Biological Indicators, Chemical Indicators, and Parametric Release
Richard Bancroft
The number of microorganisms can decrease exponentially during exposure to a steady-state disinfection or sterilization process; only by infinite exposure to a defined antimicrobial process can the absence of all viable microorganisms be assured with certainty. At the same time, it is acknowledged that indefinite exposure to the antimicrobial agent is impractical and may have deleterious effects on the device or product being processed, especially when this involves sterilization by heat or irradiation. A compromise must be struck between assuring the sterility of the final device or product and ensuring that the device or product remains fit for purpose. For sterilization processes, as an example, the concept of a sterility assurance level (SAL) can therefore be used to “measure” or define the level of exposure to a sterilization process, with the result expressed in terms of a probability of a surviving microorganism (see chapter 2).1 Once defined, through validation studies, what process is needed in order to achieve a given disinfected or sterile state, the attributes of this process can then be monitored to ensure that the process is performing to its specification. These attributes may be chemical or physical in nature, and changes in them can alter the process effectiveness; these are known as process variables, with examples including time and temperature. The specified value for a process variable is known as a process parameter. Sterilization processes are particularly defined, or specified, according to their process parameters. Biological and chemical indicators (CIs), as well as physical sensors, are used to ensure that these process parameters are attained and are used both in defining this process specification as well as ensuring the process maintains its specification by means of process monitoring.

Commercial biological indicators (BIs) are products containing known numbers of disinfectant-resistant or sterilant-resistant microorganisms deposited on a carrier that are often in the form of either metal foil, paper strips, or discs; BIs are also available in a self-contained format (Figure 65.1). These self-contained BIs (SCBIs) consist of the spore-inoculated carrier and a separate sealed container with the microbiological recovery media all packaged together within an ampoule or a container. This packaging also contains a sterilant-permeable filter that allows the SCBI to be used without any disassembly. After processing, the recovery medium is released from its sealed container to allow it to be in contact with the spore-inoculated carrier, and the BI can be incubated as a whole unit. The BIs can also be created by directly inoculating a suspension of microorganisms directly onto or into a product or device. The BIs are often used within a process challenge device (PCD) that is designed to create a defined challenge to the process. For disinfection process monitoring, BIs typically use vegetative microorganisms (see chapter 65); bacterial endospores, or spores, are typically used for sterilization processes because they can be extremely resistant to inactivation. These microorganisms are also selected based on their safety to those using them. Any large numbers of microorganisms should be treated with care to minimize any opportunity for human infection, hence the use of microorganisms that do not require special containment facilities due to being nonpathogenic is always preferred. These reference microorganisms will typically have a resistance to the disinfection or sterilization process that is significantly greater than many common bioburden microorganisms (see chapter 64). The population of the test organism can vary according to the intended application of the BI; for disinfection process monitoring, vegetative microorganisms are used in a range of populations that may be as low as 1 × 103 microorganisms per BI, to much higher. For sterilization process monitoring, the population per BI is typically in the range of 1 × 105 to 5 × 106 bacterial endospores per BI. The BI chosen will provide a challenge to the disinfection or sterilization process through a combination of
the microorganism population, and its resistance to the process. For product loads that naturally have either high bioburden or very resistant microorganisms, the reference microorganisms can be used as a basis for understanding and then defining the disinfection or sterilization process.
the microorganism population, and its resistance to the process. For product loads that naturally have either high bioburden or very resistant microorganisms, the reference microorganisms can be used as a basis for understanding and then defining the disinfection or sterilization process.
Microorganisms are inoculated onto the BI carrier and are usually protected from mechanical abrasion or contamination by a disinfectant- or sterilant-permeable package, such as a glassine peel-pouch or similar, allowing the BI to be used either within this packaging or removed and used simply as an inoculated carrier. The BIs containing bacterial spores are typically more stable in use and storage, whereas BIs containing vegetative cells are much more labile and may need to be manufactured and used in a very short time, sometimes as short as a few hours. Commercially available BIs should be carefully stored according to the labeled storage conditions and not used beyond their labeled expiry date.
The carrier onto which the microorganisms are inoculated is usually selected depending on the type of process that the BI will be used to test or monitor. Hard, and largely inert, surfaces like stainless steel and some polymeric substrates have advantages in being robust and largely not interfering with the process they are used within; however, the specific design of these carriers must consider physical protection, during handling and use, of the surface where microorganisms are inoculated. Carriers based on cellulose, such as filter paper, can afford this physical protection but may not be suitable for use in some processes based on fluids such as found in washer-disinfectors and heat-based fluid sterilization processes. In addition, some oxidative chemistries may interact with these cellulosic materials or bind the disinfectant or sterilizing agent such that false-negative results are created.
As mentioned earlier, a BI can be manufactured by directly inoculating an actual device or product with microorganisms. This approach has the advantage that placement of the microorganisms on the device intended to be disinfected or sterilized can be in a location that has been considered to be the most challenging to the process. It can also assess any inherent interactions between the device or product and the microorganism; the resistance of a microorganism to a specific disinfection or sterilization process may be increased or reduced if it is exposed to physicochemical factors on or within the product being processed, such as pharmaceutical actives. This approach does create additional practical consequences; recovery of the microorganism can present challenges, particularly if enumeration of any surviving organisms is necessary because a recovery validation will need to be conducted to ensure that an acceptable microorganism recovery is acceptable. Typically, recovery methods will use either simple elution to remove the microorganisms or a more aggressive method such as abrasion with ballotini beads (small glass beads to aid in mechanical removal) or by ultrasonication (see chapter 64). The intent is to maximize recovery while minimizing potential damage or destruction of the microorganism. If the intent is to simply determine growth or no growth of the microorganism, it may be acceptable to immerse the entire device in recovery media and observe for the presence of growth by turbidity of the media. But there may be practical implications of this approach; examples include if the device is very large, such as a large endoscope, or ensuring that the recovery media is in contact with all parts of the device, including any internal channels or areas. Direct inoculation has other consequences too, such as the need to ensure that aseptic technique is observed throughout, from inoculation to recovery, and that any microorganism detected by growth must be determined to be the reference microorganism that was originally inoculated, and not an environmental contaminant.
The microorganism used in a BI is typically selected based on a prior knowledge of which microorganism is most resistant to a given disinfection or sterilization modality; for example, Geobacillus stearothermophilus spores are generally considered to be the most resistant to moist heat sterilization processes. There are of course other considerations for the most suitable microorganism; these may include safety (pathogenic microorganisms are generally avoided unless there are specific justifications why these should be used). Labile organisms may be also unsuitable. Depending on whether the process is a disinfection or sterilization
process, as mentioned earlier, the known microbial challenge is usually composed of aerobic bacterial endospores, predominantly Bacillus and Geobacillus species, although occasionally Clostridium species may be used, based on their resistance to a given sterilization process. The BIs are, therefore, characterized by the strain of test organism, the number of colony-forming units per BI, the D-value (decimal reduction value) determined under defined (and specified) conditions, and the Z-value (relating the heat resistance of a microorganism to changes in temperature) (see chapter 2).
process, as mentioned earlier, the known microbial challenge is usually composed of aerobic bacterial endospores, predominantly Bacillus and Geobacillus species, although occasionally Clostridium species may be used, based on their resistance to a given sterilization process. The BIs are, therefore, characterized by the strain of test organism, the number of colony-forming units per BI, the D-value (decimal reduction value) determined under defined (and specified) conditions, and the Z-value (relating the heat resistance of a microorganism to changes in temperature) (see chapter 2).
The International Organization for Standardization (ISO), based in Geneva, Switzerland, has published a range of product standards for BIs used in sterilization processes. The ISO 11138 series of standards gives prescriptive requirements for a range of BI properties for the different sterilization modalities. General requirements for BIs are given in ISO 11138-1,2 and specific requirements for BIs are given in ISO 11138-2 for ethylene oxide sterilization,3 ISO 11138-3 for moist heat sterilization,4 ISO 11138-4 for dry heat sterilization,5 and ISO 11138-5 for low-temperature steam and formaldehyde.6 Recommended reference strains of the microorganisms are as follows:
Steam and other moist heat processes: G stearothermophilus spores
Low temperature vaporized hydrogen peroxide processes: G stearothermophilus spores
Dry heat processes: Bacillus atrophaeus spores
Ethylene oxide sterilization: B atrophaeus spores
Note that BIs are not commonly used for ionizing radiation processes, but Bacillus pumilus has been used as the reference resistant organism in the past.
The ISO 11138 series of standards also specifies the spore population range, the D-value under defined conditions, the Z-value (where applicable), and standardized labeling requirements, including the lot and expiry date. The resistance of a microorganism to a disinfection or sterilization process is defined as the D-value, which is also sometimes known as the D10 value. The D-value is defined as the time or dose required to achieve inactivation of 90% of a population of the test microorganisms, under stated conditions. This 90% inactivation or population reduction is sometimes referred to as a 1 log reduction. The basis for the calculation for D-values is based on microbial inactivation typically following first-order reaction kinetics. When BIs are used for thermal disinfection or sterilization, the Z-value is used to describe the temperature susceptibility of the microorganism; this is more precisely defined as the change in temperature of the thermal disinfection or sterilization process that produces 10 times change in D-value. For example, if the Z-value is 10°C, this means that if the temperature of the process is increased by 10°C, there will be a 10-fold reduction in D-value of that microorganism at that temperature.

Following exposure to the disinfection or sterilization process, BIs must be cultured as soon as possible after the process in suitable media and under appropriate recovery conditions, as specified by the manufacturer. Handling of the BIs must be done aseptically in order to prevent contamination or cross-contamination. In the simplest approach to the use of BIs, they can be immersed in the appropriate recovery media and incubated at the correct temperature for the minimum incubation time, and the result expressed as either the presence of growth or no growth, usually detected by turbidity. A much more complicated approach counts, or enumerates, any surviving organisms, first by removing the microorganisms from the carrier, then serially diluting and plating onto recovery media (agar) plates, before incubation. In order to enumerate a BI, the viable microorganisms must be removed from their carrier, ideally as soon as possible after being exposed to the disinfection or sterilization process, as microorganisms that may have been damaged, but still viable, may not be able to survive without growth media. Removal from the carrier can be carried out using a number of methods that are not damaging to the microorganisms (see chapter 64). These include elution, gentle mechanical abrasion using sterile ballotini beads (small glass beads) in water, or by ultrasonication. The recovery efficacy of this process should be determined as well as the limitation of microbial detection during the recovery and incubation process. Once the microorganisms are in solution, they can be enumerated by plating onto agar plates and incubated. By assuming that each colony that forms originates from a single microorganism, it is possible to then calculate the number of microorganisms that survived the process. It is very difficult to count the colonies that form if there are more than 250 colonies per typical agar plate; because it is not possible to know if there are large numbers of microorganisms surviving from the BI, it is normal practice to serially dilute the extracted sample using sterile water or another diluent. Typically, 1 mL of sample is added to 9 mL of sterile water, thus creating a 10-fold reduction in count per dilution; a number of these dilutions can then be plated onto recovery media for enumeration.
The recovery media can have a significant effect on the performance of the BI as a whole; small changes in the formulation of the recovery medium can have great consequences for the growth of the organism. If no growth occurs, the level of lethality can be calculated to be greater than the log arithm of the population of the BI; in the event of growth occurring, it is sometimes considered necessary to establish whether the growth is derived from the original inoculum or whether it represents accidental contamination during handling or culturing.
During incubation, detection of growth of the reference microorganism can be by a number of methods. The most
basic of these is the presence of turbidity (or cloudiness in the media); this may be quite difficult to see in an SCBI format, hence commercially available SCBIs typically include a pH indicator within the recovery media. As microorganism germination and subsequent growth occurs, there is an associated drop in pH as the microorganisms digest the recovery media. This drop in pH causes the pH indicator to change color, hence showing microorganism growth and a positive (ie, growth) result. The incubation time taken to germinate and grow these microorganisms is dependent on many factors, but a 7-day incubation is a standard reference incubation time for normal growth conditions of bacterial spores. Many commercially available BIs have been validated by their manufacturers to have a significantly shorter incubation time than the reference period and can reduce the incubation time to typically around 24 hours. In recent years, other methods of detecting early germination and growth of the reference microorganism have reduced the potential incubation time down to as little as 20 minutes. This has been achieved by adding a nonfluorescent α-glucosidase substrate to the recovery media; as the microorganisms germinate, the substrate is metabolized by the microorganisms, resulting in formation of a fluorescent compound. This fluorescence can be detected using purpose-made incubators that incorporate fluorescent detection capabilities. Other methods for detection of microbial growth are also available.
basic of these is the presence of turbidity (or cloudiness in the media); this may be quite difficult to see in an SCBI format, hence commercially available SCBIs typically include a pH indicator within the recovery media. As microorganism germination and subsequent growth occurs, there is an associated drop in pH as the microorganisms digest the recovery media. This drop in pH causes the pH indicator to change color, hence showing microorganism growth and a positive (ie, growth) result. The incubation time taken to germinate and grow these microorganisms is dependent on many factors, but a 7-day incubation is a standard reference incubation time for normal growth conditions of bacterial spores. Many commercially available BIs have been validated by their manufacturers to have a significantly shorter incubation time than the reference period and can reduce the incubation time to typically around 24 hours. In recent years, other methods of detecting early germination and growth of the reference microorganism have reduced the potential incubation time down to as little as 20 minutes. This has been achieved by adding a nonfluorescent α-glucosidase substrate to the recovery media; as the microorganisms germinate, the substrate is metabolized by the microorganisms, resulting in formation of a fluorescent compound. This fluorescence can be detected using purpose-made incubators that incorporate fluorescent detection capabilities. Other methods for detection of microbial growth are also available.

The BIs are used extensively in disinfection and sterilization cycle validation and in routine monitoring of these processes. They integrate all the process variables such as time, temperature, gas or chemical concentration, humidity, etc. In common with CIs (see the following text), because BIs are placed directly in the container or load, they will reflect the actual process conditions in or on the product or load itself, rather than just in the environment in which the container or load has been placed. As mentioned earlier, BIs generally use a bacterial or other microorganism strain that has been specially selected for its high resistance to the given process. Moreover, because the microorganisms on the BIs are likely to present considerably more of a challenge in terms of population and resistance to the process than the expected bioburden of the product, then considerable confidence can be placed in the expected level of process or sterility assurance associated with the process.
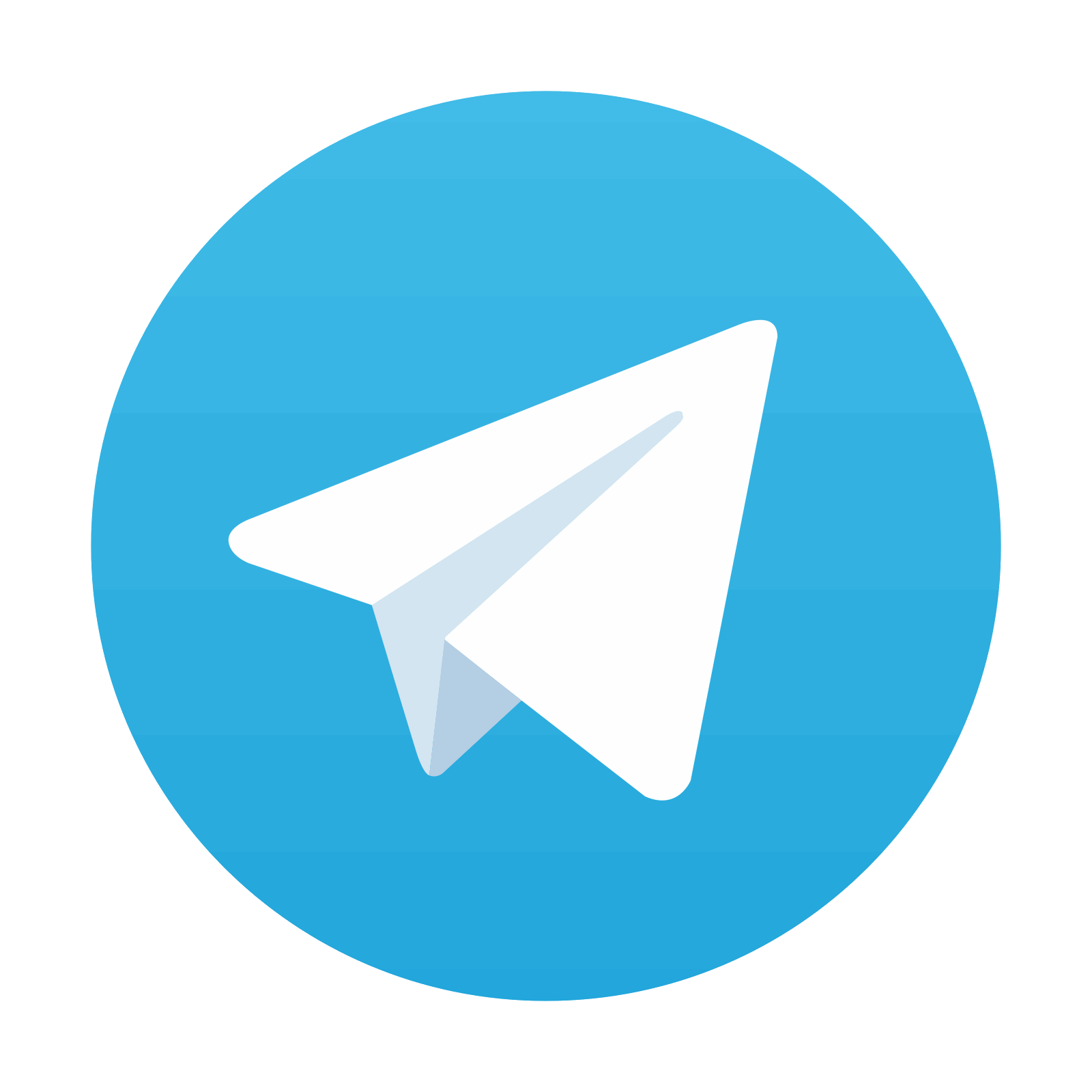
Stay updated, free articles. Join our Telegram channel

Full access? Get Clinical Tree
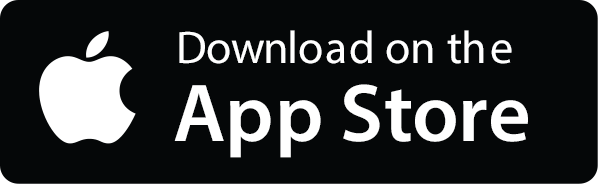
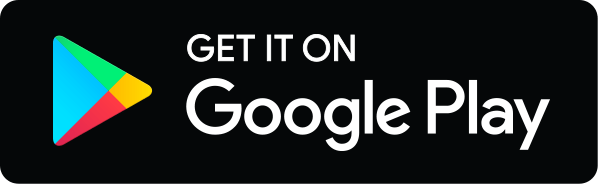