Fig. 22.1
Advancement through different phases in clinical trials
Clinical trial design for oncology studies can broadly be categorized into rule-based and model-based designs. Rule-based designs are often used in early-phase (I/II) cancer trials. A small number of patients start with an initial dose, and the occurrence of unacceptable dose-limiting toxicity (DLT) determines, based on prespecified rules, the dose for the next group of patients. Commonly used designs are the single- and two-stage up-and-down designs and optimal/flexible multiple-stage designs; see Chow and Liu [50]. The use of rule-based designs in early trials is appealing since they allow minimized exposure of patients to doses with unacceptable DLT. These designs can, however, be suboptimal from a statistical standpoint resulting in low efficiency. Model-based designs are usually more efficient. They employ statistical dose-response models to guide the dose-finding process and for estimating the effect (safety or efficacy) at different doses of the new investigational drug.
Clinical Trials for Targeted Therapy
The altered focus from cytotoxic agents to targeted therapy and predictive biomarkers has called for a shift from the classical paradigm in phase I studies where the primary focus is on safety (MTD) and phase II studies with primary focus on efficacy (see Mandrekar et al.) [51]. Within the new targeted therapy paradigm, the focus is on identifying molecularly defined subgroups of patients who will benefit from the targeted therapy and on understanding the drug activity for these patients. Novel designs have been developed, in which predictive biomarkers play a central role. Among these are enrichment, adaptive, umbrella, and basket designs. Brief descriptions of these designs are given below.
Enrichment Designs
Enrichment designs involve diagnostic tests that are used to identify which patients are positive for a biomarker and thereby eligible for the trial (Fig. 22.2). The eligible patients are then randomized to either a test group or a control group. Obviously, enrichment designs are most appropriate when patients who are biomarker negative are unlikely to benefit from the new treatment. Non-eligible patients are spared from adverse events, and overall safety of the trial is increased. Moreover, because the focus is on a targeted subgroup of patients, enrichment designs have high efficiency meaning smaller patient groups, faster trials, and hence overall cost reduction [52]. Sometimes the biomarker-negative patients can be included in a later separate study for the same treatment, but this is only meaningful if the treatment has proven to be successful for the targeted patients and if there is a reason to believe that biomarker-negative patients could benefit as well. Before enrichment designs are employed, it is important that the mechanism of the predictive biomarker is well understood. Enrichment designs are hence most appropriate in late phases of cancer drug development.


Fig. 22.2
Enrichment design, i.e. only biomarker positive patients are recruited into the clinical trial to receive experimental treatment
Umbrella Designs
Umbrella designs consist of two or more cohorts or sub-studies, e.g., two enrichment designs connected through an initial screening procedure. Patients are screened for predictive biomarkers and allocated into different cohorts (Fig. 22.3). Patients in cohort 1 are positive with respect to biomarker 1, patients in cohort 2 are positive with respect to biomarker 2, etc. Patients that are negative for all of the defined biomarkers are allocated to the last cohort. Within each of the biomarker-positive cohorts, patients are randomized to a targeted agent or a control group. Umbrella designs can be very useful, in particular, when a large number of patients are screened for low-prevalence biomarkers. As with enrichment designs, it is important that there is a strong rationale for using predictive biomarkers. These studies can be exploratory and have been used in different phases of drug development. The umbrella designs usually focus on a single histology or tumor subtype.


Fig. 22.3
Umbrella design, i.e. patients are screened for biomarkers and allocated into different marker-dependent substudies (different treatments) with subsequent randomization (study drug versus standard treatment)
Basket Designs
Different from umbrella designs, the basket designs are often used when several tumors or histological types are under investigation. Basket designs are of an explorative nature and can be appropriate for phase I targeted therapy studies. Patients are first screened and then allocated to cohorts depending on cancer type and molecular profile (Fig. 22.4). All patients within a cohort are then given the same targeted treatment.


Fig. 22.4
Basket design, i.e. multiple cohorts based on different tumour types or biomarker status are given the same experimental treatment, with or without randomization
Adaptive and Optimal Designs
Clinical trials are sequential in nature, and as a trial progresses, increasing amounts of data and information become available. The FDA has published guidance on adaptive design both for drugs and medical device clinical studies [53]. Adaptive designs allow a flexible approach to clinical trials enabling study teams to modify the design during the recruitment phase. Bayesian decision rules enable optimization of trial parameters based on real-time data at defined intervals to determine patient pathways in a “learn-as-you-go” principle [54]. The hope is that the FDA guidance will encourage the proper use of adaptive designs, reducing resource requirements and increasing the chance of study success and regulatory compliance. Optimal design theory can be used to find the most efficient designs. Adaptive designs and Bayesian analysis (Fig. 22.5) are employed to utilize the most up-to-date cumulative knowledge, and the use of surrogate endpoints together with early stopping rules can shorten the study period [55, 56].


Fig. 22.5
Adaptive design, i.e. modifying the design during the recruitment phase by optimizing trial parameters based on interim analyses of treatment outcomes
Biomarker Panels in Late-Phase Trials of Hematopoietic-Derived Blood Cancer: Acute Lymphoblastic and Acute Myeloid Leukemia
The Nordic pediatric trial of acute lymphoblastic leukemia (ALL; NOPHO ALL 2008) represent the standard therapy of patients in the age group 1–45 years in Iceland, Finland, Denmark, Lithuania, Norway and Sweden. Patients are stratified into three categories: normal, intermediate, and high risk for relapse. Therapy intensity is adjusted according to risk stratification [57]. This risk stratification is guided by a panel of markers, including white blood cell counts [58] and advanced flow cytometric determination of leukemic cell remnants, so-called minimal residual disease (MRD), after treatment. The impact of the biomarker panel in this trial is significant due to the increased toxicity experienced in the high-risk arm of therapy.
The Dutch hemato-oncology network (HOVON) has, in collaboration with the Swiss oncology society (SAKK), been running larger randomized phase III trials in acute myeloid leukemia (AML) patients 18–65 years of age [59]. The later trials have incorporated an increasing number of molecular markers [60]. Not only have the presence of such markers been used but also have exclusion of high-risk markers, e.g., the length mutation or internal tandem repeat in the juxta-membranous domain of the receptor tyrosine kinase FLT3. Like for NOPHO, a panel of markers has been formed to guide therapy, using flow cytometry-based minimal residual disease assessment (MRD) or NPM1 mutation-specific quantitative PCR. MRD is used to select intermediate-risk patients between allogenic and autologous hematopoietic stem cell transplantation. For the patients, this implicates careful selection between two cellular immunotherapies with large differences in mortality. Therapy-related mortality is below 5% in autologous HSCT, while approximately 20% in allogenic HSCT [61].
The use of single-gene mutations has been used in adult and older AML to guide therapy. The molecular target FLT3 has been explored since 2000, but results of FLT3-targeted therapy have been limited or even detrimental for the experimental group [62]. The third-generation FLT3 targeting agent quizartinib (AC-220) has been shown to have effect both in FLT3-mutated patients and to a certain degree in FLT3 wild-type AML cases. A phosphoproteomics panel examining proteins in AML cells has indicated a distinction between responders and nonresponders independent of the FLT3-ITD status [63].
Based on the heterogeneity of most aggressive cancers, it is maybe a surprise that single biomarkers are able to predict therapy responses. Failure of FLT3-ITD to determine successful inhibitor therapy is likely from a biological perspective, even before we examine the quality and efficiency of the inhibitors. One of the important questions that arise is how an ideal biomarker panel could be constructed.
Biomarker Panels in Early-Phase Trials of Mesenchymal-Derived Cancer: Sarcoma
Mesenchymal stem cells or progenitor cells of connective tissues represent the origin of sarcomas, a rare heterogeneous group of diseases often with a specific morphology and a clearly defined macro- and microanatomical location [64, 65]. Many of the various subtypes of sarcoma are characterized by defined chromosome translocations, recurrent gene amplifications, and mutations.
Clinical trials have taken advantage of the defined genetic features and are testing targeted therapy based on the signaling pathways involved. Multidimensional analysis of biomarkers for future prognostic classifiers that reflect inter- and intra-tumor heterogeneity is in development [66], but such patient-stratifying tools have not been employed in clinical trials so far. GIST is the most common subset of sarcoma with high expression of the receptor tyrosine kinase c-KIT, and most patients with inoperable or recurrent GIST obtain temporary and often long-term disease control with kinase inhibitor therapy [46]. Like most oncogene-driven tumors that are sensitive to small-molecule inhibitors, relapse or disease progression due to secondary mutations of the kinase is a clinical problem in these sarcomas. Acquired imatinib resistance is typically associated with substitutions between the first and second receptor kinase domains, rarely found in untreated tumors. A likely mechanism explaining secondary clonal evolution is gatekeeper mutations interfering with binding of imatinib at the receptor site [67]. The oncogene activations in c-KIT are however still the driving force and the main target for therapy. In lack of secondary mutations, feedback mechanisms may be responsible for reactivation of c-KIT. Another explanation suggested is a morphologic shift with altered immune phenotyping insensitive to imatinib emerging as a result of the targeted therapy [68]. Novel multi-target TKIs, either alone or in combination, may be effective in imatinib-resistant GIST; again, genotype biomarkers may predict the likelihood of response. Hence, design of clinical research protocols must take into account the impact of these molecular hallmarks.
Within the field of sarcoma, there is an ongoing search for effective targeted therapy related to pathway aberrancies detected by mutational biomarker analyses. In Norway, a population-based recording of mutational status in all new incidences of sarcoma over a period of 2–3 years has recently been initiated. This Norwegian Sarcoma Consortium (NoSarC) project assesses frozen tumor tissue according to a panel of approximately 900 mutations described in various types of cancers [69]. The project aims at increasing insight into molecular mechanism in sarcoma development as well as identifying aberrations which may be further exploited as targets for new therapeutic drugs. Potential targets will be validated in preclinical studies prior to further investigations in small-scale academic studies. The accumulation of functional information emerging from systematic genome sequencing may facilitate development of second-line personalized medicine in orphan cancer types for which the rarity hampers large-scale clinical trials.
One interesting trial design is the EORTC trial, “CREATE,” testing the ALK kinase inhibitor crizotinib in six defined rare diagnoses including three rare sarcoma subtypes (ClinicalTrials.gov Identifier: NCT01524926). However, specific ALK and/or MET pathway alterations in tumor tissue are not mandatory for patient registration, thereby allowing control patients for pathway-targeted therapy. Furthermore, substantial tumor material should be available for central review and molecular diagnostics, and previous therapy is permitted. Trials have previously demonstrated timely use of biomarkers in targeted therapy of rare diagnoses, including therapeutic antibodies against IGF-1R as well as the tyrosine kinase inhibitor pazopanib in soft tissue sarcomas [70, 71].
Summary and Discussion
Biomarker panels are an increasingly integrated part of clinical trials, used for pivotal decision-making. It is likely that combinations of biomarkers that involve tumor environment and stromal features will increase prognostic precision with immunotherapy and targeted therapy. Optimal trial design theory can be used for finding the most efficient designs. Adaptive designs and Bayesian designs are used to utilize the most up-to-date cumulative knowledge, and the use of surrogate endpoints together with early stopping rules can shorten the study period. Ethical perspectives need to be taken into account when designing a cancer study: for example, will the patients benefit from taking part in the trial?
FDA guides the process of obtaining an approved biomarker program in a clinical trial [72]. This secures the quality of the biomarker assays but has also likely limited the use of biomarker assays in many US trials.
Even if genomics alone as guidance for therapy is unlikely to prove relevant for all types of cancer, the proportion of actionable mutations is likely to be so high that cancer genetics may provide significant clinical benefit [20]. Therefore, genomic profiling may become relevant to some but not all cancer types. Development of functional biomarker assays are emerging and prepared for use in clinical trials, such as single-cell immune and signaling profiling or in vitro cancer sensitivity assays for selection of optimal therapy. AML patients responding to the combination of vitamin A, theophylline, and valproic acid displayed a low signaling signature of phospho-signaling proteins [73]. Functionality through in vitro testing of therapy response has been employed in single patients and with promising responses [7, 74]. Limitations of this in vitro drug sensitivity assay include the absence of combination testing and novel therapy availability.
Studies in AML and ALL, as well as in soft tissue sarcoma including GIST, potentially illustrate a robust use of biomarker panels. A combination of various modalities, from clinical data with cancer cell numbers via karyotype, gene expression, and mutational analyses, seems to guide therapy intensity at a higher accuracy. Similarly, surrogate markers for therapy effect need to be selected based on the nature of the disease, e.g., minimal residual disease determination by quantitative PCR in the presence of reliable markers or protein expression of the malignant clone by immunophenotype. The complexity of the decision tools for the physician seems to be increasing, and the future challenge will be to create a simplified and validated diagnostic system that provides precision medicine to the heterogeneous tumor diseases of our patients.
References
1.
Simon R. Biomarker based clinical trial design. Chin Clin Oncol. 2014 3(3):39. doi: 10.3978/j.issn.2304-3865.2014.02.03.
2.
DeVita VT Jr, Eggermont AM, Hellman S, Kerr DJ. Clinical cancer research: the past, present and the future. Nat Rev Clin Oncol. 2014;11(11):663–9. doi:10.1038/nrclinonc.2014.
3.
Schwaederle M, Zhao M, et al. Impact of precision medicine in diverse cancers: a meta-analysis of phase II clinical trials. J Clin Oncol. 2015;33(32):3817–25. doi:10.1200/JCO.2015.61.5997.
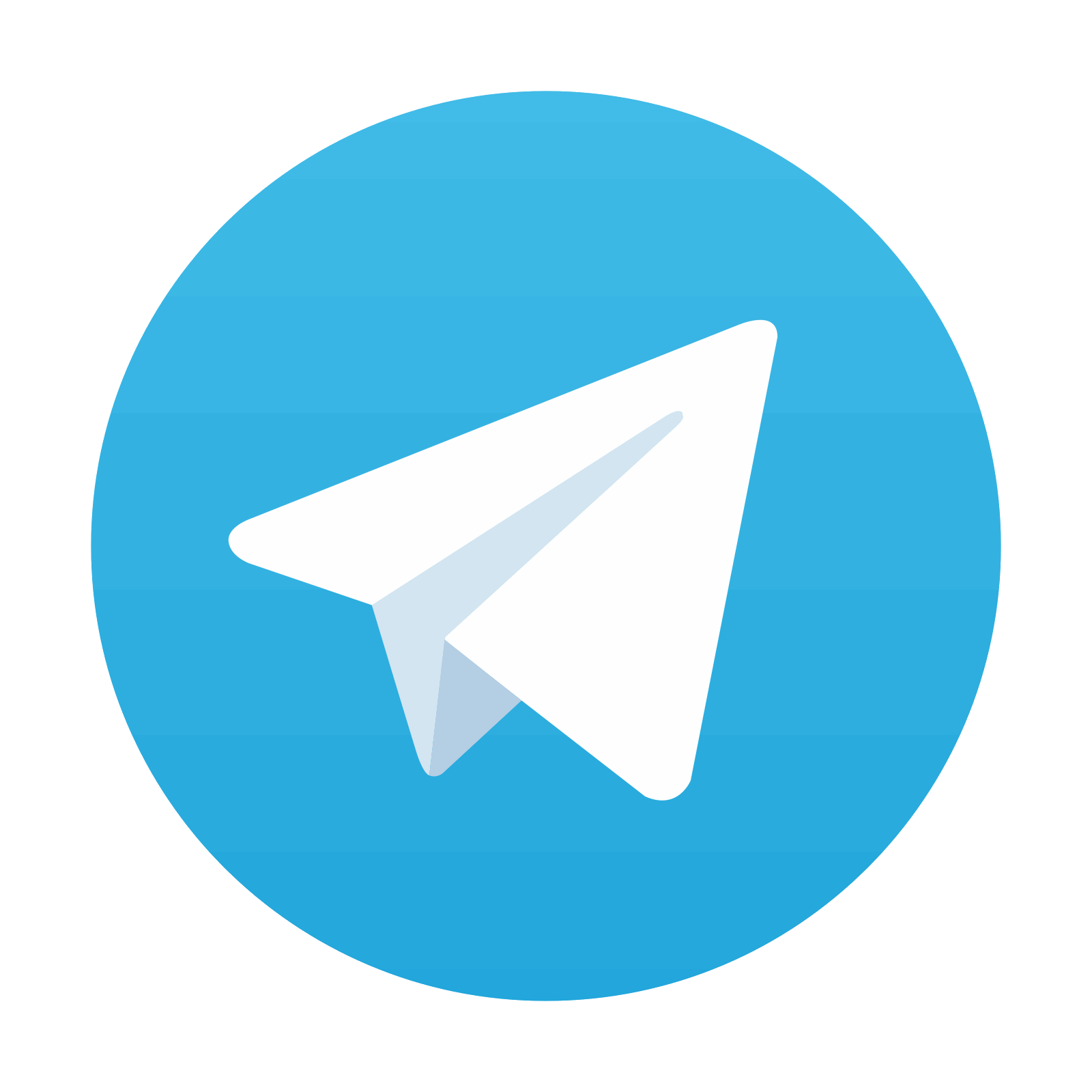
Stay updated, free articles. Join our Telegram channel

Full access? Get Clinical Tree
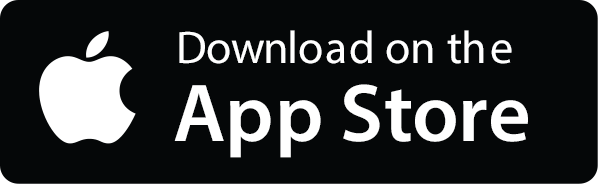
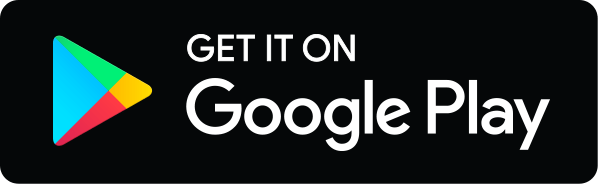