Bioburden Assessment and Tests for Sterility
Martell Winters
Trabue Bryans
Microbiology is central to all aspects of disinfection, sterilization, and preservation processes, primarily because the overall intent of these processes is to reduce, eliminate, or maintain the level of viable microorganisms. Bioburden estimation and sterility testing are likely the two most common microbiological tests performed in the validation of microbial reduction or prevention processes. These tests are useful tools in understanding the extent of microorganisms on or in a product, either before, during, or after bioburden reduction or prevention steps are applied; as such, the two tests are complimentary.
In standards relating to sterilization, bioburden is defined as the “population of viable microorganisms on or in product and/or sterile barrier system.”1 The bioburden can therefore consist of viable microorganisms found on a solid product, where an extraction process must be performed prior to plating, incubation, and enumeration. International Organization for Standardization (ISO) 11737-1 contains substantial information regarding bioburden testing of product where an extraction of microorganisms from product is required. Bioburden can also consist of viable microorganisms in a liquid sample or a sample that is soluble, where an extraction is not required prior to plating, incubation, and enumeration. Bioburden tests are addressed in ISO 11737-11 and pharmacopoeial documents such as United States Pharmacopeia (USP),2 European Pharmacopoeia (EP),3 Japanese Pharmacopoeia (JP),4 etc, in the chapters regarding microbiological examination of nonsterile products (microbial enumeration tests). The pharmacopoeial chapters are not called bioburden tests per se, but the intent and results are the same. The bioburden test is a quantitative test that results in microorganism counts, usually referred to as colony-forming units (CFU). This type of test is appropriate when there is expected to be some quantity of bioburden on or in product (ie, ≥1 CFU for the majority of product units tested).
In standards relating to sterility testing, specifically ISO 11737-2, “sterile” is defined as “free from viable microorganisms” and the primary focus of the standard is on validation of terminally sterilized products.5 Pharmacopoeial documents also address sterility testing with the primary focus being on validation and release of aseptically produced products.6 With these tests, the sterile state is difficult to prove with completeness. It is understood that there are limitations to what commonly used microbiological tests can demonstrate due to the inability of growth media to promote replication of every type of microorganism that could be present on or in a product. A sterility test is a qualitative or attribute test and is usually performed by immersing the product in, or adding the product to, a liquid growth medium followed by incubating and scoring as positive or negative for growth. This type of test is appropriate when there is expected to be no bioburden on or in product (ie, <1 CFU for the majority of units tested). Thus, the sterility test is often used to measure the presence/absence of a viable microorganism on a certain number of samples tested.
It is important to determine which test—the bioburden test or the sterility test—should be selected based on the situation. If a bioburden test is performed on product that is sterile, the result might be 0 CFU on the plated aliquot, but this result in a bioburden test is generally not as sensitive as a sterility test due to the extraction processes and/or dilutions that are often included. This means that a bioburden result of 0 CFU carries with it more uncertainty than a negative growth result in a sterility test. Alternatively, if a sterility test is performed on a group of products that likely are known to contain viable microorganisms, the expected result would be that many or all items tested were positive for growth, which usually provides no useful information as to the quantity of microorganisms per product. The focus of this chapter is to provide a review of these two tests, in some cases beyond what is generally known, or what is already contained in standards and guidance documents.

It is difficult to fully explain the need for knowledgeable microbiologists in the field of industrial microbiology. There are many microbiologists who have become testing experts, due to the many hours of time performing tests in their laboratory. This is a critical first step in developing true expertise; however, this kind of experience does not, in and of itself, develop true industrial expertise. Without exposure to other methods, alternate approaches, and regulatory challenges (external to the laboratory), true expertise cannot be gained. Indeed, the term expert is used too often by those who have become an expert at one aspect of microbiology in their industry.
True expertise is developed by the additional experience gained outside of the laboratory. Spending time in the manufacturing environment is necessary to help the microbiologist understand all that can transpire in the microbiological world. Microbiology tests performed in a laboratory often could be modified to be more appropriate for a specific product from a specific manufacturer once a better background of the manufacturing process is gathered. Involvement in industry associations, conventions and meetings also helps to develop expertise. There are few things as helpful to building expertise as working together on industry standards or guidance documents, sharing ideas and new technologies in a forum, or giving and observing presentations on the relevant topics.
It seems to be that many industries are losing needed microbiology expertise on the manufacturing side due to microbiology aspects becoming one of many routine assignments that is folded into quality, regulatory, or manufacturing. In some cases, this may be necessary due to the inability to maintain a full-time person devoted to microbiology, especially for smaller manufacturing firms. The disadvantage is that manufacturing (or industrial) microbiology as a discipline is consequently not addressed, unless an outside expert is involved in such cases. This often results in manufacturers relying on laboratory personnel for guidance on performing tests and interpreting data. Many laboratories are willing to provide this assistance, but it is often given based on what is commonly known or done rather than what is best for the specific product/process. Nonetheless, it is best if product manufacturers develop a good relationship with their laboratories, whether they are internal or external. Developing appropriate test methods and correctly interpreting bioburden and sterility test data must be a collaborative effort with the viewpoints of both the laboratory and manufacturing represented.

Disinfection, sterilization, and preservation processes do not always address all microbial issues that might be present in the production of acceptable product; some degree of control over the product components and manufacturing processes is required to allow the processes to yield consistent results. Bioburden testing is commonly performed on product obtained under standard manufacturing conditions in order to gather information (quantitative and qualitative) on the typical microbial load during or after manufacturing and packaging. Obtaining and trending these data help the manufacturer to demonstrate continued microbial control over an entire process; however, it is important to note that merely performing bioburden testing alone on a limited basis, for example, semiannually, does not constitute a robust bioburden control program.
Bioburden testing of finished product often results in reactive activities, meaning that the bioburden test data are used to determine if the process has been under some semblance of control over a specified time. The bioburden test on finished product is commonly implemented without any preliminary understanding of the contribution of microbial contamination from subprocesses or components during manufacturing. Use of bioburden testing in this manner is considered a single “end-point” test. If the data are acceptable, it is assumed that the entire process is under reasonable control. If the data are not acceptable, it could mean that any of the many upstream components and processes might have changed, or that certain processes—single or multiple—might be out of control. A thorough bioburden control program uses bioburden testing to understand and qualify higher risk components and steps of the overall process. These higher risk components and processes can usually be identified by a knowledgeable microbiologist who is familiar with the pertinent aspects of product manufacturing. The microbiologist establishes a testing scheme and gathers data either to demonstrate that the current practice is acceptable or to identify where attention is needed or corrections are appropriate.
An understanding of bioburden can be used in several situations such as the following:
Validation and maintenance of sterilization processes
Routine monitoring to demonstrate control of manufacturing processes
Monitoring of raw materials, components, or packaging
Assessment of the efficiency of cleaning or disinfection processes
Investigation into root cause for bioburden excursions
Development/maintenance of an overall environmental monitoring program
Bioburden on a finished product is the sum of the microbial contributions from several sources, including raw materials, manufacturing of components, assembly processes, personnel, manufacturing environment, assembly/manufacturing aids (eg, compressed gases, water, lubricants), cleaning processes, and packaging of finished product. To control product bioburden, attention must be
given to the microbiological status of these and other relevant sources. Strategic implementation of bioburden testing allows the microbiologist to correct practices or issues before they affect finished product, and to go into full-scale manufacturing with a degree of knowledge that it will be successful. When this approach is taken, the bioburden test of the finished product consequently becomes confirmation of the effort previously completed, rather than a hopeful exercise that will demonstrate control.
given to the microbiological status of these and other relevant sources. Strategic implementation of bioburden testing allows the microbiologist to correct practices or issues before they affect finished product, and to go into full-scale manufacturing with a degree of knowledge that it will be successful. When this approach is taken, the bioburden test of the finished product consequently becomes confirmation of the effort previously completed, rather than a hopeful exercise that will demonstrate control.
![]() FIGURE 64.1 Examples of a membrane filtration method (A), a membrane filter with colonies (B), and a spread plate with colonies (C). For a color version of this art, please consult the eBook. |
Bioburden testing is commonly performed on solid products (eg, plastics and metals) by performing an extraction with a water-based solution, followed by testing all or a portion of the extraction solution using membrane filtration, pour plating, or spread plating. For liquid products, bioburden testing is commonly performed by using membrane filtration, pour plating, or spread plating. When using the membrane filtration method, the membrane through which the liquid has been filtered is placed onto an agar plate or an absorbent pad saturated with liquid medium. After filtration and plating, or pour or spread plating, the agar plates are incubated at a specified temperature for a specified period to obtain visible colonies, followed by counting the colonies (Figure 64.1). Membrane filtration is often a preferred method because it allows for testing a larger volume of extraction solution or product. Pour or spread plating are good methods for bioburden enumeration but are limited by the quantity of solution or product that can be tested per plate. Bioburden testing is commonly performed in an environment to prevent contamination, usually a laminar flow hood in a controlled environment room, but usually not in a cleanroom because that level of control is not necessary.
It is sometimes assumed that bioburden testing will provide results of all types of microorganisms present on or in the product. This is not realistic because there are some types of microorganisms that require specific or unique growth nutrients, conditions, or incubation times to replicate and form a visible colony. For this reason, it is important that a microbiologist who is knowledgeable in the specific raw materials and manufacturing processes be involved in test development. Based on that information, growth media type(s) and incubation conditions can be selected that are best suited for the various microorganisms expected to be on the product. Most often, this assessment results in selection of media and incubation conditions that are ideal for the majority of bacteria (eg, those from surfaces, humans, and water) and environmental fungi (ie, molds and yeasts). Cultivation and detection of more unique bacteria and fungi, specific viruses, protozoa, etc, is often not practical nor indeed necessary in most situations. Specific applications, such as bioburden testing for allograft tissues and animal serum for human use, might need to consider the detection of certain material-related pathogens.7
Change is ever-present in most manufacturing environments. With multiple personnel involved in different aspects of product and processes, and each one of the personnel tasked to improve what is in their control, it is inevitable that change will happen. In many cases, change is for the better, but it must be controlled such that other aspects of the product or process are not inadvertently, negatively impacted. Bioburden tests, along with some degree of microorganism characterization (eg, morphology, selective culturing, Gram stain), are commonly employed as part of the change control process and are effective in understanding if the change has an unintended impact on the process or product.

The validation of a bioburden test, in its strictest interpretation, is not usually necessary if classical microbiological methods are being used. The terms qualification or verification usually apply, but if bioburden validation is to be considered, as with most microbiological tests, it should be assessed in two different manners. Validation of a bioburden test method is conducted as part of the
laboratory’s preparation to perform bioburden testing. This type of validation is not specific to a product type but can include several general product types to understand the variability that occurs in the parameters of the overall test method. At this stage, items such as the limits of detection (LOD) and limits of quantification as well as other components of validation such as ruggedness, precision, and accuracy can be addressed. This validation provides the laboratory with valuable information regarding the different parameters of the bioburden test method and the variability that can occur. In microbiology, it is not expected that the variability be as small as might be expected in more precise sciences such as chemistry or physical analysis.
laboratory’s preparation to perform bioburden testing. This type of validation is not specific to a product type but can include several general product types to understand the variability that occurs in the parameters of the overall test method. At this stage, items such as the limits of detection (LOD) and limits of quantification as well as other components of validation such as ruggedness, precision, and accuracy can be addressed. This validation provides the laboratory with valuable information regarding the different parameters of the bioburden test method and the variability that can occur. In microbiology, it is not expected that the variability be as small as might be expected in more precise sciences such as chemistry or physical analysis.
Once a bioburden test method has been validated, and when applying the test for a specific product, the product qualification process becomes the key factor, rather than the traditional components of a complete validation exercise. When addressing a bioburden test for a specific product, topics such as method suitability and recovery efficiency (see the following text) play a larger—and often exclusive—role compared to the other aspects of validation that are addressed in general validation of the test method. In the stage of bioburden qualification, it is usually also determined whether the entire product can be tested, which is called a sample item portion (SIP) of 1.0, or whether it is necessary to test a portion of the product (an SIP of <1.0). Most often, when testing a portion of the product becomes necessary, it is due to the large size of a product or to overcome inhibition of microbial growth in the test system. Whenever possible an SIP of 1.0 should be tested, and only when it is not practicable should an SIP <1.0 be considered. Some approaches (eg, when used in support of testing associated with radiation sterilization) require verification of the appropriateness of the SIP to ensure that the SIP is not too small to accurately represent the entire product, based on the purpose for the test.
Bioburden Method Suitability
Bioburden tests depend on the microorganisms’ ability to replicate in the growth conditions provided during incubation; hence, it is critical that the growth conditions allow replication to occur. If microorganisms are not able to replicate, a result of no colonies on an agar plate could be the result of inhibition in the test system rather than an indication of a low or negligible count of bioburden on the product. Until recently, this aspect of qualifying a bioburden test method for a specific product was not well addressed in ISO 11737-1.1 In contrast, it had been addressed for many years in the pharmacopoeial chapters on testing of nonsterile products.8 The demonstration of the absence of inhibitory substances in a bioburden test method can be addressed in different ways. The most common is to actively generate data to verify that microorganisms can replicate following the performance of the test method on a product. One common option is performing the proposed test method (eg, filtering aliquots of the extraction fluid being proposed to recover the microorganisms from the test device) and then inoculating the filter with known, low numbers of a selected microorganism (or microorganisms) followed by incubation and enumeration. The colony count on the filters through which the product extraction fluid was filtered is then compared to the count on control filters (in the absence of the product extraction fluid) of the same test microorganism suspensions (Table 64.1). Other variations of this approach can also be used, if there is a demonstration of a lack of microbiological inhibition in the test system.
TABLE 64.1 Example of bioburden method suitability data | |||||||||||||||||||||
---|---|---|---|---|---|---|---|---|---|---|---|---|---|---|---|---|---|---|---|---|---|
|
Alternatively, manufacturers who either have data on similar products or who more fully understand their processes can document that there are no inhibitory factors on their products or in associated test methods. This can be a simple exercise for synthetic materials, such as plastic and metal components, where the materials are previously qualified/understood as having no inhibitory factors and where all aspects of component manufacturing, cleaning, assembly, and packaging are generally understood and controlled. If documentation is provided in lieu of performing the test, the manufacturer must document that inhibitory substances are absent on product, such as leachable substances that might come from different polymer or component suppliers.
Bioburden recovery methods that include filtration of either an extraction fluid or filtration of the liquid product are effective methods to help eliminate or reduce inhibitory substances from the test system because the substances can potentially pass through the filter thereby being eliminated or reduced from the test system. Additionally, selection of the correct membrane filter or sufficient rinsing of the filter can minimize association or
binding of inhibitory substances to the filter material itself. If filtration and selection of specific membrane filters is not enough to eliminate or reduce inhibition in a test, options such as dilution and the addition of neutralizers into the extraction fluid, rinse fluid, or growth medium are commonly employed (see chapter 61).
binding of inhibitory substances to the filter material itself. If filtration and selection of specific membrane filters is not enough to eliminate or reduce inhibition in a test, options such as dilution and the addition of neutralizers into the extraction fluid, rinse fluid, or growth medium are commonly employed (see chapter 61).
Recovery Efficiency
The intent of the recovery efficiency test is to gather data regarding the ability of a bioburden extraction method to remove and culture microorganisms from a product. When the product can be directly tested, such as by filtration of a liquid product or a water-soluble product, the extraction of microorganisms from a surface is not required, so a recovery efficiency test would not be necessary. However, for solid products, the microorganisms need to be removed from the surface for an accurate estimation of the bioburden. This is not a new test because it has been performed for many years in the medical device and pharmaceutical industries. Typically, between 3 and 10 recovery efficiency values are determined for a product and then an average recovery efficiency is calculated, which is used to adjust bioburden data in future testing. But recent changes to the ISO standard merit some discussion on the topic.1
There are two commonly used options in performing the recovery efficiency test—the inoculated product technique and the repetitive recovery technique. The inoculated product option is more suitable for products that carry low numbers of naturally occurring bioburden (eg, <100 CFU), and involves inoculating the product with a known number of microorganisms (usually spores) followed by application of the extraction method, plating, incubation, and enumeration. The recovery efficiency is calculated by comparing the number of CFU known to have been inoculated onto the product to the number of CFU that were removed in the single extraction process. The repetitive recovery technique is more suitable for products that carry higher numbers of naturally occurring bioburden (eg, >100 CFU) and involves application of the extraction method multiple times on the same product (using the naturally occurring product bioburden), with each extraction process being followed by plating, incubation, and enumeration. The recovery efficiency is calculated by comparing the number of CFU that were removed in the first extraction process to the number of CFU that were removed in all of the extraction processes combined. Significant information and guidance on recovery efficiency is provided in the ISO standard,1 Annex C.
Previously, Annex A of ISO 11737-1 stated that for a recovery efficiency less than 50%, one should consider investigation whether the extraction method could be improved to obtain a value greater than 50%. This 50% value was arbitrarily selected, and it resulted in confusion in the industry that led many to believe that recovery efficiencies equal to or greater than 50% were a requirement. Because a requirement of this nature is not scientifically necessary, the 2018 version of the standard no longer contains the <50% recovery efficiency value. Instead, the new ISO 11737-1 standard states that the recovery efficiency value be viewed considering the purpose of the data and that a more important item regarding a set of recovery efficiency data is that the values are relatively consistent with each other and that they are appropriate for the intended use.
It is important in a bioburden test that the extraction method be capable of removing microorganisms in a consistent manner. This consistency is especially important when evaluating bioburden data over time or when the data are being used to establish sterilization processes or criteria for future reference. If this consistency is present, it is less critical whether the average recovery efficiency value is less than or greater than an arbitrary value. The new approach for recovery efficiency in the standards focuses on the use of the data in establishing an acceptable recovery efficiency. Annex C of ISO 11737-1 provides a good discussion of recovery efficiency and some examples of data.1
It could be argued that when an extraction is being performed on any product for a bioburden test that a recovery efficiency should be performed, but it should be noted that there are situations where it can be appropriate to not perform a recovery efficiency. For example, bioburden tests being performed on product components as part of routine monitoring of a supplier might not require a recovery efficiency to provide the necessary information, especially when the test is performed in the same manner over time. The intended purpose for the bioburden data is what should determine the need for and/or the use of a recovery efficiency.

Bioburden characterization is an underused practice in most industries. Where bioburden data are being gathered for health care products, it is often assumed that numerical data is the primary factor for evaluation rather than characterization data. Often, the need for, or the usefulness of, characterization is not fully understood, especially when microbiologists are not involved in the process. Characterization also requires an additional expense without—as it might appear—a direct, immediate correlation to outcome. Lastly, characterization data can be difficult for a nonmicrobiologist to interpret and trend, and therefore, the benefit can be difficult to demonstrate. Characterization should normally occur more frequently in the earlier stages of a manufacturing setting or process, to establish a baseline of the numbers and types of microorganisms present in the normal manufacturing scenario. Following that, characterization is an important key to demonstrating a controlled, stable process and product.
An understanding of the bioburden count alone is sometimes not enough to make an educated decision regarding interpreting microbiological data, assessing the potential impact of the bioburden to the product or process, or understanding the general microbiology of a product or process. Bioburden characterization is a broad term used to describe tests performed on the microorganisms after they have been recovered from the product or process. The most common methods of characterization are selection of specific growth conditions for groups of microorganisms, determination of colony morphology, Gram or other staining methods, and identification to genus and species.
The first step of characterization can include selection of specific growth conditions for groups of microorganisms. In some industries, it is common to employ use of selective or differential media to exhibit growth of specific microorganism types. Although this is considered characterization, it is sometimes too broad to be meaningful, depending on the purpose of the test. Certain types of media (Figure 64.2) or incubation temperatures can result in very specific outcomes. The important issue in using growth conditions as a means of characterization is to understand the desired end points and to ensure that the level of characterization provided meets those end points.
In the health care product industry, it is common to perform bioburden testing using different media and/or temperatures to encourage growth of both aerobic bacteria and fungi (molds and yeasts). This form of characterization helps the manufacturer gain a knowledge of the breakdown of microorganisms that are likely bacteria compared to molds. There is some usefulness in this knowledge, especially for trending bacteria and mold contamination over time. Less overlap and more differentiation will result when a different medium, specific for selection, is used for each microorganism type (eg, tryptic soy agar or nutrient agar for bacterial growth and potato dextrose agar or rose bengal agar for fungal growth). For bioburden testing in the health care industry, information regarding characterization to detect the presence of spores and anaerobes can also be considered. Testing for the presence of spores in product or environmental bioburden is sometimes performed in support of moist heat or ethylene oxide (EO) sterilization, where spores are considered more resistant to the process. Typically, spore testing is performed by applying high heat for a short period of time (eg, a heat shock at around 80°C for at least 10 min) to the bioburden extraction fluid to inactive vegetative bacteria, followed by plating and incubation.9 If testing for aerobic bacteria is also being performed on the same products, it must be understood that spores that grow after heat shocking will generally also grow without the heat shock in the aerobic bacteria test. Thus, although the spore selection test helps to distinguish the percentage of microorganisms that are spores, the spore test results should not automatically be summed with the aerobic bacteria test results, as the total number could likely be overestimated because the spores can be a subset of the aerobic bacteria.
![]() FIGURE 64.2 Different media types with microbial growth. For a color version of this art, please consult the eBook. |
Testing for the presence of anaerobes often results in a similar issue as with spores. Most health care products are composed of different types of synthetic materials, such as plastic and metal, where there is, first, no potential source for strict anaerobes (termed obligate anaerobes) and, second, little potential for survival of obligate anaerobes. With these products, an anaerobe test, if not properly conducted, will result in the growth of microorganisms that are facultative rather than obligate anaerobes, meaning that they can also grow in the aerobic bacteria test. Again, summing these two results, without a screening or differentiating step, could result in over estimation of the total bioburden as well as possibly the false assumption that the product contains obligate anaerobes. In the case of tissue or tissue-based products, there is a potential source for obligate anaerobes, therefore anaerobic testing could be warranted. Even in this case, the presence of facultative anaerobes is just as likely as that of strict anaerobes, so an evaluation is recommended prior to summing the results or assuming the presence of obligate anaerobes.
Another means of characterization involves documentation of the colony morphology, which includes visible aspects of the microorganism growth such as colony size, shape, and color (Figure 64.3). Colony morphology by itself is not usually sufficient for making decisions or trending, as there are both Gram-positive and Gram-negative microorganisms that are, for example, of similar size, shape, and pigmentation. Morphology comparisons are useful when looking for a specific microorganism, or for other general activities such as noting predominant colony types. Colony morphology is often accompanied by staining (most commonly a Gram stain) and microscopic inspection (cell morphology), which can prove to be a useful data point.
A microbiologist can use colony morphology and Gram stain information, along with an in-depth knowledge of the components and manufacturing processes, to assess potential source(s) of contamination, and, if needed, determine what kinds of mitigating activities can be employed. For example, Gram stains can provide information to assist a microbiologist in understanding whether the microorganisms comprising the product bioburden likely came from water, humans, or the environment.
A microbiologist can use colony morphology and Gram stain information, along with an in-depth knowledge of the components and manufacturing processes, to assess potential source(s) of contamination, and, if needed, determine what kinds of mitigating activities can be employed. For example, Gram stains can provide information to assist a microbiologist in understanding whether the microorganisms comprising the product bioburden likely came from water, humans, or the environment.
![]() FIGURE 64.3 Example of various colony morphologies on a membrane filter. For a color version of this art, please consult the eBook. |
Microbial identifications to the genus and species level are usually performed as part of investigations, for specific trending purposes, or to characterize predominant microorganisms for a general characterization program. There are many systems available for the specific identification of microorganisms, and it is not the intent of this chapter to go into any detail regarding these systems.10 These include various types of biochemical, lipid, and genetic identification techniques, where the genetic methods are currently generally considered necessary when establishing critical information that might be reviewed by regulatory bodies.
![]() FIGURE 64.4 Examples of gram-positive rods with spores (A), gram-negative rods (B), and gram-positive cocci (C). For a color version of this art, please consult the eBook. |
It should be mentioned that even though a quick search of a microbial genus and species might show that the microorganism in question can originate from a specific source, for example, animals or birds, it should not be assumed that such sources automatically relate to components or are present in the manufacturing area. Microorganisms have a way of populating multiple habitats, and of being spread from one place to another. Truly, they are a perfect example of how “life finds a way.” A microbiologist, armed with the genus and species information of a microorganism, is usually able to determine a likely source and, if needed, a potential means of prevention or elimination. For example, bacteria such as the Gram-positive cocci Staphylococcus epidermidis and Staphylococcus hominis are common bacteria found on human skin, whereas strains of Gram-positive rods such as Bacillus species are common dust or surface contaminants (Figure 64.4). Equally, many types of fungi (eg, species of Aspergillus and Penicillium) can be associated with airborne/dust contamination but may also indicate more serious problems such as water damage. Gram-negative rods, such as strains of Pseudomonas and Escherichia coli, are often associated with water contamination during the manufacturing process.11,12,13
Examples of Bioburden Characterization
The amount of characterization needed is dependent on the use of the data. The following are a few examples of how much characterization might be necessary in different situations. In a test where a specific microorganism is being investigated, such as an antimicrobial log reduction study, a simple comparison of the colony morphology to that of the control is often enough to accomplish characterization. In these cases, if the stock culture is pure, it is unlikely—in a laboratory with reasonable controls—that a contaminant could be introduced in sufficient numbers, with the same or similar colony morphology, to impact the results of this type of study. In these situations, it is
quite common to rely on the colony morphology as proof that the microorganism recovered is the microorganism being studied, rather than having to identify several colonies to genus and species to confirm the identity.
quite common to rely on the colony morphology as proof that the microorganism recovered is the microorganism being studied, rather than having to identify several colonies to genus and species to confirm the identity.
In an investigation into an out of specification result that is high, but not critically so, a Gram stain plus colony morphology can be acceptable to determine the likely source. For example, a high result where all colonies appear the same, and the Gram staining shows Gramnegative rods, it can suggest that the source is water contamination. It is common that water contamination causes high counts composed of a single or only a few microorganism types. Other types of contamination, such as from humans or the environment, will usually result in many types of microorganisms being present, which is usually evident by observing the various types of colonies on the plate.
In an investigation of a result that could be considered a failure of a process, such as a high bioburden count that would raise concerns regarding the acceptability of the product, it is more common to document colony morphology and perform an identification to genus and species. Although this might not provide significantly more information into the source compared to a Gram stain, it is more valuable from a comparison or trending perspective. The frequent appearance of the same genus and species on products or components, or having the same genus and species appear on both a sample and a control, for example, could demonstrate a trend, and be very valuable for investigations. This is a benefit compared to only performing a Gram stain, where there are many microorganisms that could exhibit the same Gram stain result.
It should be noted that the performance of bioburden characterization is only beneficial if a thorough baseline has been established. Characterization that is performed only when results are aberrant is usually of little benefit because there is nothing to which the results can be compared. It is important to be able to distinguish if a microorganism in an investigation is one that has routinely been present but is now present in higher numbers, or if a new type of microorganism has been introduced into the system. A solid baseline of microorganism data is usually established by more frequent monitoring—both of bioburden numbers and bioburden types—in the earlier stages of manufacturing or processing.
Characterization in Support of Sterilization and Aseptic Processing
Another aspect of characterization is in support of a sterilization process, or of aseptic processing of sterile products. All means of providing sterile product can benefit by incorporating some level of microbial characterization. Terminally sterilized products usually do not require as much characterization and overall understanding of the manufacturing process and product microbiology as does a process such as aseptic processing.
For terminal sterilization, modalities that most often employ an overkill process, such as EO, moist heat, dry heat, and vaporized hydrogen peroxide,14,15,16,17 generally need the least amount of initial bioburden characterization to demonstrate that the manufacturing process is in a state of control prior to conducting validation of the routine sterilization process. Typically, a more basic level of characterization is usually sufficient because these sterilization methods are typically established using worst-case situations and contain the highest level of additional safety for the sterilization process. In these instances, it is usually enough to establish a baseline bioburden characterization and then employ routine bioburden testing. Such testing could include a Gram stain, with occasional identifications to genus and species of predominant microorganisms, especially if the product bioburden is consistent and relatively low. Sometimes, testing for the presence of spores or identifying microorganisms to determine if they are spore-forming is recommended for overkill methods because spores are the types of microorganisms typically used to develop and validate the sterilization process. From a scientific standpoint however, if the product bioburden is moderate to low (eg, less than 1000 CFUs), even if 100% of the microorganisms are spore formers, there is almost no potential that this bioburden would impact the sterilization process based on the extreme high count of spores with a specific resistance that are used to challenge the sterilization process.
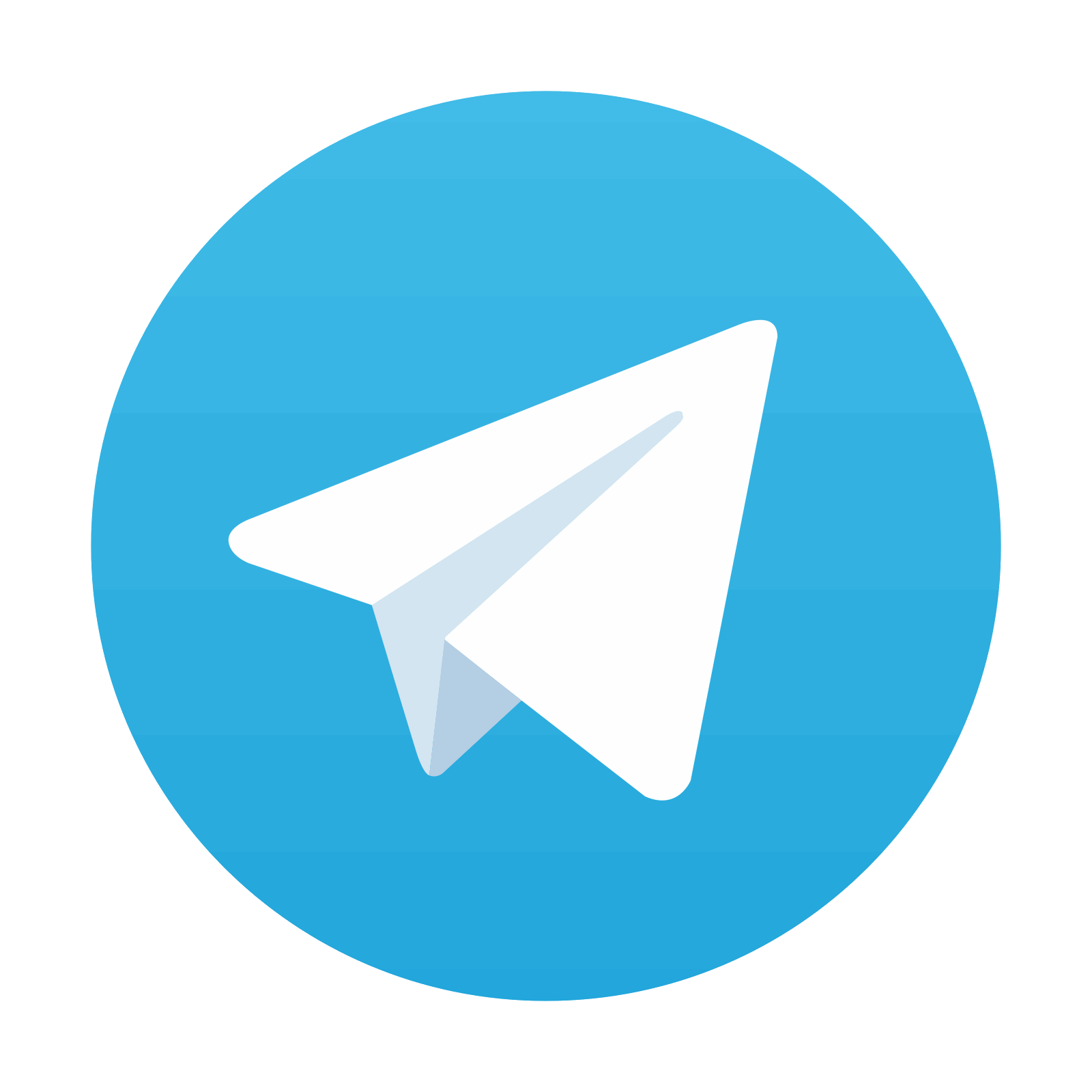
Stay updated, free articles. Join our Telegram channel

Full access? Get Clinical Tree
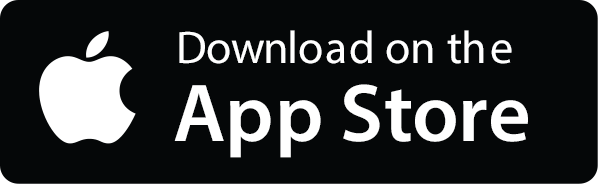
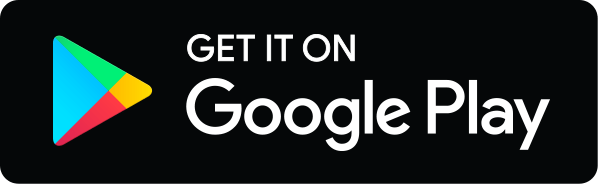