Chapter 12 Biopharmaceuticals
Introduction
The term ‘biopharmaceutical’ was originally coined to define therapeutic proteins produced by genetic engineering, rather than by extraction from normal biological sources. Its meaning has broadened with time, and the term now encompasses nucleic acids as well as proteins, vaccines as well as therapeutic agents, and even cell-based therapies. In this chapter we describe the nature of biopharmaceuticals, and the similarities and differences in discovery and development between biopharmaceuticals and conventional small-molecule therapeutic agents. The usual starting point for biopharmaceuticals is a naturally occurring peptide, protein or nucleic acid. The ‘target’ is thus identified at the outset, and the process of target identification and validation, which is a major and often difficult step in the discovery of conventional therapeutics (see Chapter 6), is much less of an issue for biopharmaceuticals. Equally, the process of lead finding and optimization (Chapters 7, 8, 9) is generally unnecessary, or at least streamlined, because nature has already done the job. Even if it is desirable to alter the properties of the naturally occurring biomolecule, the chemical options will be much more limited than they are for purely synthetic compounds. In general, then, biopharmaceuticals require less investment in discovery technologies than do conventional drugs. Toxicity associated with reactive metabolites – a common cause of development failure with synthetic compounds – is uncommon with biopharmaceuticals. On the other hand, they generally require greater investment in two main areas, namely production methods and formulation. Production methods rely on harnessing biological systems to do the work of synthesis, and the problems of yield, consistency and quality control are more complex than they are for organic synthesis. Formulation problems arise commonly because biomolecules tend to be large and unstable, and considerable ingenuity is often needed to improve their pharmacokinetic properties, and to target their distribution in the body to where their actions are required.
It is beyond the scope of this book to give more than a brief account of the very diverse and rapidly developing field of biopharmaceuticals. More detail can be found in textbooks (Buckel, 2001; Ho and Gibaldi, 2003; Walsh, 2003). As the field of biopharmaceuticals moves on from being mainly concerned with making key hormones, antibodies and other signalling molecules available as therapeutic agents, efforts – many of them highly ingenious – are being made to produce therapeutic effects in other ways. These include, for example, using antisense nucleic acids, ribozymes or RNAi (see below and Chapter 6) to reduce gene expression, the use of catalytic antibodies to control chemical reactions in specific cells or tissues, and the development of ‘DNA vaccines’. So far, very few of these more complex ‘second-generation’ biopharmaceutical ideas have moved beyond the experimental stage, but there is little doubt that the therapeutic strategies of the future will be based on more sophisticated ways of affecting biological control mechanisms than the simple ‘ligand → target → effect’ pharmacological principle on which most conventional drugs are based.
The early days of protein therapeutics
• Repeated dosage of non-human proteins generated an immune response in some patients against the foreign sequences, which differed by several amino acids from the human sequence. Such immune responses could cause illnesses such as serum sickness, or loss of efficacy of the protein.
• Human tissue was in short supply and was subject to potential contamination with infectious agents. Growth hormone extracted from human cadaver pituitary glands was contaminated with prions that cause Creutzfeld–Jakob disease, a dementing brain-wasting disease similar to bovine spongiform encephalitis (BSE) and sheep scrapie. Human blood plasma-derived products have been tainted with hepatitis B virus and HIV.
• Many agents (e.g. cytokines) cannot be extracted in sufficient quantities to be used therapeutically.
• Batch-to-batch variability was considerable, requiring standardization by bioassay in many cases.
Currently available classes of biopharmaceuticals
The major classes of biopharmaceuticals currently on the market include hormones, cytokines, growth factors, antibodies, enzymes, vaccines and nucleotide-based agents. Examples of therapeutic proteins, including antibodies, enzymes and other proteins approved for clinical use, are presented in Table 12.1. Others not included in the compilation include therapeutic preparations such as serum albumin, haemoglobin and collagen, which are not drugs in the conventional sense.
Growth factors and cytokines
Erythropoietin (EPO), normally secreted by the kidney to stimulate the production of red blood cells, is the most successful biotechnology product so far marketed. EPO boosts red cell counts and reduces transfusion requirements for patients rendered anaemic by cancer chemotherapy or renal disease. Various forms of EPO with clearance profiles – and hence duration of action – modified by linkage with polyethylene glycol (PEGylation) or alteration of its glycosylation (see below and Chapter 17) are also available. Off-label use of EPO by athletes to improve performance has caused controversy.
Therapeutic antibodies
Antibody selection by phage display
Phage display technology (Benhar, 2001) is a useful way to identify antigen combining regions to produce monoclonal antibodies that bind to therapeutically relevant antigens. Bacteriophages (or phages) are viruses that replicate in bacteria, Escherichia coli being the organism of choice in most cases. For antibody selection (Figure 12.1) a large DNA library, encoding millions of different putative antigen-binding domains, is incorporated into phage DNA, so that each phage particle encodes a single antigen combining region. The mixed phage population is added to E. coli cultures, where the phage replicate, each phage particle expressing copies of a single antigen combining region on its surface. The phage suspension is applied to plates coated with the antigen of interest (‘panning’) and those phage particles expressing antigen combining regions recognizing the antigen stick to the plates. The adherent phages are isolated, allowing the antigen combining region-encoding DNA to be identified and inserted into the DNA sequence encoding an appropriate full-size antibody scaffold to produce a specific antibody, or into a reduced size, simplified monomeric framework to produce a single-chain (sFv) antibody.
Uses of antibodies as therapeutic agents
Cancer immunotherapy
Although high-affinity mouse monoclonal antibodies to target antigens can be reproducibly produced in industrial quantities, and bind to specific human targets, they generally function poorly in recruiting human effector functions. The therapeutic potency of these antibodies can be enhanced by taking advantage of the targeting selectivity of antibodies (see Chapter 17) for the purposes of drug delivery. Linking other agents, such as cytotoxic drugs, biological toxins, radioisotopes or enzymes to activate prodrugs to targeting antibodies enhances their delivery to the target cells and reduces side effects by directing the toxic agents to the tumour and minimizing clearance. Bispecific antibodies with one H-L chain pair directed against a target cell antigen and the other against a soluble effector such as a complement component, or against a cell surface marker of an effector cell type, have also been developed to bring the components of the reaction together. A number of these are in clinical trials for a variety of different malignancies, but have in general proved less successful than had been expected on the basis of animal studies.
Antibody use in transplantation and immunomodulation
Even human antibodies face disadvantages as therapeutics. Because of their size (MW ~150 kDa), their ability to penetrate into tissues, such as solid tumours, is limited. Engineered versions lacking the Fc region, which is largely responsible for hypersensitivity responses of patients treated with monoclonal antibodies, have replaced the (Fab)2 fragments generated by proteolysis from the intact antibody. Single sFv chains containing the H and L variable regions are the smallest antibodies containing a high-affinity antigen-combining site. Still in the experimental stage, ‘di-antibodies’ with two H-L units connected by a 15-amino acid linker (Gly4Ser)3 peptide have greatly increased affinity for antigen (see also Chapter 13).
Catalytic antibodies
Antibodies can be used to enhance the chemical reactivity of molecules to which they bind. Such catalytic antibodies (‘abzymes’) created with transition state analogs as immunogens specifically enhance substrate hydrolysis by factors of 102–105 over the rate in their absence, and this principle has been applied to the development of therapeutic agents (Tellier, 2002). Both esterase and amidase activities have been reported. Catalytic turnover of substrates by abzymes is low in comparison to true enzymes, as high-affinity binding impedes the release of products. Attempts to improve catalytic efficiency and to identify therapeutic uses for catalytic antibodies have engrossed both academic and biotech startup laboratories. Targets being approached with these antibodies include cocaine overdose and drug addiction, bacterial endotoxin, and anticancer monoclonal antibody conjugated with a catalytic antibody designed to activate a cytotoxic prodrug. Attempts are also being made to develop proteolytic antibodies containing a catalytic triad analogous to that of serine proteases, designed to cleave gp120 (for treatment of HIV), IgE (for treatment of allergy), or epidermal growth factor receptor (for treatment of cancer).
Vaccines
Using the immune system to protect the body against certain organisms or conditions is a powerful way to provide long-term protection against disease. Unlike with small-molecule pharmaceuticals, which are administered when needed, once immunity is present subsequent exposure to the stimulus automatically activates the response. Most current vaccines are against disease-causing organisms such as bacteria, viruses and parasites. More complex conditions where the antigens are not so well defined are also being addressed by immunization. Vaccines are being tested for cancer, neurodegenerative diseases, contraception, heart disease, autoimmune diseases, and alcohol and drug addiction (Rousseau et al., 2001; Biaggi et al., 2002; BSI Vaccine Immunology Group, 2002; Kantak, 2003). Immune induction is complex. Pioneering experiments with attenuation of disease organisms showed that illness was not required for immunity. The goal in immunization is to retain enough of the disease-causing trait of an antigen to confer protection without causing the disease. For infectious agents, various methods of killing or weakening the organism by drying or exposure to inactivating agents still dominate manufacturing processes. Isolation of antigens from the organisms, or modifying their toxins, can be used in some cases. Vaccine production of isolated antigen ‘subunit’ vaccines benefits from protein engineering.
Novel approaches to presenting antigens are expected to have an impact on vaccination. An example is the phage display technique (Benhar, 2001), described earlier as a technique for antibody selection. The same approach can be used to provide the protein antigen in a display framework that enhances its immunogenicity and reduces the requirement for immune adjuvants (of which there are few approved for human use). Viruses encoding multiple antigens (‘vaccinomes’) can also be employed.
Genetic vaccination (Liu, 2003) employs a DNA plasmid containing the antigen-encoding gene, which is delivered to the host tissue by direct injection of DNA, or by more exotic techniques such as attaching the DNA to microparticles which are shot into tissues at high speed by a ‘gene gun’, or introduced by other transfection methods (Capecchi et al., 2004; Locher et al., 2004; Manoj et al., 2004). When the DNA is transcribed, the mRNA translated and the protein expressed in the host tissue, eukaryotic sequences will undergo appropriate post-translational modification, which does not occur with conventional protein vaccines. In general, partial sequences of pathogen-derived proteins are fully immunogenic, despite lacking the toxicity of full-length transcripts. The first human trial for a genetic vaccine against HIV took place in 1995, and others quickly followed, including hepatitis, influenza, melanoma, malaria, cytomegalovirus, non-Hodgkin’s lymphoma, and breast, prostate and colorectal tumours. Initial results have been promising. At the time of writing, recombinant vaccines against hepatitis A and B (e.g. Twinrix), papillomavirus virus for genital warts and cervical cancer (Gardisil), and against Haemophilus b/meningococcal protein for meningitis (Comvax) are approved. Single or multiple proteins from an organism can be included, and proteins that interfere with the immune response (common in many infectious agents) excluded.
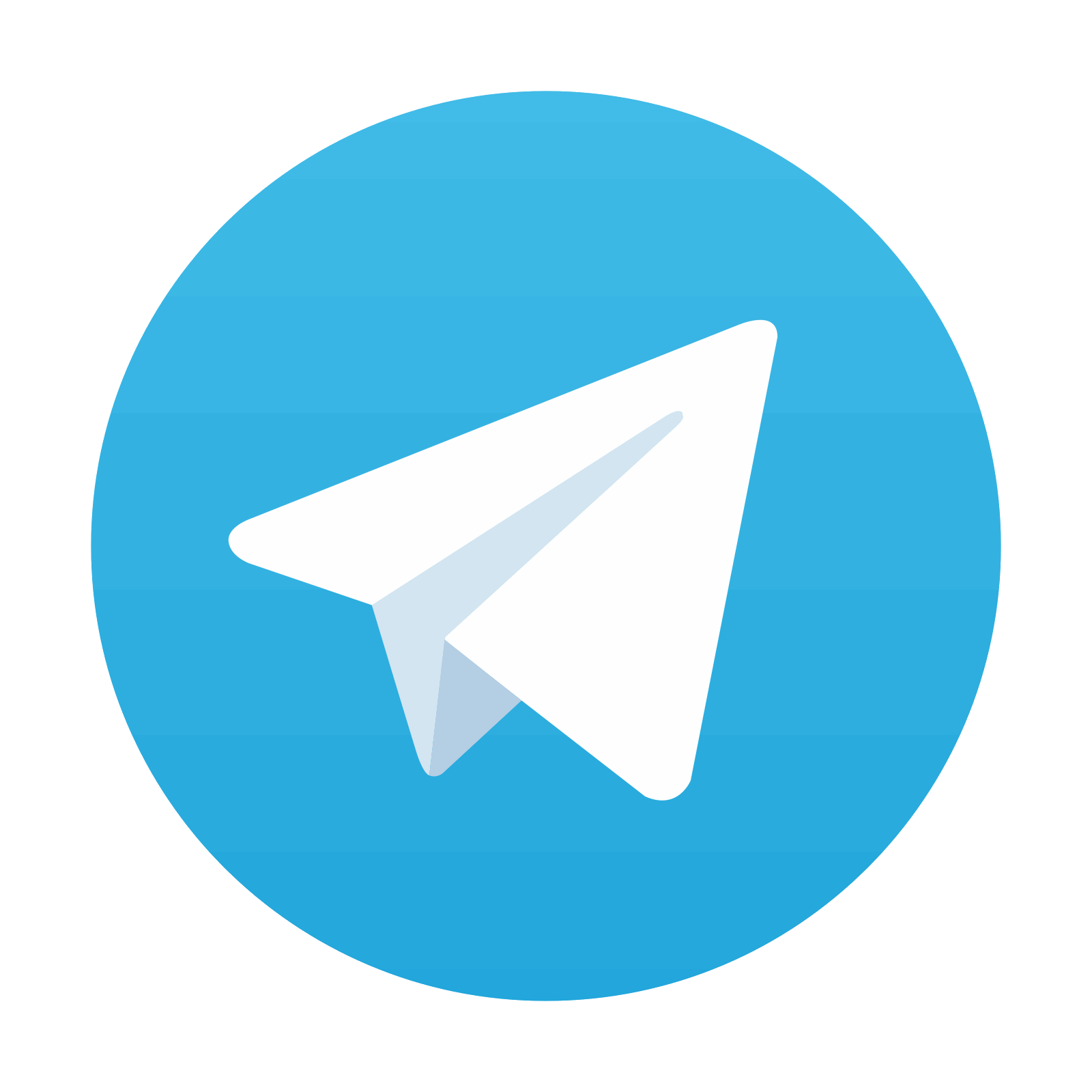
Stay updated, free articles. Join our Telegram channel

Full access? Get Clinical Tree
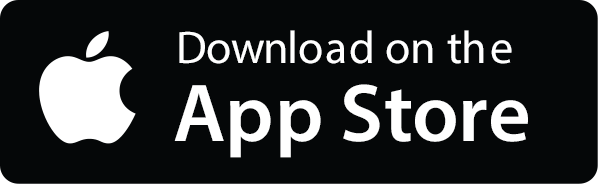
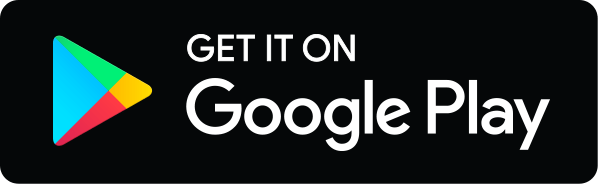