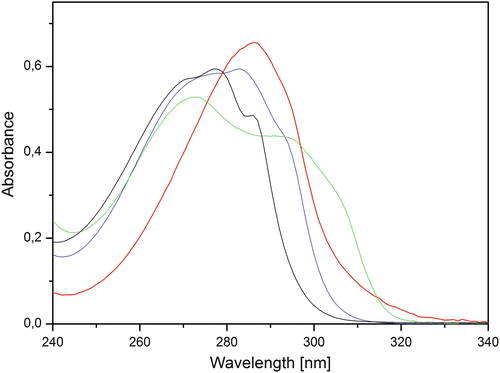
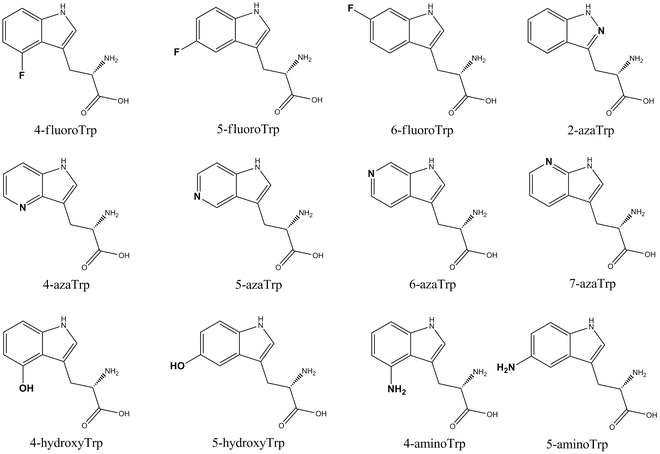
Fig. 1
Structures of Trp analogs biosynthetically incorporated in proteins using an Escherichia coli Trp auxotroph
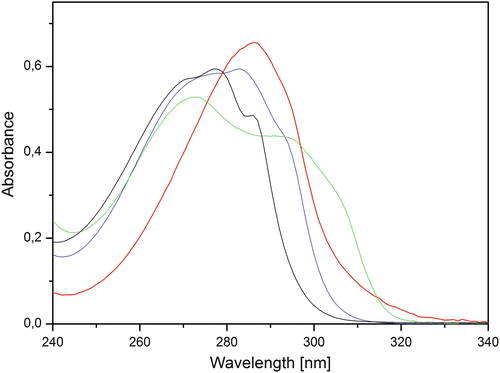
Fig. 2
Absorbance spectra of equimolar concentrations of Trp (black), 7-azaTrp (red), 5-hydroxyTrp (green), and 5-fluoroTrp (blue) in neutral buffer
For the efficient replacement of Trp in the recombinant protein by a Trp analog, a Trp auxotroph expression host is needed and the gene of the target protein should be cloned behind an inducible non-leaky promotor. Most groups use the so-called “two-step” incorporation protocol. In brief, during the first step the auxotroph, harboring the plasmid containing the gene of the target protein, is grown in a synthetic medium, like M9 medium, supplemented with Trp. Cells are pelleted at mid-exponential phase and washed several times to remove Trp. Culturing of the cells is continued in the same medium without Trp for ~30 min to reach full Trp depletion. Trp analog is added and after ~15 min, allowing uptake of the analog by the cells, expression of the target protein is induced and incubation continued during a period optimal for the expression of the target protein.
In this chapter, a “two-step” protocol is presented in Subheadings 2 and 3. Below a brief description of those analogs of interest for fluorescence spectroscopy is presented; a more comprehensive description of these analogs can be found in several excellent reviews [11–14] and in the cited references. An overview of recent developments in the Trp analog incorporation field, not included in the reviews mentioned above, is also presented.
1.1 Trp Analogs Translated by E. coli
1.1.1 Fluorotryptophan
FluoroTrp analogs have been biosynthetically incorporated in many proteins, mainly because of their application as intrinsic probe in 19F NMR experiments. Replacing Trp by fluoroTrp can also be attractive when the protein is analyzed using fluorescence spectroscopy. 4-FluoroTrp is not fluorescent at ambient temperatures [4]. Replacing Trp by this analog in a protein results in a fluorescently silent protein, which can be an advantage if a Trp residue in another protein, also present in the sample, is the target of the investigation.
The absorbance spectrum of 5-fluoroTrp is 7 nm red shifted compared to Trp (Fig. 2). 5-FluoroTrp shows a ~50 % higher Q than Trp [15] and this has been related to the increase of the ionization potential of indole upon 5-fluoro substitution [16]. The higher ionization potential suppresses electron transfer of the excited 5-fluoroTrp to a nearby peptide bond, acting as electron acceptor. This electron transfer process is an efficient quenching route of Trp in a protein, and its rate can vary between ground-state subpopulations of the protein, resulting in different Trp lifetimes. Efficient suppression of this electron transfer process in 5-fluoroTrp explains its higher Q and the homogeneous fluorescence decay, which is often monoexponential, in particular if the 5-fluoroTrp is at a buried position and features a blue emission [17–20]. The homogeneous decay of 5-fluoroTrp makes it an attractive donor in time-resolved FRET experiments. The high sensitivity of the Q of Trp for a change in microenvironment is mainly due to changes in the efficiency of the electron transfer to the peptide bond [1]. Therefore, the Q of 5-fluoroTrp embedded in a protein is less sensitive for a change in microenvironment than Trp at that position [16].
The ionization potential of 6-fluoroTrp is in between that of Trp and 5-fluoroTrp, and its decay was found more homogeneous than for Trp, but less than when it is replaced by 5-fluoroTrp [16].
1.1.2 7-AzaTrp
Replacing Trp by 7-azaTrp in a protein introduces two attractive properties: (1) its absorbance is red shifted compared to Trp, allowing its specific excitation at 310–315 nm where Trp does not absorb (Fig. 2), and (2) the Q is very sensitive for changes in the microenvironment. Larger than tenfold changes in Q for a 7-azaTrp containing protein have been observed [11]. Compared to Trp, the emission spectrum is also 30–60 nm red shifted. Free 7-azaTrp is almost nonfluorescent (Q = 0.01–0.02), while free Trp shows a Q of 0.14. The large variation in Q makes 7-azaTrp an attractive reporter probe in titration experiments. For monitoring conformational changes during protein (un)folding, including conformations where the 7-azaTrp is fully solvent exposed, loss of the emission signal limits its use as reporter group. 4-AzaTrp and 5-azaTrp have been proposed as alternatives to overcome this limitation, as they show a modest Q when free in solution [6]. These analogs can also be selectively excited >310 nm and show larger Stokes shifts than 7-azaTrp. Only few proteins have been labeled with these analogs, and the reported data indicate the λ em max and Q of 4-azaTrp and 5-azaTrp are not very sensitive for changes in microenvironment.
1.1.3 5-HydroxyTrp
Like 7-azaTrp, the absorbance spectrum of 5-hydroxyTrp allows its selective excitation at 315 nm (Fig. 2). The Q of free 5-hydroxyTrp, ~0.25, is relatively high for a Trp analog. 5-HydroxyTrp is one of the few Trp analogs known to emit from the 1Lb state. Only a small change in permanent dipole is induced when 5-hydroxyTrp is excited from ground to the 1Lb state, and this explains why the emission energy of 5-hydroxyTrp is not very sensitive for changes in microenvironment. The red shoulder of 5-hydroxyTrp is due to 1Lb absorption, and red-edge excitation gives a high intrinsic anisotropy because no depolarization from 1La to 1Lb takes place. This makes 5-hydroxyTrp an attractive probe for anisotropy studies [8, 9].
1.2 Recent Developments in the Trp Analog Incorporation Field
1.2.1 E. coli Trp Auxotroph Expression Hosts
Most Trp analog-containing proteins reported in the literature have been produced using an E. coli Trp auxotroph. We often use the M5219 Trp auxotroph, a strain engineered with the cI857 repressor gene in its chromosome. This makes it an attractive strain if the gene of the target protein is cloned behind the heat-inducible λPR or λPL promoters [21].
Employing a Trp auxotrophic strain results in the global replacement of all Trp positions in the target protein by a Trp analog. When site-specific information is needed of a multi-Trp-containing protein via Trp analog spectroscopy, first a single Trp construct needs to be created, and replacing many Trp positions can compromise structure and function of the protein. In such a case, the amber codon suppression technology [22] is attractive as it has the potential to label a multi-Trp-containing protein site selectively with a Trp analog. In this approach, the expression host is outfitted with an orthogonal tRNA/aminoacyl-tRNA synthetase pair, able to label a protein with a Trp analog in response to an engineered stop codon in the gene of the target protein.
One example is the orthogonal yeast tRNA/ aminoacyl-tRNA synthetase pair reported by Kwon and Tirrell [23]. A single mutation, T415G, was introduced in phenylalanyl-tRNA synthetase making it able to aminoacylate the tRNA with 6-chloroTrp, 6-bromoTrp, or benzothienylalanine. This orthogonal pair was introduced in a Phe/Trp double auxotrophic E. coli strain, and the three analogs could be incorporated in a protein with high fidelity in response to an amber codon in the gene of the target protein. The engineered synthetase also recognizes Trp and Phe, and to prevent incorporation of Trp at the position corresponding with the amber codon, the synthetic medium was supplemented during expression of the target protein with a very low concentration of Phe and Trp and a high concentration of Trp analog. In this way, the endogenous E. coli translation system, showing a higher affinity for Trp than the engineered synthetase, translated the Trp codon positions in the target protein, while the position corresponding to the amber codon was labeled for >98 % with Trp analog. Unfortunately, the three analogs used in this study are not or minimally fluorescent. Because attractive fluorescent Trp analogs like 7-azaTrp and 5-hydroxyTrp are translated by E. coli, this system cannot be used to label a protein, in response to an engineered amber codon, with one of these analogs.
1.2.2 Other Trp Auxotroph Expression Hosts
Besides E. coli, several other expression systems were recently reported for the incorporation of Trp analogs.
The gram-positive bacterium Lactococcus lactis is known as an attractive expression host because many proteins, including membrane proteins, can be functionally expressed at high levels [24]. The organism grows under aerobic and anaerobic conditions, shows a relative low proteolytic activity, and a tightly regulated nisin-inducible promoter system [25] is available. A L. lactis Trp auxotroph was created by El Khattabi et al. via deletion of the Trp synthetase gene trpBA [26]. A single Trp-containing target protein, cloned behind the nisin-inducible promoter PnisA, was expressed in the presence of Trp analogs using this Trp auxotroph. 7-AzaTrp and 5-fluoroTrp could be efficiently incorporated (>97 %) and also 5-hydroxyTrp, although its incorporation yield (89 %) is somewhat lower than typically found for an E. coli Trp auxotroph system. Interestingly, this system can also efficiently incorporate 5-methylTrp (92 %), an analog not translated by E. coli. This latter result suggests that the substrate specificity of L. lactis tryptophanyl-tRNA synthetase is more relaxed than E. coli tryptophanyl-tRNA synthetase. The number of Trp analogs translated by L. lactis could be increased when the L. lactis tryptophanyl-tRNA synthetase was coexpressed together with the target protein [27]. Chloro and bromo atoms containing Trp analogs and also 5,6 difluoroTrp can now be efficiently incorporated (>89 %) (Fig. 3). Coexpression of the L. lactis tryptophanyl-tRNA synthetase also increased the incorporation efficiency of 5-hydroxyTrp and 5-methylTrp to 95 %. This new expression system features the most relaxed specificity for Trp analog structures reported to date and gives a high alloprotein yield.


Fig. 3
Structures of Trp analogs biosynthetically incorporated in a protein by a Lactococcus lactis Trp auxotroph, coexpressing the L. lactis tryptophanyl-tRNA synthetase enzyme [27]
Zhang et al. reported the development of an orthogonal tryptophanyl-tRNA/ tryptophanyl-tRNA synthetase (TrpRS) pair for use in mammalian 293 T cells and able to incorporate 5-hydroxyTrp in response to an opal (TGA) codon in a target protein [28]. A high fidelity was reported for this orthogonal pair, based on tryptophanyl-tRNA and TrpRS of Bacillus subtilis. One mutation, V144P, was needed to alter its specificity from Trp to 5-hydroxyTrp. The high fidelity of this system has recently been questioned by Antonczak et al. [29]. The developed TrpRS protein by Zhang et al. was characterized in more detail using a complementation assay, X-ray crystallography, molecular modeling, and calorimetry. Their analysis indicates this TrpRS prefers Trp over 5-hydroxyTrp as substrate, similar to wt TrpRS from Bacillus subtilis. Labeling a Trp-containing protein with 5-hydroxyTrp in response to an opal codon position is therefore not possible [29].
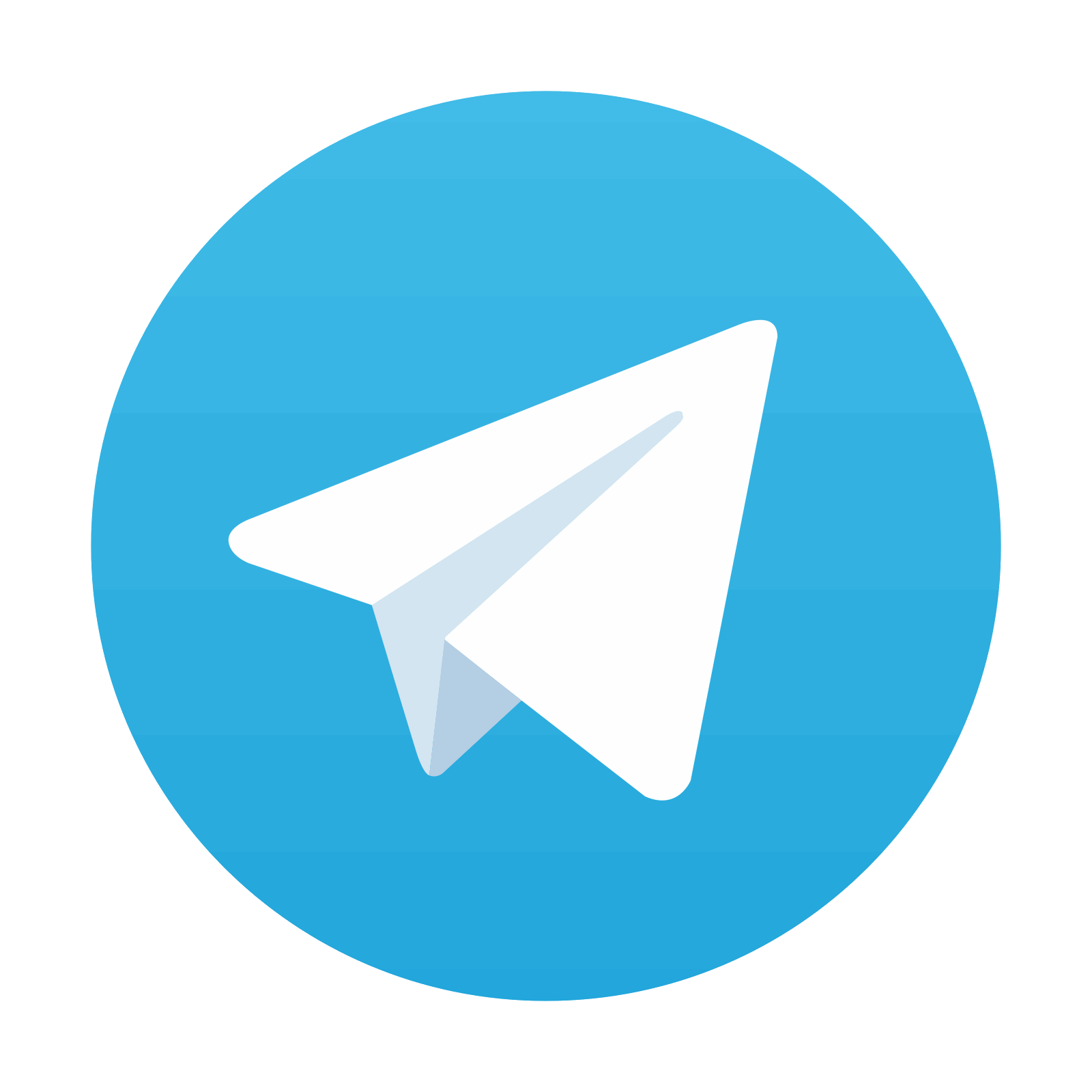
Stay updated, free articles. Join our Telegram channel

Full access? Get Clinical Tree
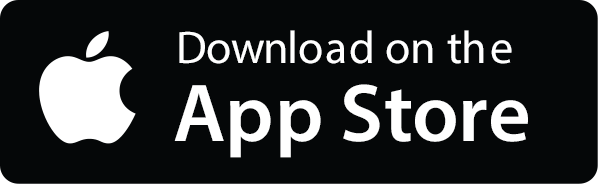
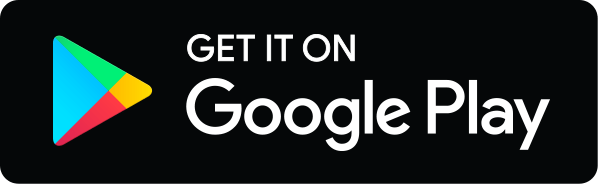
