Basic Principles of Pharmacology
Overview
Invading organisms such as bacteria, viruses, fungi, and helminths can threaten the health of the host. Cancer cells are abnormal and differ from normal cells in terms of chromosome alterations, uncontrolled proliferation, dedifferentiation and loss of function, and invasiveness. Drug therapy (chemotherapy) aims to kill invading organisms or aberrant cells directly or to reduce their numbers to a level that can be managed by a host-mounted defense. Typical drug targets for invading organisms include biochemical processes needed for cell wall synthesis or integrity. Drug targets for abnormal cells include cell-cycle regulation and enzymes involved in protein synthesis, so as to inhibit cancer cell replication. In both cases, optimal treatment occurs when a drug or combination of drugs displays selectivity against invaders or cancer cells. Such therapy—with separation between a desired therapeutic effect and unwanted (adverse or side) effects—minimizes harmful drug effects.
When the amount of an endogenous substance is insufficient for normal functions, it may be possible to supply it from sources outside of the body (exogenous supply). Examples include insulin used for diabetes and dopamine used for parkinsonism. The exogenous material may originate from humans, animals, microorganisms, or minerals or it may be synthesized—a product of technology. It can be the substance itself or a precursor metabolized to the substance (eg, levodopa is metabolized to dopamine). Excess amounts can also be harmful, eg, excess stomach acid can cause or exacerbate ulcer formation. Gastric acid levels can be reduced directly by using an antacid (a base such as calcium carbonate or magnesium hydroxide). An alternative approach—–inhibiting acid secretion—can be achieved by antagonizing the action of histamine on H2 receptors of parietal cells (eg, with cimetidine) or by interfering with the proton pump that transports acid across parietal cells (eg, with omeprazole).
Drugs use different mechanisms to modify normal homeostatic and biochemical communication in cellular and physiologic processes. They mimic (eg, carbachol) or block neurotransmitters that transmit information across synapses. Chemical substances such as hormones also act over long distances in the body. Drugs that mimic hormones include oxandrolone; mifepristone blocks hormone action. Drugs selectively modify physiologic processes by targeting enzymes, DNA, neurotransmitters, or other chemical mediators or components of signaling processes such as receptors. The total effect depends on whether a drug promotes or reduces endogenous activity. Drugs with other mechanisms of action are chelating agents (contain metal atoms that form chemical bonds with toxins or drugs), antimetabolites (masquerade as endogenous substances but are inactive or less active than these substrates), irritants (stimulate physiologic processes), and nutritional or replacement agents (eg, vitamins, minerals).
Communication (transmission of information) across synapses occurs via chemical messengers—neurotransmitters—stored in vesicles in presynaptic neurons. Action potentials at presynaptic axon terminals initiate steps that release neurotransmitter molecules into a synapse, which cross the synaptic cleft and bind reversibly to postsynaptic receptors. Receptor activation leads to cellular response. Receptor activators (eg, drugs) are agonists; antagonists are drugs that combine with but do not activate receptors. Transmitters are removed from synapses by enzymatic destruction, diffusion, and active reuptake into presynaptic neurons. Major peripheral neurotransmitters are acetylcholine and catecholamines (eg, epinephrine, dopamine). In the brain and spinal cord, major excitatory neurotransmitters are glutamate and aspartate; major inhibitory neurotransmitters are GABA and glycine. 5-HT, or serotonin, and neuropeptides are other neurotransmitters.
A synapse is a region including the axon terminal of a presynaptic neuron, the plasma membrane of the postsynaptic (receiving) cell, and the physical space between the cells (synaptic cleft). Postsynaptic cells can be neurons or other cells (eg, effector cells in muscle). At synapses, electrical transmissions—action potentials along presynaptic neurons—are translated into chemical signals, which lead to postsynaptic cell responses: increase (excitation), decrease (inhibition), or modulation of neuron activity or biochemistry. Synaptic transmission involves many steps, all possible drug targets. Steps occur in presynaptic neurons (eg, neurotransmitter synthesis and storage in vesicles), at presynaptic membranes (eg, vesicle docking with membranes, neurotransmitter exocytosis), in synaptic clefts (eg, enzymatic reuptake), on postsynaptic membranes (eg, binding to receptors, change in ion channel function), and in postsynaptic neurons (eg, effects on second-messenger transduction).
Receptors are the first molecules in or on a cell that respond to a neurotransmitter, a hormone, or another endogenous or exogenous signaling molecule (ligand) and transmit messages (via transduction) from the molecule to the cell machinery. Receptors ensure fidelity of the intended communication by responding only to the intended signaling molecule or to molecules with closely related chemical structures (such as drugs with the required shape). Receptors are composed primarily of long sequences (typically hundreds) of amino acids. The body has dozens of receptor types to maintain communication pathways that must be differentiated from each other and serve different purposes. An individual cell may express one or many types of receptors, with the number depending on age, health, or other factors.
Receptors can be classified into subtypes, as first noted for receptors for the structurally related catecholamines epinephrine, isoproterenol, and norepinephrine. The order of potency (structure-activity relation, or SAR) of these drugs in some tissues is norepinephrine > epinephrine > isoproterenol; in other tissues, it is the reverse. Catecholamine receptors (adrenoceptors) exist in pharmacologically distinct types (α and β) and subtypes (eg, α1, α2, and so on). Subtypes are differentiated by amino acid sequence and posttranslational processing, as shown for dopamine receptor subtypes. A clinical example of receptor subtype targeting involves asthma treatment. Activation of adrenoceptors in the lung relaxes smooth muscles and dilates bronchioles to ease breathing. To avoid stimulation of heart adrenoceptors, β2-selective drugs (eg, albuterol, metaproterenol, ritodrine, terbutaline) were developed to activate only lung adrenoceptors; β1-selective drugs would affect the heart.
Certain molecules have physiochemical and stereochemical (3-dimensional) characteristics that impart affinity for a receptor, affinity being the quantifiable tendency of a drug molecule to form a complex with (bind to) a receptor. Binding involves interaction between a ligand molecule (L) and a receptor molecule (R) to form a ligand-receptor complex (LR): L + R ↔ LR. Affinity is quantified by the reciprocal of the equilibrium constant of this interaction and is commonly reported (often designated Kd or Ki); the greater the affinity is, the smaller the K value is. Drugs can activate receptors and thus elicit a biologic effect (ie, have intrinsic activity, or efficacy). Such molecules have shapes complementary to receptor shapes and somehow alter the activity of a receptor. Full agonists possess high efficacy and can elicit a maximal tissue response, whereas partial agonists have intermediate levels of efficacy (the tissue response is submaximal even when all receptors are occupied).
Some molecules have physiochemical and stereochemical traits that impart affinity for a receptor but cannot activate it. Such molecules bind to (occupy) receptors and block access of agonists, thereby reducing the effects of agonists. Such pharmacologic antagonists do not elicit biologic effects directly; they modify the physiologic process that is maintained by agonist action (eg, by neurotransmitters). Examples of drugs that are receptor antagonists are atropine (muscarinic cholinergic), d-tubocurarine (nicotinic cholinergic), atenolol (adrenoceptor), spironolactone (mineralocorticoid), diphenhydramine (histamine H1), ondansetron (5-HT), flumazenil (benzodiazepine), haloperidol (dopamine), and naloxone (opioid). Chemical antagonism (eg, neutralization of gastric acid by chemical bases) or physiologic antagonism, in which an effect of one drug opposes an effect of another agent (eg, epinephrine used to counteract the histamine response to a bee sting), of drug effects can also occur.
One enantiomer of a racemic pair is often observed to bind more avidly to (has greater affinity for) a receptor than does the other enantiomer of the pair. Because the only difference between them is the stereochemistry, the 3-dimensional shape of a molecule must be a crucial characteristic for binding affinity. The relation between chemical structure and biologic response is known as the SAR and is a common focus of drug discovery efforts. Computer modeling of the ligand-receptor fit provides a visual representation of the fit of a ligand into the receptor pocket. It can also be used for virtual screening for goodness of fit of potential drug candidates before they are synthesized.Stay updated, free articles. Join our Telegram channel

Full access? Get Clinical Tree
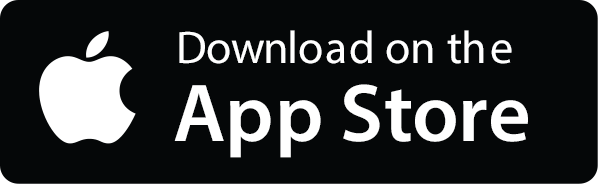
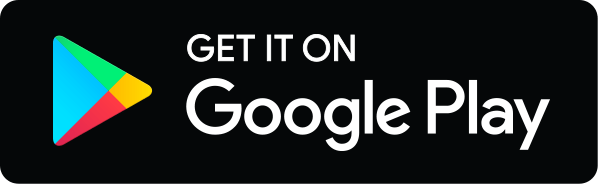