Fig. 2.1
Classification of biodegradable natural polymers
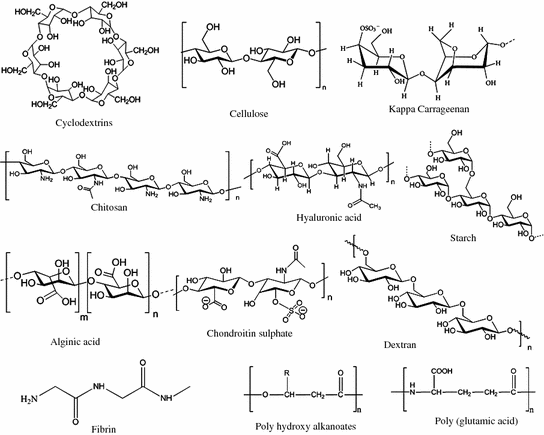
Fig. 2.2
Chemical structures of natural polymers
Natural Polymers of Plant Origin
Polysaccharide Based Polymers
Cyclodextrins
Cyclodextrins (CDs), also known as (cycloamyloses) are cyclic oligosaccharides consisting of several glucopyranose units obtained from the enzymatic degradation of starch by cyclomaltodextrin glucanotransferase, naturally secreted by B. macerans [1–3]. There are three major types of CDs, which include α-, β- and γ- (CD) with six, seven or eight d-(+)-glucopyranose units, respectively. These oligosaccharides consist of α-d-glucopyranose units with (α-1, 4)-linkages possessing a basket-shaped topology with a hydrophilic outer surface and a lipophilic central cavity. Derivatives of CD such as (2-hydroxy propyl) β-CD, γ-CD (HPβCD, HPγCD), random methyl-β-cyclodextrin (RM-β-CD), sulfobutylether CD (SBEβCD) and many other hydrophilic, hydrophobic, ionizable derivatives of CD are being explored. CDs are chemically stable in neutral and basic conditions, whereas they undergo non-enzymatic hydrolysis in acid conditions to yield glucose, maltose, and non-cyclic oligosaccharides [4, 5].
CDs exhibit multiple applications in versatile areas [6–8]. CD inclusion complexes containing guest molecules improve physicochemical properties, which in turn increase the bioavailability of drugs. The release of drugs is mediated by action of heat/enzymes/pH changes. The dimethyl derivatives of CDs are extremely water soluble, which enhances the bioavailability of lipophilic drugs. They also act as absorption and solubilization enhancers in nasal drug delivery [5, 9, 10]. The use of random methyl CD avoids in vivo crystallization due to its amorphous nature. These polymers were also being investigated for application in intestinal gene transfer. The use of CDs as non-viral vectors for gene delivery results in increased transfection efficiency with high levels of reporter gene expression and low toxicity [11–13]. CDs also serve as promising carriers for anti-cancer drug delivery in tumour therapy [14]. They also have proven suitability as an additive in ophthalmic preparations, and rectal and dermal delivery systems. They are responsible for improving the stability and solubility of various ophthalmic preparations. The problem, however, of decreased bioavailability associated with the use of CDs can be overcome by using viscosity enhancers in the final formulation [15, 16]. They also serve as carriers for peptides, oligonucleotides and proteins because of their cellular interactions. Due to their ability to recognize the surface properties of lipoprotein particles, they have also been explored as diagnostic tools for cholesterol measurement. Recent research has shown greater control of drug release by incorporation of CDs into polymeric drug delivery systems [17].
Cellulose and Hemicellulose
Cellulose is an organic polysaccharide with the formula (C6H10O5)n, consisting of a linear chain of several hundred to over ten thousand β (1 → 4) linked d-glucose units [18, 19]. Cellulose is the most abundant organic polymer and is the essential structural component of cell wall in plant cells. The parallel aligned cellulose molecule forms crystalline microfibril that provides mechanical strength and resistance to enzymatic attack. Cellulose is insoluble in water and indigestible by the human body [19, 20]. Cellulose obtained from fibrous materials such as wood and cotton can be mechanically disintegrated to produce powdered cellulose, which has been used in the pharmaceutical industry as filler in tablets. Many cellulose derivatives have been prepared by the hydroxyl moieties on the D-glucopyranose unit of cellulose. Microcrystalline cellulose, cellulose esters, cellulose ethers, and cross linked or graft copolymers are widely used in the pharmaceutical industry.
Microcrystalline cellulose (MCC) is mainly used in the pharmaceutical industry as a diluent/binder in tablets for both granulation and direct compression processes. Treating high quality cellulose with hydrochloric acid to the desired level yields purified microcrystalline cellulose which is a partially depolymerized cellulose [21]. Membrane controlled systems or monolithic matrix drug delivery systems obtained from cellulose derivatives provide controlled release of drugs. Film coating techniques are employed for the manufacture of membrane controlled release systems, which include enteric coated dosage forms and semi-permeable membranes in osmotic pump delivery systems.
Hydroxypropylmethylcellulose (HPMC) is a partly O-methylated and O-(2-hydroxypropylated) cellulose ether derivative. It is a widely used cellulose derivative, used as excipient in controlled release drug delivery systems owing to its gel forming properties. Carboxymethylcellulose has been employed for controlling the release of soluble drugs; the polymer can sustain release over an extended period of time enabling a zero order release profile [22]. Hydroxypropylmethylcellulose monolithic matrix systems show similar dissolution profiles as a commercial osmotic pump system for glipizide, a drug with low solubility. In addition it provides superior in vivo performance in terms of matrix resistance to the destructive forces within the gastrointestinal tract [23].
Hemicellulose is a heteropolymer consisting of a matrix of polysaccharides, such as arabinoxylans, present along with cellulose in almost all plant cell walls. Unlike cellulose, hemicellulose has a random, amorphous structure with little mechanical strength. Hemicelluloses include xyloglycans, xylans, mannans and glucomannans, and β-(1 → 3, 1 → 4)-glucans [24]. Their extraction from the plant cell wall is carried out with the aid of strong alkali. Hemicellulose consists of shorter chains and 500–3,000 sugar units. It is branched, unlike cellulose which is unbranched. Hemicelluloses have β-(1 → 4)-linked backbones with an equatorial configuration. Although xyloglycans have a similar backbone as cellulose, they contain xylose branches in 3 of every 4 glucose monomers, while the β-1, 4-linked d-xylan backbone of arabinoxylan contains arabinose branches [25].
Starch
Starch is a common polysaccharide that is found mainly in plants. Starch acts as a storage material in plants. Chemically, it is composed of recurring units of glucopyranose in an alpha d-(1,4) linkage. It is comprised of two polymers, namely amylose (a non-branching helical polymer consisting of α-1, 4 linked d-glucose monomers) and amylopectin (a highly branched polymer consisting of both α-1, 4 and α-1, 6 linked d-glucose monomers), on hydrolysis it yields the monosaccharide, glucose [26].
Usually, a plasticizer is added to starch to overcome brittleness and to make it easily processed. The amount of plasticizer added determines final physical properties of starch. Generally water is the common plasticizer used. Other plasticizers like low molecular weight alcohols are added to produce thermoplastic starches.
This abundantly available polymer has been investigated for various biomedical applications such as scaffolds for bone tissue engineering applications, bone cements, and as drug delivery systems. Starch based microparticles and fiber mesh scaffolds are used as carriers for osteoblasts, bone marrow stromal cells and endothelial cells facilitating bone tissue engineering and vascularization [26]. Moreover, starch is blended with other natural or synthetic polymers to improve properties and make it suitable for various applications. For instance, starch−alginate beads were developed to obtain a detained release of model peptide drug phenylalanine. The release of drugs can be altered by the number of alginate coatings outside the bead. Similarly, blends of starch with other polymers like poly lactic acid, polycaprolactone, polyethylene have been researched for various specific indications. These modified derivatives of starch exhibit altered properties related to degradation profile and physical properties of the polymer [27–29].
Inulin
Inulins are a group of naturally occurring polysaccharides produced by many types of plants including onion, garlic, chicory, and artichoke. Chicory acts as a major source for industrial production. They belong to a group of carbohydrates known as fructans. Structurally, inulins consist of 2 to more than 60 fructose molecules linked by β-2, 1-bonds and a glucosyl unit at the reducing end. Due to its ability to maintain inertness in upper GIT, it is applicable in developing colon specific drug delivery systems. It is degraded by colonic microflora (more specifically, bifidobacteria). The rate of degradation of inulin derivatives depend on the degree of substitution. Highly substituted inulin has higher mechanical strength, which in turn slows the degradation process [30].
For colon specific delivery, polysaccharides like inulin, pectin, dextran, etc., remain intact in GIT and show specific digestion and degradation at the colon by microflora in that region. The β-2,1 osidic bonds are not significantly hydrolyzed by enzymes from the endogenous secretion of the human digestive tract. For this purpose, biodegradable colon-specific films were developed in combination with Eudragit® RS. Apart from this, methylated inulin derivatives and inulin-azo hydrogels were investigated for colon specific delivery. Research to enhance the dissolution rate and to control the rate of drug release for poorly water soluble drug Irbesartan has proven successful. Inulin and its analog sinistrin are used to help measure kidney function by determining the glomerular filtration rate. Inulin-based glycopolymers have great potentials as cell-targeted drug carriers. Also, inulin is a superior lyoprotectant of PEGylated lipoplexes compared with dextran [30–33].
Pectin
Pectin is a complex heteropolysaccharide, found both in the cell walls of plants and between the cell walls. It is produced commercially as a white to light brown powder, mainly extracted from citrus fruits. Pectins are anionic polysaccharides composed of a backbone of poly-(1-4)-α-d-galacturonic acid [34].
Pectins are commercially available as low methoxy (LM) pectin (degree of esterification (DE) <50 %) and high methoxy (HM) pectin (DE > 50 %). LM pectins form a gel in the presence of divalent ions such as Ca2+, and can gel in the absence of Ca2+ when the pH is below about 3.3.
Pectin remains intact in the upper GIT and is degraded by colonic microflora, which makes it suitable for designing colon specific drug delivery systems administered via oral route [35, 36]. Amidated pectins in which some of the carboxylic acid groups are amidated are more tolerant to pH variations. They are used to develop a multiparticulate system (hydrogel beads) for site specific delivery to the colon [37]. The properties of this system can be altered using chitosan, which controls the release rate of the drug [38]. The potential of in situ gelling xyloglucan/pectin mixture has been explored for the oral administration of paracetamol. The reported gelation characteristics of this mixture are a result of a combination of both thermal and ion responsiveness due to a synergistic interaction between these two polymers [39]. There have also been efforts to develop nanocarrier based anti-cancer drug delivery systems. Fabricated pectin nanocarriers loaded with oxaliplatin have been investigated as a potential targeted anticancer-drug therapy by incorporating super paramagnetic iron oxide nanoparticles (SPIONs) [40]. Pectin has also been explored as a carrier to develop an intra gastric floating drug delivery system using calcium pectinate gel (CaPG) beads containing carbonate salts. All the types of pectin (LM, HM, and AM) protect the liposomes against aggregation during storage. Pectin coated liposomes have been investigated as potential drug delivery systems [41]. Other possibilities include pectin–chitin/nano CaCO3 composite scaffolds in tissue engineering and freeze-dried chitosan/pectin nasal inserts for antipsychotic drug delivery [42, 43]. It is used in food industry as a gelling agent particularly in jams and jellies. It is also used in fillings, medicines, sweets, as a stabilizer in fruit juices and milk drinks, and as a source of dietary fiber.
Glucomannan
Konjac glucomannan (KGM) is a straight chained hydrocolloidal polysaccharide of the mannan family. Glucomannan consists of β-1,4 linked d-mannose and d-glucose with an acetyl side chain on some backbone units (8 % branching of glucosyl linkage). The mannose: glucose ratio may differ depending on the source [44]. The presence of acetyl group contributes to its swelling capacity and solubility, thus making it a soluble natural polymer with high viscosity and water retaining capacity. Konjac glucomannan is the most commonly used glucomannan, which is obtained through the extraction of tubers from the plant Amorphophallus konjac belonging to the ulmaceae family.
KGM has been investigated as an excipient for a controlled release drug delivery system with or without combination with other polymers. Modification of the chemical structure is another approach for increasing its effectiveness as an excipient for controlled release delivery devices. The combination of KGM and xanthan gum in matrix tablets has been shown to effectively retard drug release by stabilization of the gel phase of the tablets by a network of intermolecular hydrogen bonds between the two polymers [45]. KGM gels also control the release of theophylline and diltiazem for 8 h, but they are region dependent, since the degree of acetylation changes in KGM [46]. KGM is used to form hydrophilic cylinders and particles for the controlled release of DNA [47]. Cross linked KGM with disodium trimetaphosphate forms cross linking density and enzymatic degradation dependent upon the hydrogel system with sustained release of hydrocortisone [45]. The use of a drug binding effector like graphene oxide to functionalize hydrogel based on KGM/sodium alginate has been found to effectively control the release rate of 5-Fluorouracil. Gels based on copolymers of KGM with acrylic acid have been investigated for colon specific drug delivery. A pulsatile capsule based on KGM/HPMC/lactose has also been designed to deliver 5-ASA specifically to the colon.
Guar Gum
Guar gum, also called guaran, is a galactomannan and a non-ionic polysaccharide obtained from the seeds of Cyamopsis tetragonolobus and is composed of mannose and galactose sugars. It consists of a linear backbone with (1, 4) mannose residues to which 1, 6-linked galactose residues are linked to every second mannose, thereby forming short side branches on the backbone [48]. DAVANAT®, a modified version of guar-gum, is being developed by Pro-pharmaceuticals, Inc. It is not affected by ionic strength but is sensitive to changes in pH and temperature extremes. The pH of 1 % w/v aqueous dispersion varies from 5 to 7. Strong acids and strong alkaline conditions tend to reduce viscosity due to acid/base catalytic reactions. It is dispersed in hot and cold water, and forms a highly viscous thixotropic gel. It is insoluble in organic solvents [49].
Guar-gum exhibits potential applications in various industries such as pharmaceutical formulations, cosmetic, food, textile industries, etc. This hydro colloidal agent is used as a binder and disintegrant in solid dosage forms, and as a suspending, thickening and stabilizing agent in liquid formulations [48]. It is a non-toxic, biodegradable polysaccharide applicable for colon targeting drug delivery, controlled matrix for intestinal delivery of peptides, potential hydrophilic carrier for oral controlled release for drugs with variable solubility, site specific targeting interpenetrating network hydrogels formed from cross-linked guar gum derivatives, and as a compression coat [50, 51]. Synthetic derivatives of hydrogels like guar acetate, guar phthalate, and guar acetate phthalate have been investigated for various pharmaceutical applications. Low-swelling index derivatives of guar-gum are used to retard premature drug release. Such derivatives are obtained by cross-linking gum with glutaraldehyde/trisodiumtrimetaphosphate. These derivatives have been explored for colon specific targeting. The properties of swelling (to retard the drug release in gastric environment) and ability to release the drug at colon (by enzymatic degradation of polymer by colonic microflora) make it a potential material for colon specific delivery [51, 52]. There are potential applications of this polysaccharide: hydrogel based on acryloyl guar gum as a carrier for the slow release of l-DOPA and l-tyrosine, hydrophilic carrier for metoprolol tartarate, trimetazidine hydrochloride etc., pH sensitive hydrogels based on guar-gum/poly (acrylic acid), poly (n-Iso propyl acrylamide), glutaraldehyde cross-linked derivatives, alginate-guar gum based controlled matrix delivery system for delivery of bovine serum albumin (BSA), and colon targeted system of 5-Fluorouracil [50, 51, 53–57]. Other applications include use as a thickener in toothpaste, conditioner in shampoos, and retardation of ice crystal growth.
Arabinogalactan
Arabinogalactan (AG) is a highly branched natural polysaccharide comprised of arabinose and galactose monosaccharides present in furanose configuration. This biopolymer is obtained from both plant (Larix occidentalis) and microbial origin. The arabinan portion of the polymer is a complex branched structure possessing α-(1–3), α-(1–5), and β-(1–2) glycosidic linkages, usually capped with mycolic acids. The microbial AG is a major structural component of the mycobacterial cell wall. It is often found attached to proteins. The resulting arabinogalactan protein (AGP) functions as an intercellular signaling molecule. AGPs belong to a class of glycoproteins with a hydroxyproline-rich core protein along with arabinose and galactose-rich polysaccharide units [58–60].
The high water solubility, biocompatibility, biodegradability, and the ease of chemical modification in aqueous media make it a potential material for development of scaffolds applicable in tissue engineering. The oxidized polymer is cross linked with polyamines like chitosan, spermine, and spermidine to form three dimensional scaffolds. The degree of oxidation alters the pore size and degradation rate of these scaffolds. AG–chitosan sponges were found to exhibit high swelling behavior in aqueous media; they have suitable pore size for cell growth. This polysaccharide has been explored for hepatic drug delivery [61]. AG has properties that make it suitable as a carrier for delivering diagnostic or therapeutic agents to hepatocytes via the asialoglycoprotein receptor. This polymer also plays a role as a carrier for drug formulations [62]. For instance amphotericin-B (AM-B), a standard antifungal agent, is conjugated to both AG and AGP and investigated in two different studies. These conjugates show increased stability and solubility of AM-B in aqueous media and also reduced toxicity with a high degree of biocompatibility [62–65]. Apart from pharmaceutical applications, it possesses some other industrial uses. It is a major component of many gums, including gum arabic and gum ghatti. Gum arabic finds its application as a valuable additive in the food and candy industries. The extent to which the AGPs in gum arabic are responsible for remarkable properties has yet to be investigated.
Carrageenan
Carrageenans belong to a family of natural linear sulfated carbohydrates extracted from red edible seaweeds. Carrageenans are formed by alternate units of d-galactose and 3, 6-anhydro-galactose (3, 6-AG) joined by α-1, 3 and β-1, 4-glycosidic linkage. There are different types of carrageenans, which are classified according to their differences in solubility, ester sulphate content and amount of 3, 6-AG. Among λ, κ, ι, ε, μ, types kappa, iota, lambda types are major kinds [66–68].
Kappa forms strong, rigid gels in the presence of potassium ions. It has an ester sulfate content of about 25–30 % and a 3, 6-AG content of about 28–35 %. κ is soluble in hot solution.
Iota forms soft gels in the presence of calcium ions. It has an ester sulfate content of about 28–30 % and a 3, 6-AG content of about 25–30 %.
Lambda does not gel and is used to thicken dairy products. It has an ester sulfate content of about 32–39 % and no content of 3, 6–AG. λ-type is readily soluble in cold or hot aqueous solution. λ-carrageenan exhibits the highest antioxidant and free radical scavenging activity.
Commercially, it is available as salts of sodium, potassium, calcium or a mixture of these, which in turn determines the physical properties of carrageenan. These salts are generally water soluble and form highly viscous solutions. Viscosity depends on the type of carrageenan, molecular weight, concentration, temperature, and the presence of other solutes. It is depolymerised at low pH (especially pH ≤3.0) by hydrolysis of glycosidic linkages at high temperature [69].
They are widely used in the food industry for their gelling, thickening, and stabilizing properties. Their main application is in dairy and meat products due to their strong binding to food proteins. λ and κ combine easily with milk proteins to improve solubility and texture. Carrageenan-induced rat paw edema is a widely used animal model to determine anti-inflammatory activity for evaluation of pain [69]. They have been investigated for use in delivery systems for controlled release of drugs [70]. Gelatin–carageenan hydrogel loaded with Quercetin, cross linked carageenan beads for controlled drug release, temperature responsive κ-carageenan angels, buckle drug delivery by lyophilized wafers comprising carageenan and pluronics acid, trans-sclera delivery of macromolecules by carageenan/methylcellulose polymeric systems, carboxy-methylated kappa-carageenan for intestinal-targeted delivery of bioactive macromolecules, microparticles based on lection-functionalized carboxy-methylated kappa-carageenan for oral insulin delivery, and carageenan–gelatin mucoadhesive systems for ion-exchange based ophthalmic delivery all prove the potential of this sulfated Polyglactin in versatile areas [66, 67, 70–75].
Protein Based Polymers
Soy Bean
The soy or soya bean is rich in protein (40–50 %), carbohydrates (26–30 %), and lipids (20–30 %). It is obtained from an annual plant, Glycine max, a species of legume native. Soy flour, soy concentrate and soy isolate are different soy bean products with different protein content among which isolated soy protein has the highest protein proportion (90 %) on dry weight basis. The major soy proteins in the isolate are glycine or 11S and β–conglycinin or 7S; it also contains isoflavones like genistein and daidzein [76]. There are many desirable characteristics associated with this protein, which makes it an excellent material of investigation for making drug delivery devices and tissue engineering applications. Soy bean exhibits excellent thermal stability, hydrolytic stability, melt processability, storage stability, biodegradability, and abundant availability, all of which make it an adaptable material for various purposes [77]. Soy bean also exhibits tailoring flexibility where the degree of cross linking can be modified to make it suitable for a particular purpose. Highly versatile properties of soy protein based thermoplastics make them suitable for many biomedical applications [78]. Soy protein based double layered control delivery devices have also been investigated [77].
Transgenic Plant Based Collagen
Collagen is a group of naturally occurring proteins present in the form of fibrils and forms a major part of the connective tissue. This is generally derived from animal sources, but collagen derived from these sources exhibits immunogenicity. Type I human collagen obtained from transgenic tobacco is a breakthrough technology invented by Collplant Ltd. of NesTziyona, Israel. There are several challenges to be met, for example development of transgenic tobacco plants that are able to develop human collagen, and extraction methods successfully overcome by Collplant from this new source. This development requires high-level genetic engineering combined with general plant genetics [79]. Plant engineering based pharmaceuticals are feasible alternatives to conventional fermentation based expression models. Vergenix™ wound dressing, a tobacco-derived collagen sponge, is used for chronic wounds and skin ulcers (pressure sores and diabetic wounds). Collagen plays a major role from medical devices to tissue repair [80]. A detailed review of collagen types and their applications is given in later sections.
Polyesters from Higher Plants
Polyesters, namely cutin and suberin, respectively present in epidermal cell walls and the inner periderm layer in the higher plants protect them from physical, chemical, and biological factors in the environment. These are glycerolipid polymers associated with cell walls in higher plants. Cutin, an insoluble cuticular polymer, is composed of hydroxy and hydroxy epoxy fatty acids, whereas suberin is composed of aromatic and aliphatic domains. These polyesters are degraded by enzymatic degradation/alkaline hydrolysis of ester linkages. These polyesters allow the tailoring of specific properties, making them suitable for drug delivery applications. Abundant availability, sustainability, renewability and biodegradability are some of the desirable characteristics of these biopolymers [81].
Natural Polymers of Animal Origin
Polysaccharide Based Polymers
Chitosan
Chitosan is a linear polysaccharide composed of randomly distributed β-linked d-glucosamine and n-acetyl-d-glucosamine. It is a naturally occurring cationic polysaccharide derived by partial n-deacetylation of chitin from shrimp and crustacean shells. The pKa value of chitosan is about 6.2–7; it is insoluble in neutral and alkaline pH solutions. The degradation rate mainly depends on the degree of deacetylation that occurs by hydrolysis of glycosidic links of acetylated residues in the presence of lysozyme. Chitosan adopts a more extended and coiled conformation at a high or low degrees of deacetylation respectively. Chemical modifications due to the presence of amino group at C-2 and primary and secondary hydroxyl groups at C-6 and C-3 improve mechanical properties and impart new biological activities in chitosan. The chief constituent in chitosan is glucosamine, a natural material present in the body and used to produce glycosaminoglycans. Chitosan and its derivatives are the most extensively investigated materials for pharmaceutical and biomedical applications. The cationic property of chitosan imparts key bioactive properties like biodegradability, biocompatibility, and a microbicidal and mucoadhesive nature. Amenability of chitosan to different chemical modifications makes it suitable for a broad range of therapeutic applications. Each modification imparts a different biomedical or pharmaceutical application. For example, sulfated chitosan exhibits antioxidant activity [6, 7, 82, 83].
Chitin consists mostly of n-acetyl-d-glucosamine-unit. Most units are deacetylated to d-glucosamine-units during the preparations of chitosan. Chitosan is a linear polymer of β-d glucopyranose units characterized by a degree of deacetylation. It is structurally similar to cellulose, but it has –NHCOCH3 (acetamide groups) at C-2 position [84].
Key features of chitosan: The amino and hydroxyl groups of chitosan that are chemically modifiable make it a highly versatile molecule for various applications (Table 2.1). The metabolism of chitosan by lysozyme makes it biodegradable. Chitosan exhibits a pH-sensitive behavior as a weak poly-base because of the large quantities of amino groups on its chain. At low pH conditions, the amino groups of chitosan are protonated and exhibit pH-sensitive swelling. Chitosan dissolves easily at low pH, while it is insoluble at higher pH ranges. Dialdehydecrosslinkers like glyoxal are used to cross link chitosan molecules to modify the properties of the molecules. Glutaraldehyde reduces the antigenicity of the material. Natural crosslinkers like genipin are gaining wide acceptance for crosslinking chitosan. Chitosan is found to be biologically renewable, bioadhesive, biocompatible, biofunctional, nonantigenic, and nontoxic. Chitosan, which is structurally similar to glycosaminoglycan (GAG) found in extracellular matrices (ECM) available in native articular cartilage, plays a key role in modulating chondrocytes morphology, differentiation and function, and has application in cartilage engineering. It also exhibits biosensor and antihyperlipidemic actions [82, 83, 85].
Application | Role of chitosan |
---|---|
Biological properties | Wound healing, osteogenesis, biodegradation |
Vaccine and drug delivery by chitosan particles: | Mucoadhesive property of chitosan improves the bioavailability of peptide in GIT |
1. Peptide drug delivery | Antigen encapsulated chitosan microparticles and nanoparticles |
2. Ophthalmic drug delivery | |
3. Targeted drug delivery | |
Promising carriers for nasal and mucosal vaccine delivery | |
Cyclosporine A loaded chitosan nanoparticles | |
Colon targeting by corticosteroid loaded chitosan microspheres | |
Gene delivery vehicle | Topical application of plasmid-DNA based chitosan nanoparticles |
Regenerative medicine | Chitosan-gelatin wound dressings |
Regeneration of various tissues like skin, bone, liver, cartilage and nerve | |
Hydrogels | Chitosan/β-glycerophosphate hydrogel loaded with anti cancer agents against breast cancer/cervical cancer |
Hyaluronan
Hyaluronic acid (HA), also called hyaluronan, is a high molecular weight (105–107 Da) naturally occurring biodegradable polymer. This name is derived from “hyalos” (Greek for glass + uric acid). HA is an unbranched non-sulfated glycosaminoglycan (GAG) composed of repeating disaccharides (β-1, 4-d-glucuronic acid (known as uronic acid) and β-1, 3-n-acetyl-d-glucosamide). HA is a polyanion that can self-associate and that can also bind water molecules when not bound to other molecules. HA, one of the major elements in the ECM of vertebrate tissues is also found in almost all body fluids and tissues, such as the synovial fluid, the vitreous humor of the eye, and hyaline cartilage. HA is involved in several important biological functions, such as regulation of cell adhesion and cell motility, manipulation of cell differentiation and proliferation, and providing biomechanical properties of tissues. HA interacts with several cell surface receptors such as CD44, RHAMM, and ICAM-1, and it controls cellular processes including morphogenesis, wound repair, inflammation, and metastasis. HA is responsible for maintenance of viscoelasticity of biofluids (synovial fluid and vitreous humor of the eye) and controlling tissue hydration and water transport [88].
d-glucuronic acid and dn-acetyl glucosamine, in the HA disaccharide structure are connected together through alternative beta-1, 4 and beta-1, 3 glycosidic bonds. The number of repeat disaccharides in a completed hyaluronan molecule can reach 10,000 or more, a molecular mass of ~4 million daltons (each disaccharide is ~400 Da). The average length of a disaccharide is ~1 nm. The structure of the disaccharide is energetically very stable in light of its beta configuration [88].
Key features of hyaluronic acid: HA is naturally synthesized by a class of integral membrane proteins called hyaluronan synthases, of which vertebrates have three types: HAS1, HAS2, and HA. The rheological property (concentration and molecular weight dependent) of HA solutions has made HA ideal for lubrication in biomedical applications. For example, a 1 % solution of high molecular weight HA (Mw ≳1,000 kDa) can behave like jelly, but when shear stress is applied it easily shears thin and can be administered via a thin needle. Viscoelasticity is another characteristic of HA, resulting from entanglement and self-association of HA random coils. This property, due to molecular interactions, depends in turn on concentration and molecular weight. Three types of enzymes like hyaluronidase (hyase), β-d-glucuronidase, and β-n-acetyl-hexosaminidase catalyze the enzymatic degradation of HA. Hyase cleaves high molecular weight HA into smaller oligosaccharides, while β-d-glucuronidase and β-n-acetyl hexosaminidase further degrade the oligosaccharide fragments by removing nonreducing terminal sugars. The degradation products of hyaluronan, oligosaccharides and very low molecular weight hyaluronan, exhibit pro-angiogenic properties. By catalyzing the hydrolysis of HA, a major constituent of the interstitial barrier, hyaluronidase lowers the viscosity of HA, thereby increasing tissue permeability. It is, therefore, used in medicine in conjunction with other drugs to speed dispersion and delivery [88]. Applications of HA are represented in Table 2.2.
Application | Role of Hyaluronic Acid |
---|---|
Dermal filler | Recover soft-tissue volume of the skin and remove skin wrinkles |
Chondroprotective effects | Effect of a HA injection may be mediated via CD44 interactions |
Potential agent of therapeutic intervention in osteoarthrosis (OA) | |
Orthopaedic applications | Development of cartilage, the maintenance of the synovial fluid and the regeneration of tendons |
Lubricant and shock absorber | |
Viscosupplementation (osteoarthritis treatment) | |
Antiadhesion applications | Prevent bacterial adhesion to dental implants, intraocular lenses, and catheters |
Ophthalmology | HA solutions also serve as a viscosity-enhancing component of eye drops and as an adjuvant to eye tissue repair |
Cardiovascular applications | HA increases the blood compatibilities of cardiovascular implants such as vascular grafts and stents. For example, biomaterial surfaces treated with cross-linked HA have been associated with reduced platelet adhesion and thrombus formation |
Chondroitin Sulphate
Chondroitin sulphate (CS), a GAG of the same class as glucosamine, is a disaccharide composed of alternate sequences of differently sulfated residues of d-glucuronic acid (GlcA) and n-acetyl-d-galactosamine (GalNAc) linked by β bonds. Sequences of these disaccharides are formed into polysaccharide chains. Some GlcA residues are epimerized into l-iduronic acid (IdoA); the resulting disaccharide is then referred to as dermatan sulfate. CS found extensively in the extracellular matrix of articular cartilage is obtained by extraction from tissues of several animals (bovine, porcine, avian, cartilagenous fishes, etc.). Glycosaminoglycans gather on the core protein and mass attach to HA via a link protein, which makes proteoglycans that are hydrated and resist compression stress. CS chains are linked to hydroxyl groups on serine residues of certain proteins [90].
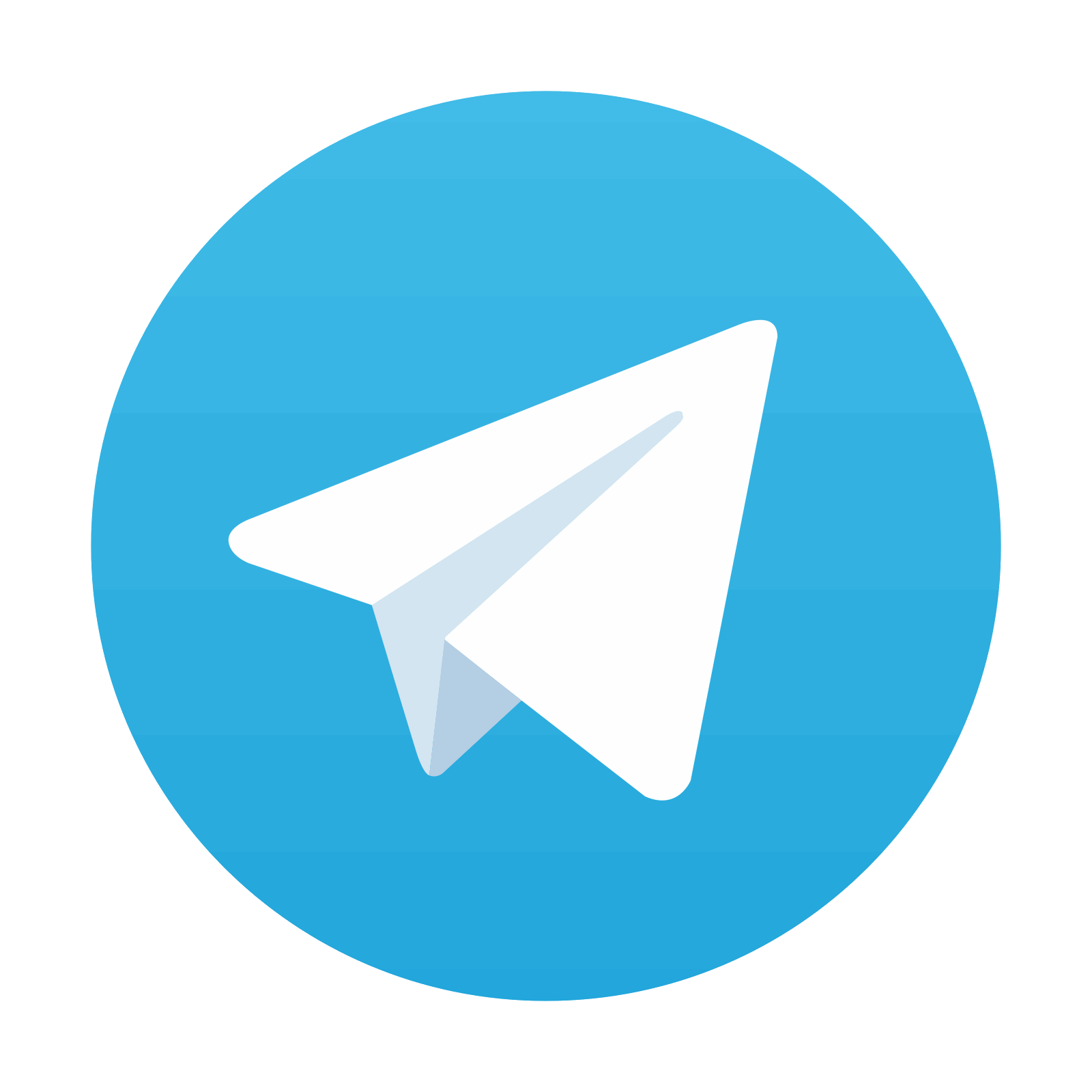
Stay updated, free articles. Join our Telegram channel

Full access? Get Clinical Tree
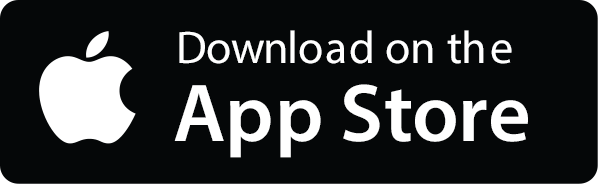
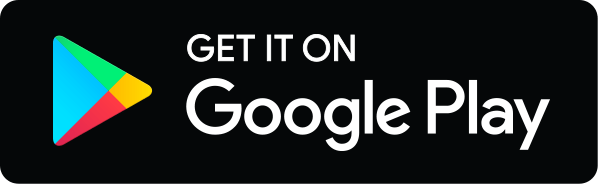